Exploring the Future of Quantum Computing Technology
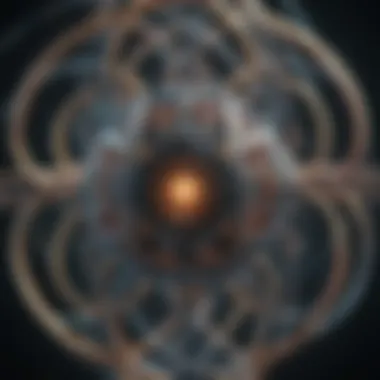
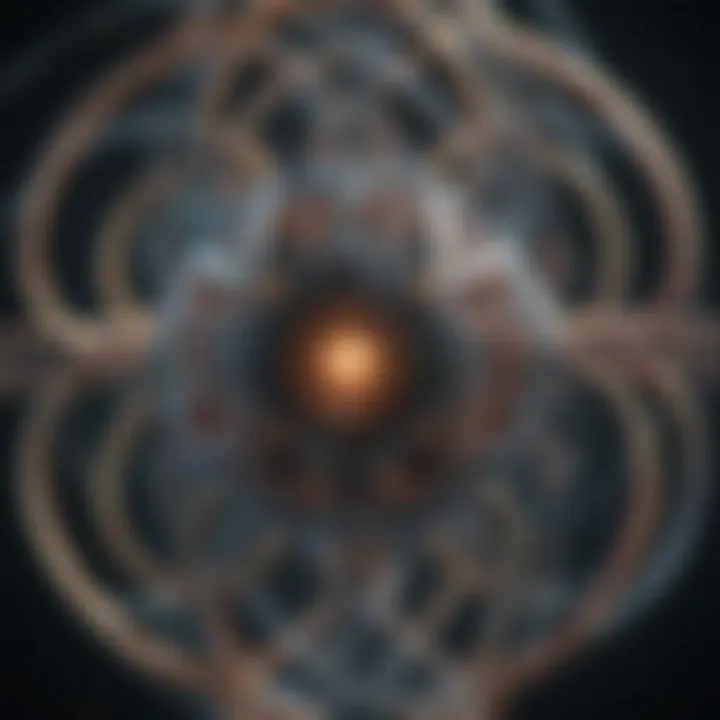
Intro
Quantum computing is not just a buzzword tossed around at tech conferences; it’s a fundamental departure from a world dominated by classical computation. In essence, while traditional computers process information in binary, quantum computers tap into the curious and often unfathomable realm of quantum mechanics. Quantum bits, or qubits, represent the core of this new computational framework. They can exist in multiple states at once, allowing for complex calculations that might take classical computers an eternity to solve.
This article aims to peel back the layers of quantum computing, offering insights into its key concepts, significant advancements, and its potential to transform various industries. Through our exploration, we’ll also contrast these innovative systems with classical computing, helping to elucidate why this new technology holds so much promise.
Key Research Findings
Overview of Recent Discoveries
The field of quantum computing is evolving at an astonishing pace. Recent breakthroughs in quantum error correction and algorithm design are paving the way for more practical applications. One of the noteworthy developments is the emergence of quantum supremacy—where a quantum computer performs a specific task that a classical computer cannot accomplish within a reasonable timeframe. Google’s Sycamore processor achieved this milestone in 2019, demonstrating that particular quantum problems could be solved exponentially faster than their classical counterparts.
Another crucial discovery involves advancements in quantum networks. Researchers are actively working on achieving quantum entanglement over greater distances, which could eventually lead to the creation of a quantum internet. This concept not only heightens security protocols through quantum encryption but also enhances data transmission efficiency.
Significance of Findings in the Field
These discoveries hold profound implications. Increased stability and error correction in quantum operations can potentially unlock powerful algorithms for cryptography, complex modeling, and even machine learning. As quantum technology matures, different sectors, such as pharmaceuticals and finance, may reap significant benefits by speeding up processes far beyond what is possible today.
"Quantum computing promises potential we are only beginning to scratch on the surface. Each breakthrough is a step towards a paradigm shift in how we understand computation."
Breakdown of Complex Concepts
Simplification of Advanced Theories
To grasp quantum computing, one must become familiar with a few core principles. Quantum superposition and entanglement are fundamental. Superposition allows qubits to exist in multiple states simultaneously, as opposed to classical bits, which can only be in one state at a time, like a light switch that is either on or off.
