Exploring Schrödinger: The Essence of Quantum Mechanics
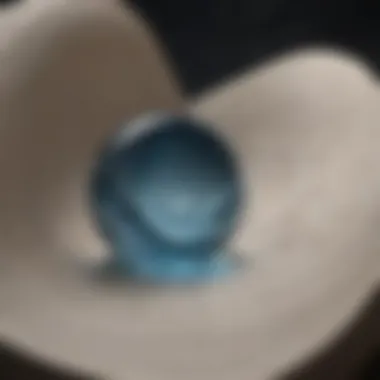
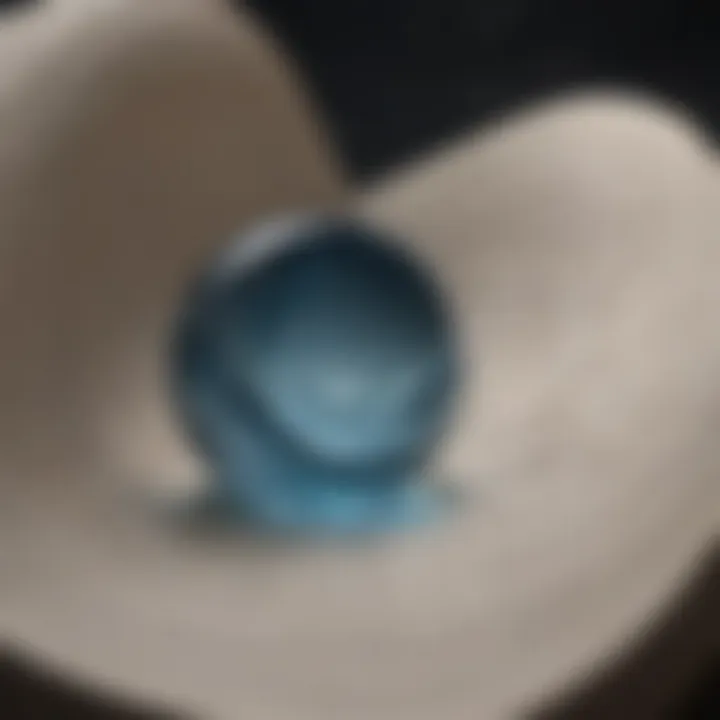
Intro
In the realm of physics, few names resonate as profoundly as Erwin Schrödinger. His contributions to quantum mechanics not only laid the groundwork for modern physics but also challenged the very fabric of how we understand reality. Schrödinger, through his notable equations, pushed the boundaries of science and philosophy, compelling us to reconsider our perceptions of物质 and consciousness.
With a work that coordinates abstract mathematical principles and complex physical realities, Schrödinger's theories serve as a lens through which we can glimpse the peculiarities of the quantum world. In this exploration, we will dive into the depth of his findings, analyze their significance in today's scientific narrative, and piece together how these discoveries continue to shape our understanding of the universe.
This article provides a detailed examination of the intricate developments in quantum mechanics, giving attention to Schrödinger's unique perspectives and highlighting where his work stands amid modern physics. From the foundational Schrödinger equation to the philosophical implications of wave functions, we aim to unravel the threads that connect his research with both theoretical and practical applications in contemporary science.
Prelude to Schrödinger's Legacy
Understanding the legacy of Erwin Schrödinger goes beyond merely recognizing his equations and theories. It dives into the essence of a scientific era that challenged the traditional frameworks of physics and opened doors to a multitude of possibilities in various fields. Schrödinger's contributions to quantum mechanics are not just academic pursuits; they represent radical shifts in our appreciation for how the universe operates at its most fundamental level.
One of the fascinating aspects of Schrödinger's work is how it elegantly weaves mathematical rigor with philosophical inquiry. His famous wave equation isn't merely a formula; it's a glimpse into the unpredictable nature of reality. This section acts as a gateway to grasp not only Schrödinger's genius but also the broader implications of his work. Readers gain insight into why his contributions resonate throughout contemporary research and education, shaping our very understanding of matter and existence.
By exploring the key components of Schrödinger's legacy, including both the historical developments that laid the groundwork and the man himself, we can build a more holistic view. The nuances of his theories challenge us to rethink past assumptions regarding nature, causality, and the very act of observation.
Therefore, taking a closer look into this legacy is essential for anyone interested in physics, philosophy, or the intertwining paths of science and society.
