Exploring the World of Single-Celled Organisms
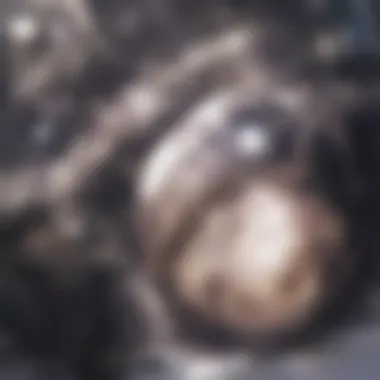
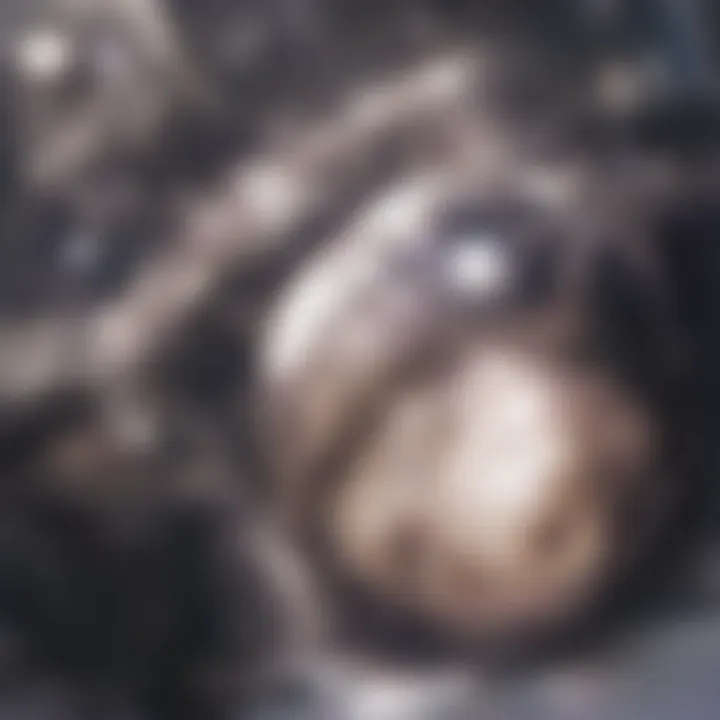
Intro
Single-celled organisms, often referred to as microbes, form a vast and essential component of life on Earth. They inhabit every conceivable environment, from the depths of the oceans to internal human systems. This section opens an exploration of their complexity and role in our biosphere.
Understanding single-celled organisms involves recognizing their diversity, from bacteria to protozoa, and their unique structural features. These organisms, often microscopic, operate in intricate ecosystems and have significant ecological impacts. They play crucial roles in nutrient cycling and energy flow, influencing larger biological systems.
Furthermore, recent advances in research shed light on their genetic makeup, reproductive strategies, and even their use in various biotechnological applications. The next sections delve deeper into these findings, emphasizing how they enhance our grasp of fundamental biological processes.
Prologue to Single-Celled Organisms
Single-celled organisms are often overlooked but are fundamental to life on Earth. Their structures and functions reveal vital information about biological processes. Understanding these organisms provides insights into ecosystems, disease mechanisms, and biotechnological applications. Their significance spans diverse fields like medicine, environmental science, and genetics, making them a critical topic of study.
Definition and Classification
Single-celled organisms, or unicellular organisms, are defined as living entities that consist of only a single cell. This cell can perform all necessary functions for life. Common classifications include bacteria, archaea, protozoa, and algae.
- Bacteria: These are prokaryotic organisms that lack a nucleus. They are widespread and can be found in various environments. Bacteria can have different shapes such as rods, spheres, and spirals.
- Archaea: Similar to bacteria but with distinct biochemistry and genetics. They often inhabit extreme environments and are known for their ability to survive in harsh conditions.
- Protozoa: These are mostly motile eukaryotic organisms. They often feed on organic matter and other microorganisms.
- Algae: Simple photosynthetic organisms that can be unicellular or multicellular. They can be found in various aquatic environments.
These classifications help in understanding the vast diversity of life forms and their evolutionary relationships.
Historical Perspective
The exploration of single-celled organisms dates back to the 17th century. Anton van Leeuwenhoek, a Dutch scientist, was among the first to observe these microbes using a microscope. His findings laid the groundwork for microbiology.