Entanglement, on the other hand, is the phenomenon where multiple qubits become interconnected. Changes to one qubit affect another, regardless of distance—like a pair of magical dice that land on the same number no matter how far apart they are rolled. This interconnectedness enables quantum computers to solve complex problems with unparalleled efficiency.
Visual Aids and Infographics
For a clearer understanding, visuals can play a pivotal role when trying to comprehend these theories. Elements like charts or diagrams demonstrating a circuit composed of qubits can illustrate how a quantum algorithm functions. Simple infographics can be designed to depict voting based on superposition, to clarify how decisions are made in a quantum realm versus classical binary outcomes.
These aspects not only enhance comprehension but also invite readers to visualize the transformative power of quantum computing.
In summation, the world of quantum computing is a fascinating tapestry of complexity and potential, interwoven with discoveries that challenge our understanding of computation. As we continue with this discussion, the subsequent sections will delve deeper into how these facets interplay with advancements, challenges, and the broader implications for society.
Defining Quantum Computing
In the labyrinth of technological advancements, quantum computing stands out like a lighthouse amidst a storm. To understand its impact, it is critical to define what quantum computing really means and why it’s a hot topic today. This section will dissect the fundamental aspects and provide insight into its significance in the modern digital landscape.
The Essence of Quantum Mechanics
Quantum mechanics, the backbone of quantum computing, offers a fascinating glimpse into the behavior of matter and energy at the smallest scales. At its core, it introduces concepts that upend traditional thinking. For instance, the idea of superposition allows quantum bits, or qubits, to exist in multiple states at once. Think about it like flipping a coin; while it's in the air, it’s both heads and tails simultaneously. This quirky behavior underpins the incredible processing power of quantum computers.
In a classical world, we are accustomed to binary states: either a 0 or a 1. However, in quantum mechanics, the probabilistic nature adds layers of complexities and potentials. By utilizing phenomena such as entanglement, where qubits become interlinked and the state of one can instantaneously influence another, we open doors to computations that were once considered a pipe dream. The beauty of quantum mechanics isn't just in its equations; it’s in how these principles can revolutionize the processing of information.
Fundamental Principles of Quantum Computing
When we dive deeper into quantum computing, several fundamental principles rise to the surface, shaping the architecture and functionality of quantum systems. Understanding these principles is essential for grasping how quantum computers differ from their classical counterparts.
- Superposition: This principle, as discussed earlier, is where a qubit, unlike a traditional bit, can represent both 0 and 1 at the same time. This property exponentially increases the computational power as more qubits are added, allowing for more complex calculations to be performed simultaneously.
- Entanglement: This phenomenon enables qubits that are entangled to become intertwined in a way that the state of one qubit will directly affect the state of another, no matter the distance between them. It’s like having a pair of magical dice; no matter where you roll one, the other always copies the outcome. This interconnectedness of qubits greatly enhances the capacity for processing information across quantum systems.
- Quantum Interference: Through constructive and destructive interference, quantum algorithms leverage paths of computation that enhance the probability of desired outcomes while canceling out less favorable ones. It’s akin to a symphony where various instruments harmonize to produce a beautiful melody from what would otherwise be a cacophony of sounds.
These principles collectively differentiate quantum computing from classical computing, offering not just speed but a fundamentally different manner of thinking about computation itself. They entail a paradigm shift that could redefine industries and problem-solving scenarios in ways we've yet to fully grasp.
"Quantum computing is a game-changer, reshaping the very fabric of computational possibilities."
In unraveling these concepts, the promise of quantum computing becomes clearer. It’s not just about performing calculations faster, but about reimagining the scope of what problems can even be tackled. This conversation not only fosters excitement among technologists but also hints at the immense potential for diverse fields, from cryptography to material science.
Historical Context
Understanding the historical context of quantum computing is crucial for grasping its significance in the broader landscape of technology. This aspect reveals the evolution of thought and innovation that led to the current capabilities and applications of quantum computing, illustrating not only technical advancements but also shifts in theoretical understanding.