The Man Behind Quantum Mechanics
Erwin Schrödinger, born in Vienna in 1887, blossomed in a world fraught with paradox and uncertainty. Despite his sometimes elusive nature, he had a profound effect on the scientific landscape. His training wasn't just limited to physics; he dabbled in various other disciplines, including philosophy, which ultimately influenced his views on quantum mechanics.
After attaining his doctorate in 1910, Schrödinger worked in diverse scientific institutions across Europe. Notably, he contributed substantially to thermodynamics and statistical mechanics. But his most significant breakthrough came in 1925, when he arrived at what is now known as Schrödinger's Equation. This equation transformed quantum mechanics by providing a mathematical framework to understand wave functions—essentially the probabilities of a particle's position.
However, it wasn't just about equations for Schrödinger; he often pondered over the implications of his findings. He famously said, "The thinker and the thought are one,” emphasizing a holistic view of existence that questions the separation between observer and observed.
Historical Context of Quantum Theory
To fully appreciate Schrödinger's contributions, we must step back to the early 20th century when physics was at a turning point. Classical mechanics, which had dominated for centuries, faced challenges from experiments that unveiled the bizarre behaviors of light and atoms. Prior to Schrödinger, figures like Max Planck and Albert Einstein had illuminated aspects of quantum theory, but the field lacked a unified framework.
As new technologies emerged, scientists began to encounter phenomena that didn't fit neatly within classical explanations. Experiments, like the double-slit experiment, revealed the wave-particle duality of light, leaving many scratching their heads. It was Schrödinger's insights that bridged these mysterious gaps.
His wave mechanics provided an innovative alternative to the then-prevalent matrix mechanics proposed by Werner Heisenberg. The historical tensions between these schools of thought not only advanced the field but posed philosophical questions about reality, making it a rich period for scientific inquiry.
This historical background sets the stage for an understanding of how Schrödinger's equation revolutionized quantum mechanics, influencing both theoretical interpretations and practical applications. The interplay of theories proposed during this time reflects the vibrant debate over the fundamentals of reality, ultimately leading us to advancements in technology and modern physics we see today.
Schrödinger's Equation and Its Significance
The Schrödinger equation lies at the heart of quantum mechanics, serving as the foundation upon which a vast array of quantum phenomena are examined. Understanding this equation is akin to learning a new language; it opens doors to comprehending complex interactions at a subatomic level. This section will illuminate the significance of Schrödinger’s Equation, its derivation, applications, and the underlying mathematical framework that supports it.
Derivation of the Schrödinger Equation
Deriving the Schrödinger equation requires a blend of classical mechanics' principles and innovative quantum insights. The equation itself articulates how the quantum state of a physical system changes over time. Schrödinger built this framework on the compelling notion that instead of dealing just with particles, one should consider their wave-like behaviors.
To start, we invoke the principle of superposition, where multiple states can exist simultaneously. Schrödinger took Max Planck’s concept of quantization and combined it with the wave behavior of electrons, leading to the wave function, denoted by ( \Psi ). The equation takes the form:
[ H \Psi = E \Psi ]
where ( H ) is the Hamiltonian operator (representing total energy), ( E ) is the energy eigenvalue, and ( \Psi ) is the wave function of the system. This derivation reflects the duality between wave and particle, an idea that was radical at its inception and remains profound to this day.
Applications in Quantum Mechanics
The implications of Schrödinger's equation extend beyond theoretical considerations; they are practically vital in various domains. One key application lies in quantum chemistry, where it provides insight into atomic and molecular structures. For instance, when exploring chemical bonding, the equation reveals how electrons distribute around atomic nuclei, paving the way for understanding chemical reactions and material properties.
Additionally, the equation plays a critical role in predicting behaviors in semiconductor physics and material science. Devices like transistors and diodes—the building blocks of modern electronics—rely heavily on principles arising from the Schrödinger framework.