Over time, the development of various classification systems evolved. Carl Woese introduced the three-domain system, which divided life into Archaea, Bacteria, and Eukarya. This model highlighted the fundamental differences between these groups and improved our understanding of evolutionary biology.
Understanding the historical context of single-celled organisms enriches our knowledge about their evolution and relevance to modern science. This timeline showcases humanity's journey in uncovering the hidden intricacies of these life forms.
Types of Single-Celled Organisms
Understanding the types of single-celled organisms is essential for grasping their roles in ecosystems and their interactions with other life forms. This knowledge supports advancements in fields like biotechnology, medicine, and environmental science. The classifications below emphasize the diversity within single-celled life and highlight their unique characteristics.
Bacteria
Bacteria are among the most abundant and versatile life forms on Earth. They exist in nearly every environment, from deep oceanic vents to human intestines. Their cell structure is prokaryotic, meaning they lack a membrane-bound nucleus.
Bacteria can be classified based on shape, such as cocci (spherical), bacilli (rod-shaped), and spirilla (spiral-shaped). These shapes impact their functions in various contexts. Important processes include:
- Decomposition: Bacteria play a critical role in breaking down organic matter, recycling nutrients back into the ecosystem.
- Nitrogen Fixation: Some bacteria convert atmospheric nitrogen into forms usable by plants, essential for agricultural productivity.
- Pathogenicity: While many bacteria are beneficial, some can cause diseases in humans, plants, and animals, making the study of pathogenic bacteria necessary for public health.
Archaea
Archaea are a distinct group of single-celled organisms, similar in appearance to bacteria but genetically and biochemically different. They often thrive in extreme environments, such as hot springs and salt lakes, which sets them apart from most other life forms. Archaea can be classified into various groups based on their metabolic pathways, including:
- Methanogens: These archaea produce methane and are crucial in anaerobic environments, such as wetlands and the digestive tracts of ruminants.
- Halophiles: These organisms thrive in high-salt conditions, such as salt flats and salt mines.
- Thermophiles: Found in extreme heat environments, these archaea can survive in temperatures above 70 degrees Celsius.
Their existence expands the understanding of life's adaptability and contributes to biotechnological innovations.
Protozoa
Protozoa are eukaryotic microorganisms that are generally motile and require moisture for survival. They are primarily classified based on their locomotion. Protozoa provide insights into the evolution of multicellular organisms. Notable groups include:
- Amoeboids: Characterized by their ability to change shape and move via pseudopodia. They play significant roles in soil ecosystems.
- Flagellates: These protozoa are equipped with flagella for movement. Some are symbiotic while others can be pathogenic, as seen with Giardia lamblia.
- Ciliates: Covered in cilia for movement and feeding. A well-known ciliate is Paramecium, often studied in biology classes.
Protozoa interact with each other and their environment, affecting nutrient cycling and food webs.
Algae
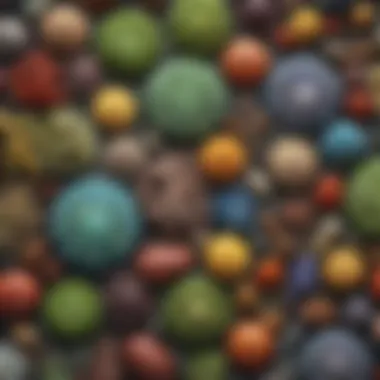
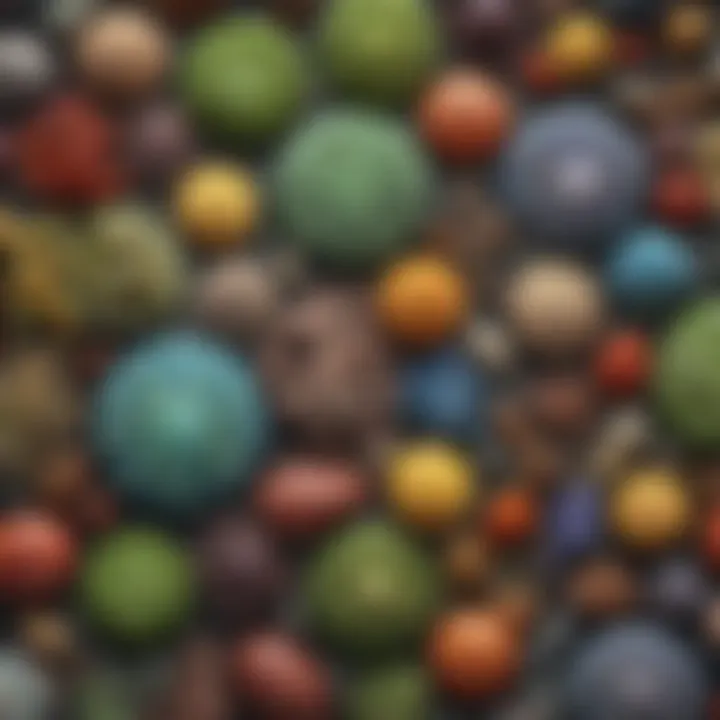
Algae are primarily photosynthetic eukaryotic organisms that can be unicellular or multicellular. They occupy a range of aquatic environments, contributing significantly to global primary productivity. Algae can be categorized into several groups:
- Chlorophyta (Green Algae): Contentious in freshwater, they contribute to oxygen production and form the base of many aquatic food chains.