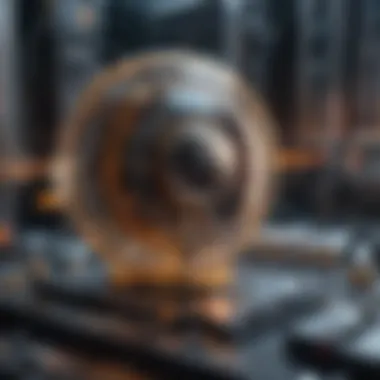
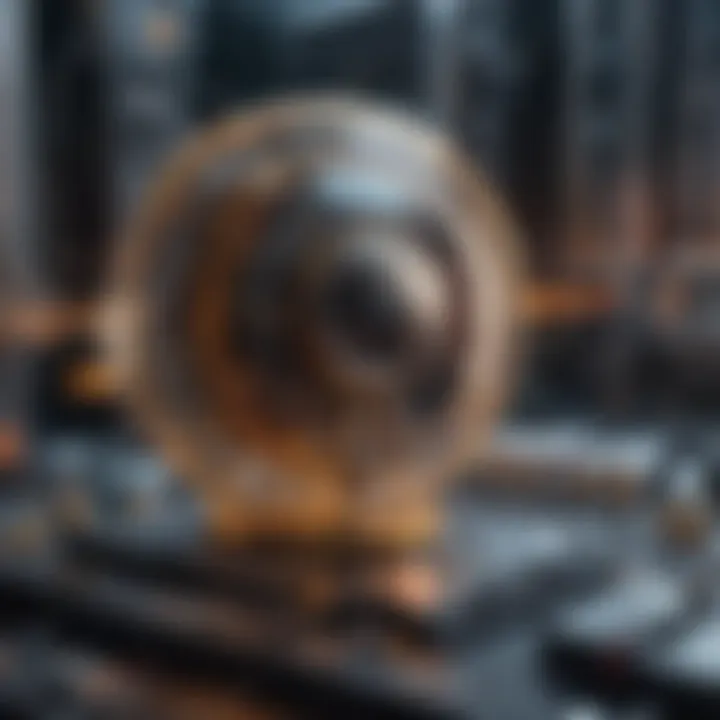
The Evolution of Computing Technology
From the inception of the first mechanical devices, like the abacus, to today's lightning-fast computers, the trajectory of computing technology has been anything but linear. Each advancement built on the previous layer, revealing a complex tapestry of ingenuity and necessity driven by societal demands.
In the mid-20th century, classical computing began to flourish. The advent of transistors and integrated circuits revolutionized how data could be processed, leading to the computers we know today. However, this technology had its limits. Classical computers manipulate bits, the basic units of data that represent a state of either 0 or 1. This binary framework maxed out in terms of complexity and speed when tasked with solving intricate problems. As theorists pondered on these limits, they turned their gaze towards a novel frontier that nature itself had long whispered about: quantum mechanics.
A pivotal moment unfolded in the 1980s, as physicist Richard Feynman and computer scientist David Deutsch posited that a new kind of computing could transcend traditional computing barriers. They suggested using the principles of quantum mechanics, a realm where particles can exist in multiple states simultaneously, to create a radically different computer architecture. This was not just about speeding up computations; it was about rethinking computation itself.
Key Milestones in Quantum Computing Development
The journey of quantum computing is dotted with significant milestones that have propelled this field forward. Here are a few that stand out:
- 1981: Richard Feynman's seminal lecture introduced the concept of a quantum computer, emphasizing the limitations of classical computing when modelling quantum systems.
- 1985: David Deutsch formulated a universal quantum computer model, laying the groundwork for quantum algorithms.
- 1994: Peter Shor's algorithm demonstrated that quantum computers could factor large integers exponentially faster than the best-known classical algorithms, igniting interest from both academia and industry.
- 2001: IBM and Stanford University conducted the first experimental demonstration of a quantum algorithm, showcasing quantum computing's practical potential.
- 2019: Google claimed to achieve "quantum supremacy" by demonstrating that their 53-qubit processor, Sycamore, could perform a specific calculation faster than the world’s fastest classical supercomputer.
Each of these milestones sheds light on the immense potential of quantum computing, underscoring its role as a transformative force across various domains.
"Quantum computing is not just a step forward; it is a leap into a new paradigm, reshaping our understanding of computation itself."
In summary, the historical context of quantum computing is pivotal. It outlines the limitations of classical technologies while highlighting pivotal breakthroughs in both thought and experiment. Understanding this lineage equips researchers, educators, and technology enthusiasts alike with a perspective that is essential for comprehending where quantum computing is headed and its implications for the future, as well as how these advances will redefine problem-solving approaches across numerous fields.