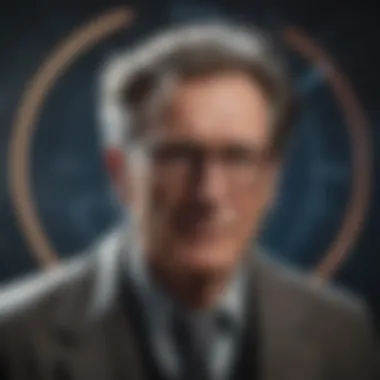
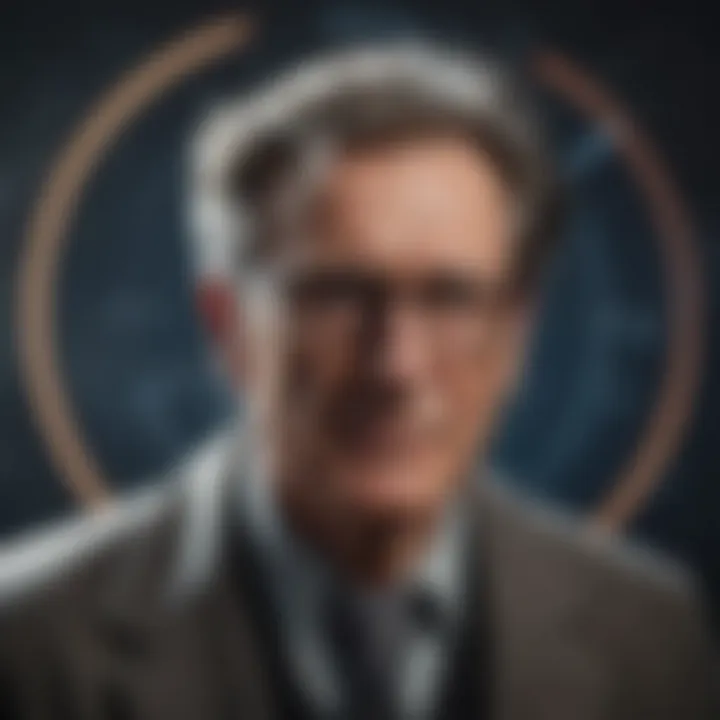
The applications are not limited to material science. Schrödinger's work enables advancements in optics and electromagnetism, contributing to technologies like lasers and MRI machines, thus illustrating the profound reach of his ideas.
Mathematical Framework Explained
The mathematical structure of the Schrödinger equation is as significant as the phenomena it describes. The equation is typically expressed in two forms: time-dependent and time-independent.
- Time-Dependent Schrödinger Equation: This version describes how ( \Psi ) evolves over time and is expressed as:[ i \hbar \frac\partial \Psi\partial t = H \Psi ]Here, ( i ) is the imaginary unit, and ( \hbar ) (h-bar) is the reduced Planck constant. This equation considers all dynamics of quantum systems over time.
- Time-Independent Schrödinger Equation: In scenarios where systems do not change with time (steady states), this form is more applicable:[ H \Psi = E \Psi ]
Understanding this mathematical framework is paramount for delving deeper into quantum mechanics. It serves as a toolkit, equipping scientists and engineers to unravel complexities within various fields.
Quantum mechanics may seem daunting at first, but the clarity provided by Schrödinger's equation allows for a structured approach to understanding the universe on a fundamental level.
In summary, Schrödinger's equation is more than just a mere formula; it encapsulates the quantum world's intricacies and allows for advancements that continue to influence our technologies and scientific understanding. Each application arises from a robust mathematical foundation that merges theory with reality.
Philosophical Interpretations of Quantum Mechanics
Philosophical interpretations of quantum mechanics act like the wind beneath the wings of modern physics, stirring up both intrigue and debate. As we plunge into this realm, we encounter a labyrinth of concepts that shape how we understand the universe's fundamental nature. The importance of philosophical interpretations lies in their ability to provide frameworks for the perplexing principles underlying quantum mechanics. Even seasoned physicists sometimes squint into the fog of quantum phenomena, and these interpretations help unearth meaning where raw mathematics may fall short. In this section, we will explore three primary interpretations that have sparked conversation and contemplation: the Copenhagen interpretation, the Many-Worlds interpretation, and the thought experiment famously known as Schrödinger's Cat.
Copenhagen Interpretation
The Copenhagen interpretation, which emerged from the minds of Niels Bohr and Werner Heisenberg in the early 20th century, has long been the dominant theory in the discussion of quantum mechanics. Its essence comes down to a rather provocative idea: particles don’t have definite properties until they are measured. This leads to the somewhat unsettling conclusion that reality might not be as straightforward as it seems. When a quantum particle is not being observed, it exists simultaneously in multiple states—this phenomenon is often represented by the concept of wave function.