- Rhodophyta (Red Algae): Found mostly in marine environments, they are critical for coral reef formation and other marine ecosystems.
- Phaeophyta (Brown Algae): These include larger forms such as kelp, which provide habitat and food for many marine creatures.
Algae are also studied for their applications in biofuel production and carbon capture strategies.
"The diversity of single-celled organisms reflects the complexity of life itself and underscores the significance of these microorganisms in various biogeochemical processes."
Understanding the types of single-celled organisms enhances knowledge of their ecological roles, their applications in various fields, and their contributions toward a sustainable future.
Cell Structure and Function
Understanding cell structure and function is essential when studying single-celled organisms. These minute entities serve as the foundation of more complex life forms. Each component within a single-celled organism plays a distinct role. This knowledge is crucial, as it allows researchers to better comprehend biological processes and how life functions at the most fundamental level. The intricacies of these microscopic entities reveal much about life science's evolution and application.
Cell Membrane and Transport Mechanisms
The cell membrane is a critical structure that defines the boundary of the cell. It regulates what enters and exits the cell, maintaining homeostasis. This semi-permeable membrane consists of a phospholipid bilayer with embedded proteins. These proteins facilitate transport mechanisms.
There are several transport types that cells use:
- Passive Transport: This occurs when substances move across the membrane without energy expenditure. This process includes diffusion and osmosis.
- Active Transport: Here, cells require energy, usually from ATP. This process is critical for moving substances against their concentration gradient.
The efficiency of these mechanisms impacts how single-celled organisms adapt to their environments and acquire essential nutrients.
Genetic Material and Protein Synthesis
Inside single-celled organisms, genetic material is often located in a region known as the nucleoid. It consists primarily of DNA which holds the instructions vital for cellular functions. The ability to replicate DNA is essential for the organism's reproduction and survival.
Protein synthesis is a fundamental process that enables cells to produce proteins necessary for metabolism, structure, and response to stimuli. This occurs in two main stages:
- Transcription: The DNA is transcribed to messenger RNA (mRNA) in the nucleus or nucleoid.
- Translation: The mRNA is translated into proteins at the ribosomes.
These processes underscore the importance of genetic material as it directly influences how single-celled organisms function and evolve.
Metabolism and Energy Production
Metabolism refers to the sum of all chemical reactions within a cell, enabling it to grow, reproduce, and respond to stimuli. Single-celled organisms possess various metabolic pathways. These pathways allow them to convert different substrates into energy.
Main metabolic types include:
- Aerobic Metabolism: This process requires oxygen to generate energy. Organisms like Escherichia coli can utilize this method when oxygen is available.
- Anaerobic Metabolism: Oxygen is not required in this process. Some organisms can thrive in entirely oxygen-deprived environments, employing fermentation.
The versatility of metabolic processes in these organisms highlights their ecological adaptability. They fulfill crucial roles in nutrient cycling and contribute significantly to their environments.
Understanding the structures and functions of single-celled organisms expands our knowledge of lifeβs complexity and adaptability in diverse conditions.
Reproduction and Lifecycle
The study of reproduction and lifecycle in single-celled organisms offers a profound window into their adaptability and survival, critical elements in understanding these minimalist life forms. Single-celled organisms employ diverse reproductive strategies that allow them to thrive in various ecological niches. This examination is essential because it sheds light on their population dynamics, ecological roles, and evolutionary potential. By understanding the nuances of these processes, one can appreciate how these organisms contribute to broader biological systems and human applications.