Key Components of Quantum Computing
To grasp the significance of quantum computing, one must first appreciate its fundamental components. These elements serve as the backbone of the entire quantum computational framework, setting it apart from classical computing. Understanding these key components helps delineate the advantages quantum computing offers, as well as the challenges it presents.
Qubits: The Building Blocks
The first and foremost component of quantum computing is the qubit. Unlike classical bits, which hold a binary state of either 0 or 1, qubits have the unique ability to exist in a superposition of states. This means a qubit can represent both 0 and 1 simultaneously, a feature that exponentially increases the computational power of quantum systems.
Imagine flipping a coin. While it spins, it's neither heads nor tails but something in-between. This blend of states enables quantum computers to perform multiple calculations at once, offering a profound leap in processing speed and efficiency.
Moreover, qubits can exhibit entanglement, a phenomenon where the states of two or more qubits become linked. This interdependence allows for more complex operations that classical bits simply cannot accomplish. For instance, manipulation of one qubit instantaneously affects its entangled partner, no matter the distance separating them, leading to faster information exchange and processing capabilities that classical systems can only dream of.
Quantum Gates and Circuits
Building upon qubits are quantum gates, which act on these fundamental units much like classical logic gates operate on bits. Quantum gates manipulate qubits by altering their states, enabling computations. Common gates include the Hadamard gate, which puts a qubit into superposition, and the CNOT gate, which flips the state of a qubit depending on another's state.
Quantum circuits consist of sequences of such gates designed to perform specific computations. By arranging these gates in various configurations, quantum algorithms can be structured to solve problems efficiently. The design of these circuits is crucial; poorly defined circuits not only diminish performance but can also introduce errors, highlighting the complexity involved in quantum computing.
"The real challenge is not just creating qubits, but developing reliable quantum gates and circuits that can harness their full potential."
Quantum Algorithms
At the heart of quantum computing's capabilities lies quantum algorithms. These specially crafted sets of instructions exploit quantum principles to solve problems more efficiently than classical algorithms. Well-known examples include Shor's algorithm, which factors large numbers exponentially faster than classical methods, and Grover's algorithm, which provides a quadratic speedup for unstructured search problems.
Quantum algorithms capitalize on superposition and entanglement to perform operations at unprecedented speeds. They are designed around the capabilities and peculiarities of qubits rather than adhering to the traditional paradigms of classical computing. As researchers continue to develop and refine these algorithms, the potential applications in various fields are staggering—from cryptography to optimization in logistics.
Understanding these core components—qubits, quantum gates, and quantum algorithms—not only enriches our knowledge but illuminates the future possibilities that quantum computing can manifest. The interaction among these elements defines the unique nature of quantum computers, setting the stage for advancements that could reshape numerous industries and societal frameworks.
Comparison Between Quantum and Classical Computing
The analysis of quantum computing in relation to classical computing is fundamental for several reasons. Understanding how these two paradigms differ enables us to appreciate the vast potential of quantum systems in addressing complex challenges that current technology struggles with. The contrast between these computing models sheds light on the inherent advantages and limitations of quantum computing, and it reveals the kinds of problems where quantum solutions could outshine classical ones.
Fundamental Differences
At the heart of distinguishing quantum from classical computing is the concept of information representation. Classical computers operate using bits as the smallest unit of data, which can exist in one of two states: 0 or 1. This framework is straightforward but limits the range of computations that can be performed simultaneously. On the other hand, quantum computers use qubits, which capitalize on the principles of superposition and entanglement. Thanks to these properties, a qubit can exist in multiple states at once.
Here's a straightforward breakdown of key differences:
- Bit vs. Qubit: Classical bits are binary, while qubits can exist in multiple states simultaneously, allowing for parallel processing.
- Deterministic vs. Probabilistic: Classical computing follows a predictable path, executing one instruction at a time. Quantum computing leverages probabilities, letting certain outcomes be part of a computational process until measured.
- Information Manipulation: Quantum computers have the ability to manipulate data in ways classical machines cannot, such as performing complex calculations involving numerous variables at the same time.
These differences present unique advantages for quantum computing, suggesting that it can solve certain types of problems more efficiently than classical systems, especially as problems scale in complexity.