This interpretation highlights the inherent probabilistic nature of quantum events, wherein outcomes can only be predicted with certain likelihoods and are not predetermined. For instance, imagine tossing a coin: before it lands, it exists in a superposition of heads and tails, much like a quantum particle inhabits multiple states until observed. The philosophical implications are rich; it raises questions about the role of the observer in defining reality itself. In a way, it draws a line between the objective and subjective realms, leaving many eager to delve deeper into its human-centric ramifications.
Many-Worlds Interpretation
Enter the Many-Worlds interpretation, proposed by Hugh Everett III in the 1950s. Unlike the Copenhagen interpretation, which relies on the act of observation to collapse the wave function, the Many-Worlds theory argues that all possible outcomes of a quantum event actually occur, each in its own disparate universe. This paints an intriguing yet complex picture of reality, where every decision or quantum event spawns a new universe.
Imagine a scenario where you decide whether to go out for pizza or stay in and cook. In the Many-Worlds framework, there exists a universe where you’ve snatched that pepperoni pizza and another universe where you’ve opted for a homemade meal. This interpretation challenges elimination of any potential outcomes and posits an ever-expanding multiverse. Beyond just a playful notion, it forces us to confront profound notions about existence, identity, and our understanding of free will. Philosophically, it triggers discussions about what it means to be human in a cosmos that could be teeming with alternative versions of ourselves.
Schrödinger's Cat: A Thought Experiment
Schrödinger's Cat encapsulates the conundrums of quantum mechanics in a strikingly vivid thought experiment. Imagine a cat, placed inside a sealed box along with a radioactive atom, a Geiger counter, and a vial of poison. If the atom decays, it triggers the Geiger counter, breaking the vial and resulting in the cat's demise. However, until we coldly open the box and peek inside, quantum mechanics suggests the cat is simultaneously alive and dead—a state of superposition.
This thought experiment does not just configure an unsettling image but prompts essential inquiries about the nature of reality itself. It raises questions about when a quantum system transitions from superposition to a single outcome, challenging our conventional understanding of death, existence, and observation. The paradox of Schrödinger's Cat illustrates the friction between quantum mechanics and classical intuition, spotlighting the intricate dance between knowledge and mystery. It’s this provocative blend of scientific rigor and philosophical depth that continues to inspire a dual investigation into the nature of reality, urging thinkers from all walks of life to grapple with the enigmatic laws that govern our universe.
"The most incomprehensible thing about the universe is that it is comprehensible."
- Albert Einstein
Observing these interpretations opens the floor for thought-provoking discussions. As we advance deeper into quantum mechanics, the interplay of philosophy and science becomes clearer, each lending insight to the other in our pursuit of understanding the cosmic ballet.
Impact of Schrödinger's Work on Modern Physics
The significance of Schrödinger's contributions cannot be understated when considering the evolution of modern physics. His theories and equations not only revolutionized the understanding of atomic and subatomic phenomena but also laid down the foundation for various applied fields today. Outlining the impact of his work reveals how deeply it intertwines with contemporary science and technology.
Advancements in Quantum Chemistry
One of the key breakthroughs influenced by Schrödinger’s work is the field of quantum chemistry. His wave equation provides an essential framework for interpreting chemical interactions on a quantum level. By applying Schrödinger's equation, chemists can derive the electronic structure of atoms and molecules, leading to a better understanding of their chemical properties and reactions.
This understanding opens up new avenues in many practical applications such as drug discovery, materials science, and nanotechnology. For instance, in drug design, computational methods derived from quantum mechanical principles allow scientists to model how a drug molecule interacts with its biological target, significantly speeding up the process of finding effective treatments.
Overall, Schrödinger helped bridge the gap between physics and chemistry, reinforcing the necessity of using quantum mechanics as a tool in modern chemistry.