Asexual Reproduction
Asexual reproduction is the primary mode of replication for many single-celled organisms. This process is defined as the generation of new individuals from a single parent without the involvement of gametes. One common method of asexual reproduction is binary fission. During binary fission, the organismβs DNA replicates, then the cell splits into two identical daughter cells. This method is efficient and allows rapid population growth under optimal conditions.
Other methods of asexual reproduction include budding and fragmentation. In budding, an offspring grows directly from a parent organism. Fragmentation involves breaking into pieces, each of which can develop into a new organism. These processes are indicative of the versatility of single-celled organisms in adapting to various environments. However, asexual reproduction does not allow for genetic diversity, which can be a limitation when facing environmental challenges.
Sexual Reproduction
Sexual reproduction, although less common among single-celled organisms, is a significant process that introduces genetic variation into populations. This method generally involves the fusion of specialized cells, known as gametes, from two parent organisms. In many protozoa, for example, sexual reproduction occurs through a process called conjugation. Here, two organisms exchange genetic materials, contributing to genetic diversity in the population.
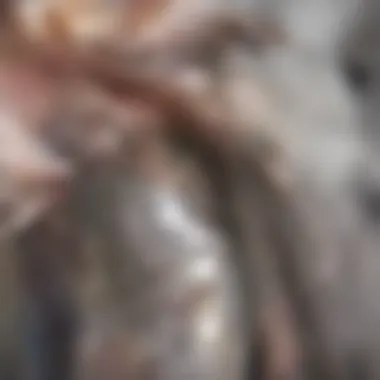
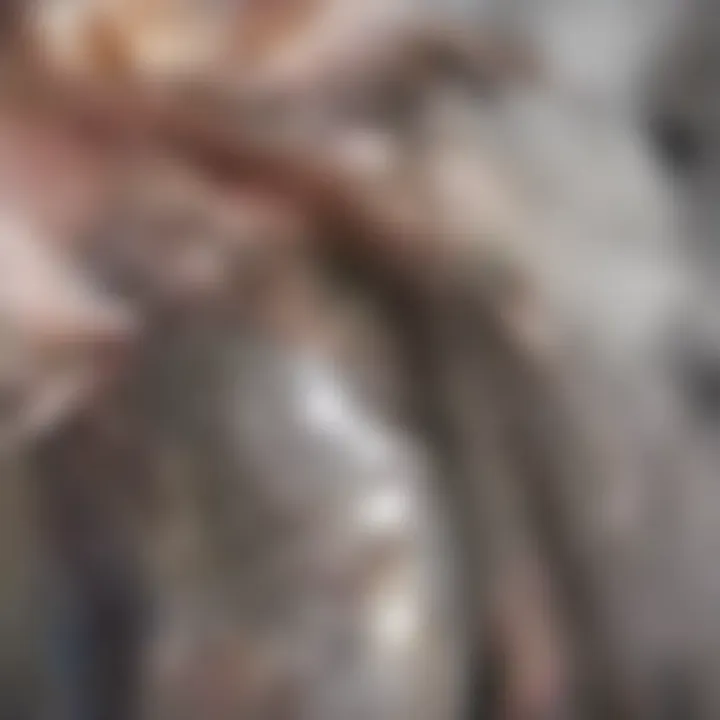
The importance of sexual reproduction cannot be overstated. It allows for greater adaptability by creating new combinations of genes. This genetic mixing can increase resilience against environmental stressors and diseases. Furthermore, the evolution of sexual reproduction can be viewed as a significant milestone in the history of life, leading to the complexity of multicellular organisms.
Lifecycle Variations
Single-celled organisms display a range of lifecycle variations, enabling them to adapt to their environments. Some organisms have simple life cycles, while others have more complex stages that may include both asexual and sexual phases. For example, the social amoeba Dictyostelium discoideum begins its life as a solitary organism but can aggregate to form a multicellular structure when food is scarce.
Some species can undergo encystment, forming a protective cyst when conditions are unfavorable. This capability allows survival during adverse environmental changes, such as drought or extreme temperatures. The ability to switch between different lifecycle stages underscores the adaptability and resilience of single-celled organisms, making them an integral part of ecosystems.