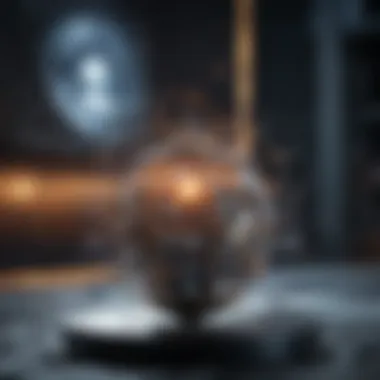
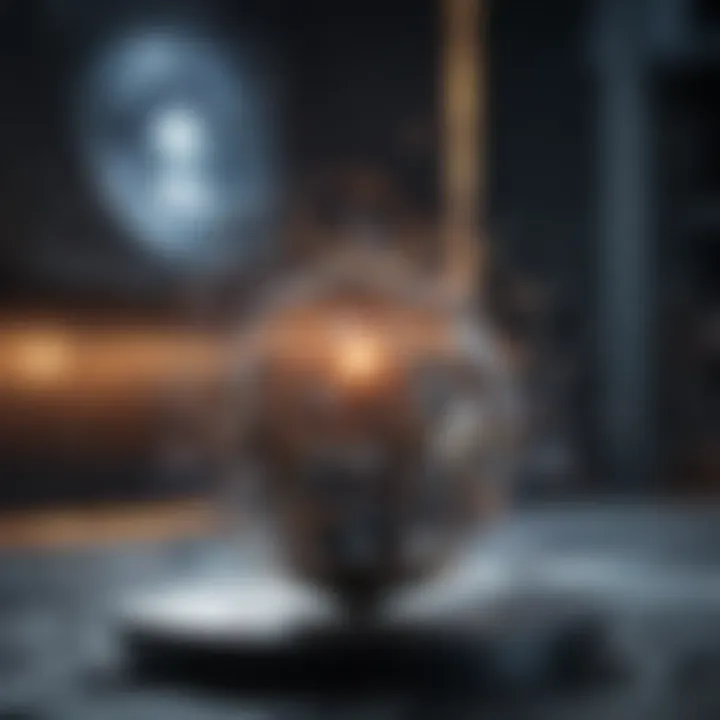
Performance Metrics: Speed and Capability
When discussing performance between quantum and classical computing, it’s crucial to note the metrics used for evaluation. Classical computers get continually faster and more complex, yet they face significant constraints in terms of operational speed when confronted with certain computational tasks.
Quantum computers, while still in their nascent stages, promise astonishing speed improvements in specific areas. For example, they can theoretically perform complex simulations in seconds, tasks that might take classical systems hundreds of years. This potential leads to fascinating implications in fields like cryptography and material science.
Some key performance metrics to consider include:
- Speed of Computation: Quantum computations can process an exponential amount of data compared to classical computations, due primarily to the principle of superposition.
- Scalability: As more qubits become entangled in quantum systems, computational capacity increases in ways that classical computers simply can’t match.
- Problem-Solving Capability: Quantum algorithms, like Shor's algorithm for factoring large numbers, demonstrate how quantum computers can outperform classical computers in certain tasks, highlighting their specialized performance.
While quantum computing is not universally superior, its strength lies in specific fields where classical systems may falter.
"The ability to harness the principles of quantum mechanics fundamentally alters the landscape of problem solving in computing, expanding both speed and capability in unprecedented ways."
In the grand scheme of computing technologies, comparing quantum and classical computing not only reveals the strengths and weaknesses of each but also the transformative potential of quantum computing in the years to come. As we proceed in this investigation, the stark contrasts and pronounced advantages of quantum systems become ever clearer.
Applications of Quantum Computing
Quantum computing stands not only as a glimpse into the future but also as a practical solution to some nagging problems faced by various fields today. Its applications are poised to revolutionize industries ranging from data security to pharmaceuticals, offering capabilities that classical computers can only dream about. As the world is flooded with more data than ever before, the significance of quantum computing can’t be overstated. Let’s unpack this further.
Cryptography and Data Security
In the ever-evolving landscape of digital communication, cryptography serves as a backbone for protecting sensitive information. Classical encryption methods, while competent, are rapidly being outpaced by the capabilities of quantum computers. For instance, Shor’s algorithm can factor large integers exponentially faster than the most advanced classical algorithms. This poses a significant threat to current encryption standards like RSA, which relies on the difficulty of factoring large numbers for security.