Quantum Computing and Information Theory
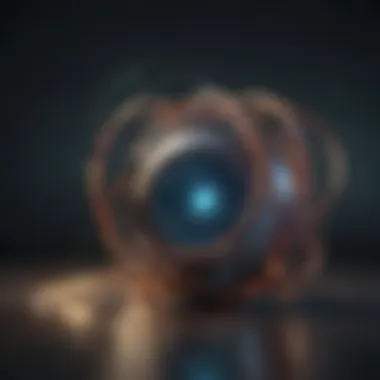
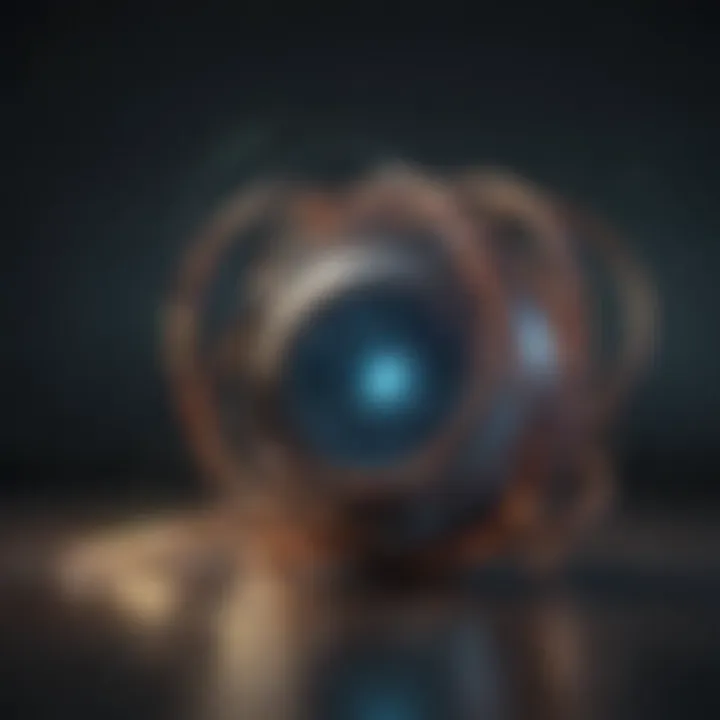
Another monumental advancement is in the realm of quantum computing and information theory. The principles postulated by Schrödinger inform the very basis of how quantum bits, or qubits, function. Unlike classical bits, qubits can exist in multiple states simultaneously thanks to the superposition principle introduced by Schrödinger.
This unique property enables quantum computers to perform complex calculations at speeds unattainable by traditional computers. Applications of such technology can range from cryptography to optimizing supply chains, signifying a paradigm shift in computation and data handling.
The potential for quantum technology to transform industries is immense. As research progresses, the once-theoretical concepts laid out by Schrödinger are becoming increasingly relevant, pushing the boundaries of what is technically feasible today.
Contemporary Physics Research Inspired by Schrödinger
Scrutiny of Schrödinger’s theories continues to inspire fresh lines of inquiry in contemporary physics research. His works encourage physicists to delve deeper into fundamental questions about the nature of reality itself. For example, the ongoing debates around quantum entanglement and non-locality can trace their intellectual lineage back to the ideas Schrödinger presented.
As new experimental technologies emerge, researchers are able to investigate these phenomena directly. Quantum entanglement, for example, has been harnessed in experiments that expand our understanding of the fabric of reality, and fuel developments in quantum networks.
The spirit of inquiry that Schrödinger embodied drives researchers to explore not only the measurable aspects of quantum mechanics but also its philosophical implications. Furthermore, initiatives in fields like quantum thermodynamics and quantum gravity owe a debt to the groundwork laid by Schrödinger, emphasizing how ideas from one era can ripple through time to electrify future research.
"The physicist cannot ignore the fact that through his work he enters the realm of philosophy.” — Erwin Schrödinger.
Thus, the contributions made by Erwin Schrödinger to modern physics transcend his era, shaping disciplines and methodologies that resonate with contemporary scientific exploration.
Challenges and Critiques of Schrödinger's Theories
The discourse surrounding Schrödinger's contributions to quantum mechanics isn't just a showcase of scientific achievements; it's also a space where conflicting ideas collide. Evaluating these challenges and critiques is essential not only for understanding his work but also for grasping the overall evolution of quantum mechanics. This section rises as a pivotal point in our discussion, shedding light on the criticisms aimed at Schrödinger's theories and encouraging a dialogue that extends well beyond the realm of physics.
Conflicts with Classical Physics
One of the core challenges that Schrödinger's theories face lies in their tension with classical physics. Traditional physics, with its solid ground in Newtonian mechanics, shaped how the world was viewed for centuries. Here, entities were predictable, and outcomes followed a logical progression. When Schrödinger proposed the concept of wave functions and particle superposition, the proverbial applecart was upset
- Determinism vs. Probabilism: In the classical sense, if you know the initial conditions precisely, you can predict future states. This deterministic view heavily clashes with the probabilistic interpretation present in Schrödinger’s work. The famous wave function, which suggests a range of possibilities rather than certainties, shakes the very foundations of classical thought.