The capacity for asexual and sexual reproduction in single-celled organisms underlines their evolutionary success and ecological significance.
In summary, reproduction and lifecycle strategies in single-celled organisms are fundamental aspects that underline their survival and adaptability. Understanding these processes illuminates their critical role in ecosystems and their potential applications in biotechnology and medicine.
Ecological Roles
Ecological roles of single-celled organisms are fundamental to understanding their significance within ecosystems. These tiny life forms participate in various processes that sustain life on Earth. Their involvement in nutrient cycling, symbiotic relationships, and even pathogenic interactions highlights their diverse nature.
Decomposers and Nutrient Cycling
Single-celled organisms play a crucial role as decomposers in ecosystems. They break down organic matter, which is essential for nutrient cycling. When plants and animals die, their remains become a source of energy for these organisms. For example, bacteria and fungi decompose dead matter into simpler substances. This process releases nutrients back into the soil, promoting plant growth.
Benefits of decomposition include:
- Recycling essential nutrients
- Enriching soil fertility
- Supporting primary productivity
Without these decomposers, ecosystems would be overwhelmed by dead organic material, disrupting the balance of the environment.
Symbiotic Relationships
Symbiosis refers to the interaction between different organisms, which can be mutualistic, commensalistic, or parasitic. Single-celled organisms are often involved in these interactions, influencing ecological balance. One well-known example is the relationship between Rhizobium bacteria and legumes. In this mutualistic relationship, bacteria fix nitrogen from the atmosphere, enriching the soil while legumes provide carbohydrates to the bacteria.
Types of symbiotic relationships include:
- Mutualism: Both organisms benefit, as seen in the case of corals and zooxanthellae (a type of algae).
- Commensalism: One organism benefits and the other remains unaffected.
- Parasitism: One organism benefits at the expense of the other; for example, Plasmodium species that cause malaria in humans.
Understanding these relationships is vital for predicting ecological changes and managing natural resources.
Pathogenic Single-Celled Organisms
While many single-celled organisms have beneficial roles, some are pathogenic and can cause diseases in humans, animals, and plants. Pathogenic single-celled organisms such as bacteria and protozoa contribute to many health problems worldwide. Notably, bacteria like Escherichia coli, in specific strains, can lead to severe foodborne illnesses.
Examples of pathogenic single-celled organisms include:
- Giardia lamblia, responsible for giardiasis, a gastrointestinal infection.
- Entamoeba histolytica, which causes amoebic dysentery.
Addressing the challenges posed by these pathogens requires ongoing research into disease prevention and treatment. Awareness of their ecological roles can lead to better environmental and public health strategies.
"Single-celled organisms not only contribute to the stability of ecosystems but also remind us of our interconnectedness with all life forms."
In summary, the ecological roles of single-celled organisms are diverse and complex, influencing nutrient cycling, fostering mutually beneficial relationships, and posing health risks. Their importance in the biosphere cannot be overstated.
Evolutionary Context
The evolutionary context of single-celled organisms is crucial for understanding their significance in the larger tapestry of life. These organisms are often regarded as the fundamental building blocks of all life forms. They illustrate the processes of evolution, adaptation, and speciation that have occurred over billions of years. As prokaryotic organisms, such as bacteria and archaea, they represent some of the earliest life forms on Earth. Their study can reveal insights into the origins of life and the mechanisms driving evolution in more complex organisms. Through this context, we can appreciate how single-celled organisms contribute to biodiversity and ecosystem dynamics.
Origin of Single-Celled Life
The origin of single-celled life is a topic of great interest in biological sciences. It is widely believed that life began in the form of simple cells more than 3.5 billion years ago. The first single-celled organisms, likely similar to today's prokaryotes, emerged in a world vastly different from the one we know today. Conditions on early Earth, including heat from volcanic activity and solar radiation, created an environment ripe for the formation of complex molecules like amino acids and nucleotides.