Taking a step back, the larger picture reveals a need to rethink encryption altogether. Quantum cryptography, particularly quantum key distribution (QKD), offers a solution, allowing two parties to generate a shared, secret key, with the authenticity guaranteed by the laws of quantum physics. As such, even if a hacker attempts to intercept the communication, any disruption introduced would be immediately detectable, ensuring a new level of data security. Overall, this application hints at physical laws doing the heavy lifting in safeguarding our information.
Complex Problem Solving in Science and Engineering
Then there’s the realm of problem-solving in science and engineering, where quantum computing is like giving a child a box of crayons in a black-and-white world. Take, for example, the optimization of complex systems like traffic flow or logistic chains. Classical algorithms struggle with large datasets, often leading to computational bottlenecks and inefficiencies. Quantum computers, however, can evaluate countless possibilities simultaneously, dramatically reducing the time it takes to find the optimal solution.
Some specific areas that stand to gain tremendously include:
- Materials Science: Designing new materials with specific properties often involves complex simulations. Quantum computing can speed up material discovery and molecular modeling, opening doors to innovative solutions for industries from technology to renewable energy.
- Engineering Challenges: From aerospace to civil engineering, quantum models could simulate physical systems in real-time, making project planning and execution much more efficient.
These advancements could fundamentally reshape how solutions are approached in engineering and science, paving the way for faster, more effective methodologies.
Pharmaceutical and Material Science Innovations
The potential of quantum computing isn’t just a far-off concept; it’s already transforming pharmaceuticals and material sciences in extraordinary ways. Given the deeply intricate nature of molecular interactions, devising new drugs remains a time-consuming and costly process. Classical computers model these interactions with considerable difficulty, often needing approximations that may overlook subtleties.
Here’s where quantum computing strides in like a superhero. By accurately simulating molecules and their interactions at a quantum level, researchers can predict how drugs will behave in the body with a higher degree of accuracy. Essentially, this can expedite drug discovery, ultimately resulting in faster development of essential medications.
Moreover, in materials science, quantum algorithms can identify and optimize the properties of new materials. These materials can possess unique characteristics, leading to breakthroughs in everything from energy storage to superconductivity, aligning perfectly with the quest for sustainable solutions.
Quantum computing has the potential to enhance the pace of innovation, thus redefining industries and addressing urgent societal needs.
In summation, applications in cryptography, complex problem-solving, and pharmaceuticals illustrate how quantum computing can be a game changer. Its ability to perform calculations beyond classical computers creates solutions to fits various industries, ultimately steering us toward a more advanced future. Quantum computing not only holds promise but also provides a multifaceted toolkit for tackling some of the most pressing challenges we face today.
Challenges and Limitations
Understanding the challenges and limitations of quantum computing is crucial. This subject underscores the complex nature of quantum technologies and sheds light on the various issues that researchers and developers face. The rapid strides made in quantum computing bring both excitement and obstacles, revealing how far we have come and what still lies ahead.
Technical Hurdles in Quantum Hardware
When we talk about technical hurdles in quantum hardware, it’s clear that building a functional quantum computer isn't just about throwing together some qubits. Many factors come into play that complicate this seemingly simple task.
One of the major issues is decoherence. In plain terms, decoherence happens when a quantum system loses its quantum properties due to interaction with the environment. Think of it as trying to maintain a delicate balance while juggling flaming torches amidst a gusty wind. This instability can lead to errors in computations, meaning that maintaining the delicate quantum state is a challenge that scientists are grappling with.
Another issue is error correction. Unlike classical bits that are clearly either 0 or 1, qubits can exist in superpositions. Sounds cool, right? But when it comes to correcting errors, it gets tricky. There’s a significant overhead in quantum error correction that requires extra qubits and complex logic operations, which not only complicates the hardware but also makes it less efficient.