- Wave-Particle Duality: Schrödinger's equation describes entities as both waves and particles, but this duality created a philosophical conundrum. How can something be two distinct things at once? This question baffled both physicists and philosophers, creating friction between classical beliefs and novel quantum concepts.
"The quantum world challenges our basic intuitions. It stands to remind us that our familiar notions of reality can often be misleading."
— A leading figure in quantum philosophy
Responding to these critiques requires acknowledging that Schrödinger himself was initially skeptical of the full implications of his work. He famously expressed reservations about the random nature of quantum phenomena, hinting at the throbbing conflicts between his personal beliefs and the scientific principles he set forth.
Debates on Quantum Mechanics Interpretations
The philosophical debates ignited by Schrödinger’s theories do not end with the friction against classical physics; they continue to shape quantum mechanics to this day. Two major interpretations often draw lines in the sand among physicists and philosophers alike.
- Copenhagen Interpretation: Associated largely with Niels Bohr, this viewpoint posits that quantum particles do not have definite properties until measured. Schrödinger’s equation provides the foundation, but its implication—that observation alters reality—drew heavy criticism for being counterintuitive and even nonsensical to some.
- Many-Worlds Interpretation: Alternatively, this concept proposed by Hugh Everett takes a radical approach. It suggests every possible outcome of a quantum event actually happens, just in different branching realities. Such a notion puts a strain on the imagination. Critics argue it spirals into an infinite regression of worlds, which can be daunting to consider.
Ultimately, these debates fuel a vibrant ongoing dialogue among physicists and philosophes. They not only challenge Schrödinger's conclusions but also enrich our understanding of the complexity and depth found within quantum theory. The challenges to Schrödinger's theories remind us that science is not just about discovering new truths but also reexamining and grappling with existing beliefs.
Contributions to Other Fields
Erwin Schrödinger's work doesn't just sit neatly in the realm of physics; its ripples spread across various fields, notably biology and philosophy. Understanding these contributions highlights the far-reaching implications of his scientific interpretations, showing how a single mind can spark change in different domains.
Schrödinger's Influences in Biology
Schrödinger's foray into biology primarily surfaced in his book, What Is Life? published in 1944. This text stirred the scientific community by contemplating life’s unique characteristics through the lens of physics. He proposed that living organisms are not simply complex machines but exhibit distinct behaviors guided by quantum mechanics and thermodynamics.
Within this book, he introduced the concept of genetic information as a blueprint for life. His ideas paved the way for modern molecular biology, challenging researchers to view the processes of heredity and evolution through a new, quantum-inspired perspective.
- Genetic Code: Schrödinger suggested that life at its core involves orderly information storage, similar to how data is structured. This idea laid groundwork for breakthroughs like the discovery of DNA’s structure.
- Entropy and Life: He argued that living systems are organized entities that maintain their order by constantly exchanging energy with their environment, thus combating entropy. This concept connects deeply with contemporary ecological and evolutionary theories.
- Quantum Biology: The emergence of a field known as quantum biology explores phenomena like photosynthesis and avian navigation, suggesting that quantum mechanics might underlie biological functions in ways yet to be fully understood.
Schrödinger effectively opened a door, urging biologists to consider quantum physics when investigating life at a molecular level.