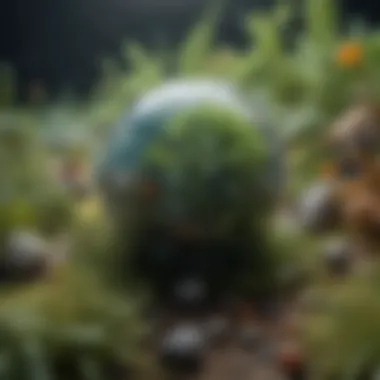
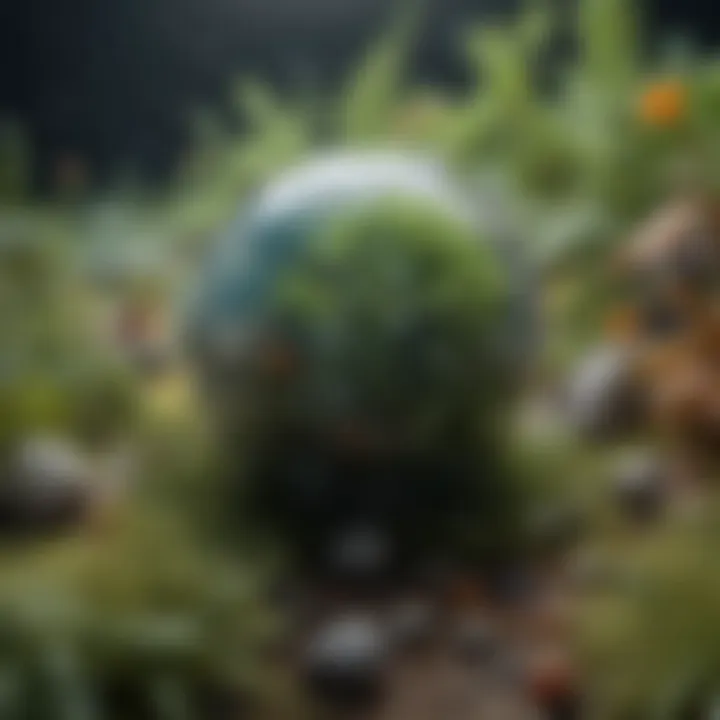
This process, known as abiogenesis, led to the formation of the first living entities. RNA molecules may have played a pivotal role in this early life, possibly serving as the original genetic material before DNA took over. The emergence of single-celled life forms marks a significant step in the evolutionary timeline, paving the way for more complex multicellular organisms. Understanding these origins helps frame the evolution of life itself, illustrating the adaptability and versatility of these simple entities.
Evolutionary Innovations
Throughout their long evolutionary history, single-celled organisms have undergone numerous innovations that have improved their survival. One of the most significant evolutionary innovations is metabolic diversity. For instance, some bacteria can perform photosynthesis, while others have adapted to survive in extreme environments, like hydrothermal vents. These capabilities reveal the ability of life to exploit various ecological niches.
Another innovation is the development of complex cellular structures, such as organelles within eukaryotic cells. The endosymbiotic theory posits that certain organelles, like mitochondria and chloroplasts, originated from free-living prokaryotes that entered into a symbiotic relationship with ancestral eukaryotic cells. This theory not only explains the evolution of cellular complexity but also highlights the cooperative nature of life.
"Single-celled organisms represent a vast array of evolutionary strategies, showcasing life's ability to adapt and thrive under varying circumstances."
In summary, the evolutionary context of single-celled organisms provides valuable insights into the origins and innovations of life on Earth. The exploration of these aspects enhances our understanding of biological processes, adaptability, and the intricate connections within the biosphere.
Biotechnology and Application
Biotechnology that involves single-celled organisms represents a vital area in modern science. The manipulation and application of these organisms lead to significant advancements in various fields. The use of single-celled life forms facilitates processes that range from industrial to medical applications. Understanding the role of these organisms in biotechnology provides insights into both their potential and challenges.
Role in Bioengineering
Single-celled organisms are essential in bioengineering for several reasons. Notably, bacteria, yeast, and certain algae can be genetically modified to produce valuable products.
- Protein Production: Bacteria like Escherichia coli are extensively used to synthesize proteins. This includes insulin, which is crucial for diabetes treatment. The efficiency of these organisms allows for large-scale production.
- Biodegradation: Certain microorganisms can break down pollutants, contributing to bioremediation efforts in contaminated environments. This highlights their potential in sustainable practices and environmental recovery.
- Bioreactors: Bioengineering relies on bioreactors where single-celled organisms are cultured under controlled conditions. This enhances yields of desired products while minimizing waste.
In these contexts, single-celled organisms are not only tools but also agents of change that can remedy ecological disturbances and improve human health.