Here are a few key points to consider:
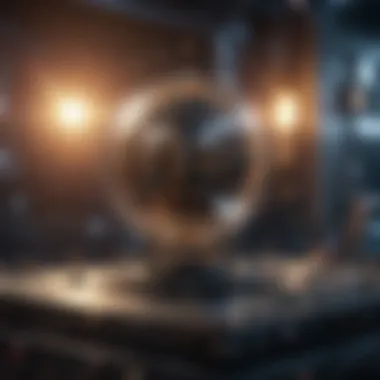
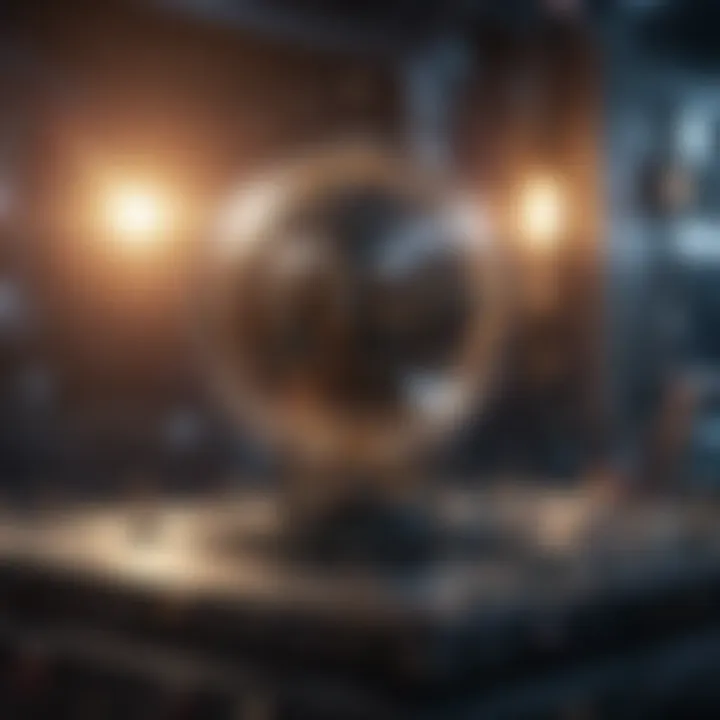
- Decoherence and noise: Quantum systems must be isolated from their surroundings as much as possible.
- Error correction methods: Developing effective methods is pivotal for reliable quantum computing.
- Temperature control: Many quantum computers require extremely low temperatures to function effectively, adding complexity to their construction.
The Question of Scalability
Scalability remains a central question in the advancement of quantum computing. Even if we manage to build a reliable quantum computer, can we just keep stacking qubits like Legos?
The answer isn’t straightforward. Scaling up quantum systems presents unique challenges. We can't just scale quantity without considering quality. Each additional qubit magnifies the potential for operational errors, increasing the chances of decoherence. Furthermore, as we add more qubits, the complexity of interconnections grows significantly. Often, achieving a higher number of qubits means nonlinear scaling of control systems, which can quickly become impractical.
The factors at play include:
- Integration Complexity: Managing larger systems with more qubits requires sophisticated control schemes, which may not exist yet.
- Crosstalk and interference: The interaction between qubits can lead to unwanted errors, complicating overall operations.
- Resource Availability: The demand for specialized materials and technology can restrict growth potential.
Ethical Considerations
As we navigate the challenges of quantum computing, we must also take a good hard look at the ethical implications. This technology holds significant potential but could also lead to new dilemmas.
First and foremost is the impact on security. Quantum computers, once fully realized, could break the encryption that keeps our personal and financial data safe. This raises questions about privacy and data protection. Imagine waking up to find that your bank account was compromised because someone's new quantum computer cracked your encryption overnight. Sounds like a plot twist from a cyber-thriller, right?
Moreover, as with many advanced technologies, there’s a risk of accessibility. Who gets to benefit from quantum computing? If only a handful of corporations or nations develop these systems, the digital divide may grow wider, leaving smaller players in the dust.
Here are some ethical considerations worth pondering:
- Impact on cybersecurity: Preparing for a quantum future necessitates new strategies for data protection.
- Equity in tech access: Ensuring that advancements benefit society as a whole is vital.
- Job displacement: Quantum computing could disrupt certain industries, making it necessary to consider retraining programs for affected workers.
In summary, the journey toward effective quantum computing is fraught with hurdles. While the allure of speed and capability is tempting, we must tread carefully and consider the iceberg lurking beneath the surface of progress.
As we move forward, the conversation on these topics will continue to evolve. The questions raised in this section are not simply academic; they represent real concerns that affect everyone— researchers, practitioners, and everyday users alike.