Philosophy and Ethics: Schrödinger's Perspective
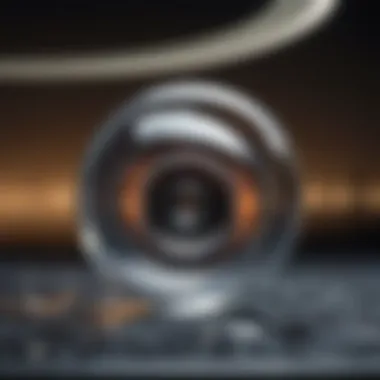
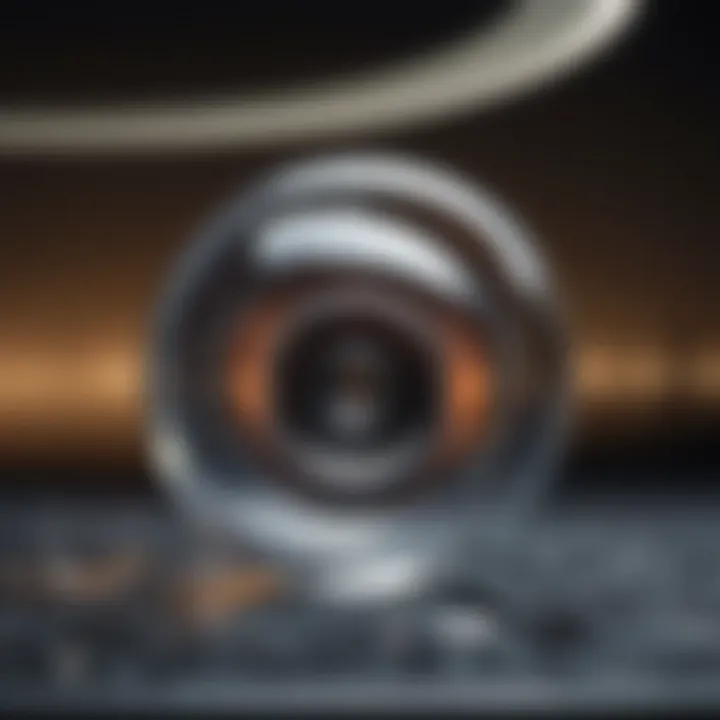
Turning towards philosophy, Schrödinger was not just a physicist; he was a thinker deeply engaged with the implications of his work for our understanding of existence. His thoughts often grazed the boundaries of ethics and metaphysics. He was known to wrestle with mechanical reductionism—the notion that everything can be understood solely through its physical components.
- Unity of Nature: Schrödinger advocated for a more holistic view, emphasizing the interconnectedness of all things. He stated, "Consciousness cannot be accounted for in physical terms. For consciousness is absolutely distinct from it." In doing so, he challenged reductionist views, suggesting that the mind has qualities that elude physical quantification.
- Ethical Considerations: His engagement with ethics derives from this belief in interconnectedness. If everything is interlinked, we have a moral duty to honor our connection with the world, leading to discussions on environmental ethics as well as the philosophy of mind.
“The fundamental laws necessary for the mathematical treatment of a large part of physics and the whole of chemistry are thus completely known, and the difficulty lies only in the fact that these laws are expressed in terms of wave functions.”
In the broader scope, Schrödinger's writings often serve as a bridge between science and philosophy. His emphasis on consciousness and ethics urges us to look beyond empirical data and acknowledge the moral implications arising from scientific advancements.
Understanding these contributions offers a window into how Schrödinger's theories resonate through several disciplines. His insights not just enriched physics but also laid foundations for future inquiries in biology, philosophy, and even ethics, making his legacy truly interdisciplinary.
Schrödinger's Lasting Influence on Future Generations
Erwin Schrödinger’s contributions have created ripples across many fields since he first introduced his revolutionary ideas. The impact of his theories transcends mere equations and interpretations; they have become a touchstone for how we understand not just physics, but a host of related disciplines. In the context of education and ongoing research, the influence of Schrödinger’s work can’t be overstated. His legacy serves as a foundation for many principles in quantum mechanics, shaping curricula and inspiring new generations of scientists and thinkers.
Educational Impacts on Science Curricula
Within the classroom, Schrödinger’s theories have become essential ingredients of modern science education. From high school textbooks to university courses, his contributions are woven into the fabric of how quantum mechanics is taught. Students now encounter the concepts borne from his ideas quite early in their academic journeys. The emphasis on Schrödinger’s Equation as a fundamental tool in quantum theory prepares students to engage deeply with the subject.
Moreover, educators often connect Schrödinger's work to real-world applications, fostering curiosity and critical thinking. Learners explore complex topics, such as wave-particle duality and uncertainty principles through Schrödinger's frameworks. This approach solidifies theoretical concepts, making them tangible and relatable.
- Many institutions have adopted inquiry-based learning strategies to engage students with Schrödinger's theories, ensuring a hands-on experience with abstract ideas.
- Workshops and interdisciplinary courses often highlight how Schrödinger's work lays the groundwork for modern physics, biology, and chemistry, bridging gaps between diverse fields.
The educational impact of Schrödinger's work has not just been confined to physics. His insights have influenced biophysics, bioinformatics, and even the ethical discussions that revolve around scientific advancements. Such interdisciplinary learning is critical; it fosters a more holistic understanding of both science and its societal implications.