Medical Applications
In medicine, the applications of single-celled organisms are profound. The versatility of these organisms paves the way for novel solutions to complex medical challenges, including:
- Vaccine Development: Living organisms like yeast are used to create vaccines. This method allows for the production of safer, more effective immunizations.
- Therapeutic Agents: Certain bacteria are engineered to deliver therapeutic agents directly to diseased tissues. This targeted approach reduces side effects compared to traditional methods. The development of such therapies is transforming cancer treatment and other diseases.
- Diagnostic Tools: Single-celled organisms play a role in diagnostic equipment. They enhance the detection of pathogens and diseases, facilitating faster and more accurate diagnoses.
The relevance of these applications becomes clearer when considering the impact on public health. The advancements resulting from utilizing single-celled organisms in biotechnology will shape the future of medicine. As research continues, the possibilities for addressing current medical hurdles expand.
"The exploration of single-celled organisms in biotechnology demonstrates a unique blend of biology and innovation, unlocking solutions that were previously unattainable."
Future Perspectives
The exploration of single-celled organisms is not merely a scientific ambition; it is pivotal for comprehending broader biological concepts that govern life on Earth. This section highlights key aspects regarding future research and the significance these organisms hold for humanity as we evolve in our understanding of biology and technology.
Research Directions
- Genomic Advancements: As methods for genome sequencing continue to improve, there is a potential for groundbreaking discoveries in single-celled life. By studying the genomes of various single-celled organisms, researchers can uncover genetic adaptations that contribute to their survival in extreme environments.
- Synthetic Biology: Using single-celled organisms as chassis in synthetic biology presents an opportunity to engineer microbes for specific purposes. This could include producing biofuels or pharmaceuticals. The fundamental understanding of cellular mechanisms will drive innovations in bioengineering fields.
- Ecological Impact Studies: Investigating the roles of single-celled organisms in ecosystems will alert us to the shifts that impact biodiversity and climate change. Given their pivotal position in food webs and nutrient cycling, it is essential to monitor their populations in various environments.
- Bioremediation Techniques: Researching the capabilities of single-celled organisms in breaking down pollutants opens doors for sustainable environmental solutions. Specifically, certain bacteria and algae are already being explored for their potential in cleaning contaminated sites.
In essence, this ongoing research holds vast potential. Insights gathered from single-celled organisms can directly inform strategies for various global challenges, such as sustainability, health, and environmental balance.
Implications for Humanity
Understanding single-celled organisms is integral to advances in multiple sectors:
- Healthcare Innovations: The study of pathogenic single-celled organisms has profound implications for public health. Knowledge gained can lead to the development of new therapeutic strategies against diseases caused by these microorganisms, such as malaria and tuberculosis.
- Food Production: Microbes play significant roles in food processing. Insights on algae could enhance food security through sustainable practices in aquaculture and vegetable oil production.
- Agricultural Development: Beneficial single-celled organisms enhance soil health and crop yields. Research into these can improve agricultural practices, which is crucial given the global population increase.
According to experts, the ongoing research into single-celled life can empower solutions to some of the most pressing issues facing humanity today.
"Unlocking the secrets of single-celled organisms can be the key to addressing global challenges in health, ecology, and sustainability."
Culmination
The conclusion serves as a vital aspect of this article, synthesizing the various intricate elements that characterize single-celled organisms. It highlights their complexity and versatility in an ecosystem, revealing the foundational roles they play across multiple domains. A comprehensive understanding of these organisms is crucial not only for biological science but also for diverse fields, such as medicine and environmental research.
Understanding single-celled life has several benefits. Firstly, they are essential in nutrient cycling. Their metabolic processes contribute significantly to the ecological balance. Secondly, single-celled organisms inform the field of biotechnology. They have become an innovative resource in genetic engineering and sustainable practices. This shows their potential to address global challenges, like food security and environmental sustainability.
Another crucial consideration regarding the conclusion is the implications for future research. There is immense scope to explore the genetics and adaptability of these organisms. Current advancements in technology can lead to further revelations about their impact on health and disease. As researchers continue to delve into this field, greater insights will emerge, making it imperative to stay informed of these developments.
"Single-celled organisms are not just the simplest forms of life; they are also among the most ingenious. Their processes underlie critical ecological functions and biotechnological applications."
Thus, this conclusion emphasizes not only a summary of key points but also the prospects that studying single-celled organisms holds. Acknowledging their importance offers a pathway to unlocking new knowledge and practical applications.