The Future of Quantum Computing
The future of quantum computing is both exciting and complex, intertwining hope with a fair share of skepticism. As this technology advances, the implications stretch across various fields—from computing efficiency to revolutionizing existing industries. Looking ahead, understanding what quantum computing means for the broader landscape of technology is crucial.
Many experts agree that achieving practical quantum computing could address problems classical computers struggle with. Fields like material sciences, pharmaceuticals, and even cryptography stand to gain immensely from these advances, breaking ground in areas that were previously thought impossible.
Predictions for Quantum Advancements
In the realm of quantum advancements, predictions often wade into speculative waters. Still, several key trajectories appear to be gaining consensus among researchers.
- Increased Qubit Stability: Future models may see qubits that are less susceptible to decoherence, allowing for longer operational times and more complex computations.
- Error-Correcting Techniques: With the enhancement of quantum error-correction protocols, it would be feasible to run larger algorithms more accurately.
- Hybrid Quantum-Classical Algorithms: Such algorithms might emerge, merging the robustness of classical computing with the unique strengths of quantum processing.
- Accessibly Powerfull Quantum Machines: As the technology matures, we may witness a shift from large-scale, specialized machines to more accessible and user-friendly quantum units.
Predicting the timeline of these advancements is tricky, of course. Many propose timelines spanning anywhere from five to fifty years, emphasizing that breakthroughs will come in fits and starts rather than a straight line.
Quantum Computing and Artificial Intelligence
The intersection of quantum computing and artificial intelligence (AI) could redefine capabilities in ways we can only begin to imagine. Quantum computers are not just faster; they can handle vast datasets far more efficiently than classical computers.
- Enhanced Machine Learning: Quantum algorithms designed for machine learning could lead to faster training times and more precise predictions.
- Data Processing: The ability to process multifaceted datasets in mere moments can make a significant difference in real-time applications, such as autonomous vehicles or intelligent systems operating in dynamic environments.
- Optimization Problems: Tasks that involve finding the most efficient route, resource allocation, or scheduling could benefit from combinatorial optimizations that quantum computers excel at.
"AI powered by quantum systems may not just be a new generation but could leapfrog current capabilities, transforming industries beyond recognition."
End
In a world increasingly driven by technology, understanding quantum computing stands paramount. This domain not only offers solutions to problems that classical computers grapple with but also heralds a transformation across diverse sectors, ushering in a new era. From healthcare to artificial intelligence, the ramifications of quantum computing extend beyond mere technical capabilities; they redefine how we approach problem-solving and data processing.
The Impact on Society and Future Professions
The implications of quantum computing for society are profound. The shift from classical to quantum represents not merely an upgrade but a fundamental rethinking of computing. As we delve into specific impacts, a few areas emerge as particularly noteworthy:
- Healthcare Innovation: Quantum algorithms have the potential to optimize complex data sets, paving the way for advanced genomic analysis and personalized medicine. This could lead to faster drug discovery and better patient outcomes, thus transforming health services.
- Environmental Solutions: By tackling simulations at unprecedented speeds, quantum computing may offer breakthroughs in climate modeling. Enhanced predictions about weather patterns or assessing the impact of environmental policies are tangible benefits that can influence global efforts in sustainability.
- Job Creation and Evolution: As industries adapt to quantum technologies, new professions will arise. Roles focused on quantum algorithm development, hardware engineering, and even quantum ethics will demand skills that currently may not exist in educational curricula. This shift necessitates a reevaluation of educational pathways, urging academic institutions to prepare students for jobs that we are only beginning to imagine.
Delving deeper, the future professionals who engage with quantum computing will not only possess technical acumen but also a nuanced understanding of ethical considerations. They will need to address challenges posed by quantum capabilities in cryptography, for instance. As security paradigms shift, it is crucial that these future leaders think critically about the implications of their work.
"A problem well-stated is a problem half-solved." – Charles Kettering
This quote underscores the essence of quantum computing’s role in society. As researchers, educators, and industry leaders articulate problems in new ways, the solutions may evolve alongside them. The very nature of problem-solving will shift, influenced by the unique capacities of quantum systems.
In summary, the conclusion of this exploration into quantum computing reaffirms its significance not only in technological domains but also in societal context. As we look ahead, the interplay between quantum advancements and societal needs will shape our future, and recognizing this relationship is crucial for harnessing the revolutionary power of this computing paradigm.