Continued Research and Framework Development
Research influenced by Schrödinger’s insights continues to flourish. Scientists and researchers find themselves delving deeper into the realms of quantum mechanics, often inspired directly by his equations and theories. The exploration of quantum entanglement, quantum computing, and even advances in nanotechnology often roots back to the questions that Schrödinger posed.
In the arena of quantum computing, for instance, methodologies that stem from Schrödinger’s principles are shaping new digital frontiers. With powerful applications that promise to revolutionize industries, the relevance of his ideas remains robust.
Moreover, researchers today often revisit the philosophical quandaries he raised. Questions of measurement, reality, and observation in quantum mechanics push the envelope of scientific inquiry further, ensuring that Schrödinger’s inquiries still invigorate discussions within the scientific community. This cycle of questioning and discovery highlights how influential Schrödinger has been:
- Ongoing projects in quantum biology are beginning to decipher processes such as photosynthesis and avian navigation through the lens of quantum effects, echoing the ideas Schrödinger brought to light.
- Framework developments in statistical mechanics and elements of thermodynamics often pay homage to Schrödinger’s philosophical insights about entropy, establishing bridges between varying fields of study.
In summary, Schrödinger's legacy is not merely a chapter in the history of science; it is a continually evolving narrative that shapes both education and research. His influence transcends numbers on a page, inviting future generations to question, explore, and innovate.
End and Reflection on Quantum Mechanics
As we draw to a close on our exploration of Schrödinger's profound contributions to quantum mechanics, it's essential to recognize how his work continues to shape our understanding of the universe. The journey through Schrödinger's principles and theories not only underscores the evolution of scientific thought but also ignites the curiosity necessary for future advancements in physics.
Schrödinger's equation, his philosophical musings, and the rich debates sparked by his theories serve not merely as historical artifacts but as living frameworks that influence today’s scientific inquiry. This reflects a dual nature where foundational principles merge with modern exploration—an intersection where students and researchers find fertile ground for innovation. This era of quantum mechanics is characterized by complexity and depth, yet it offers a clearer lens through which to observe phenomena previously deemed unfathomable.
The Future of Quantum Theory
Looking ahead, quantum theory stands at the precipice of groundbreaking advancements. With the rise of technologies like quantum computing, one can visualize rather quickly how Schrödinger's ideas are poised to become even more significant. Abundant challenges remain, of course. Issues surrounding entanglement, uncertainty, and the interpretations sparked by Schrödinger's famous cat continue to fuel discussions in scientific circles. Researchers are beginning to unravel the mysteries that were once the exclusive domain of theoretical discussion.
Several noteworthy trends are visible as we veer into the future:
- Quantum computing capacity is expected to skyrocket, enabling calculations that today are simply impossible.
- Entanglement technologies may lead to secure communication methods, revolutionizing fields like cybersecurity.
- The advent of quantum biology may usher in new understandings of biological processes at the quantum level, potentially reshaping areas like medicine and ecology.
Ultimately, the future of quantum theory rests on the foundations laid by Schrödinger and his contemporaries. Developments in these domains will likely challenge and expand upon established norms, propelling the field to torrential heights.
Schrödinger’s Legacy in Modern Science
Schrödinger's influence extends far beyond his own time; it casts a long shadow over modern scientific inquiry. The rigorous frameworks he introduced, such as the wave-function approach, have permeated various branches of science. In today’s academia, his principles are not only taught to aspiring physicists but also employed by professionals engaged in cutting-edge research.
His legacy flourishes in:
- Quantum Chemistry: The tools derived from his equation allow chemists to predict molecular behavior with profound accuracy, aiding in the design of new materials and drugs.
- Theoretical Physics: The dialogues initiated by Schrödinger's work stimulate fresh perspectives and challenge existing paradigms, encouraging scholars to venture beyond conventional wisdom.
- Interdisciplinary Studies: Fields as diverse as biology and sociology utilize frameworks inspired by quantum mechanics, fostering innovative methodologies that bridge traditionally isolated disciplines.
"The Schrödinger equation has become a foundational aspect of quantum mechanics education, setting the stage for countless discoveries and developments across scientific fields."
In summary, as we reflect on Schrödinger's manifold contributions and their implications for quantum theory and modern science, we ascertain that his work remains not just a beacon of knowledge but a dynamic influence that will continue to inspire generations of scholars and professionals. His theories serve as a cornerstone of scientific progress, prompting inquiry and challenging our perceptions of reality itself.