Exploring Earth-Like Planets: Life Beyond Our World
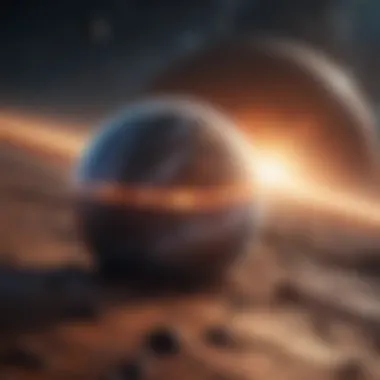
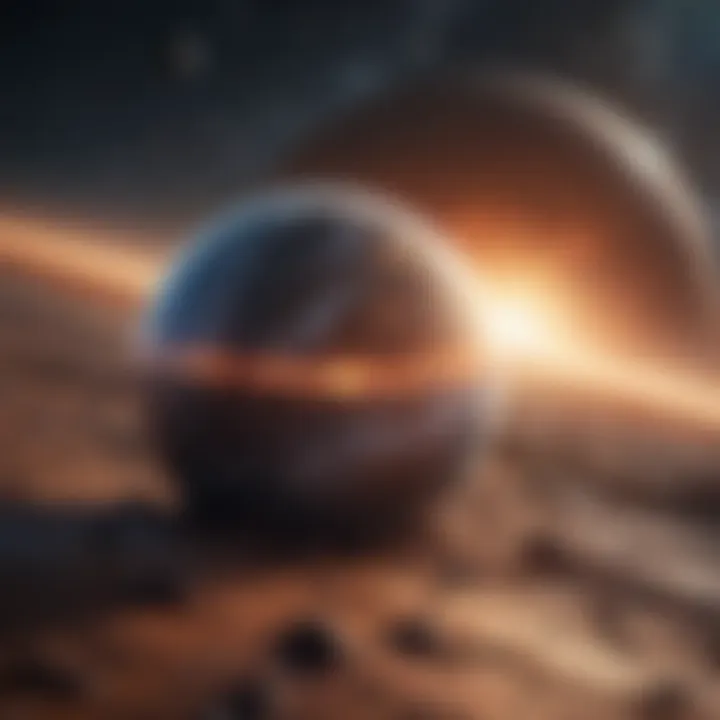
Intro
The prospect of discovering planets that bear a resemblance to Earth has captivated scientists, researchers, and the curious minds of humanity for decades. The universe, vast and largely unexplored, holds secrets that could shift our understanding of life itself. As we look beyond our familiar blue planet, we find ourselves asking: what makes a planet Earth-like? More importantly, could these distant worlds support life?
To venture into these questions requires an exploration of both the characteristics that define these planets and the significance they hold in astrobiology and our understanding of the cosmos. From recent discoveries that awaken our imaginations to the complex criteria used to classify these surfaces as potential havens for life, our journey offers insights into the incredible advancements in technology that make this pursuit possible.
In this section, we will unpack key research findings, shedding light on recent discoveries and their meanings to the field. We'll touch on the simplifying of intricate scientific concepts so that even those less familiar can appreciate the wonder locked away among the stars. Let's step forth into the unknown and draw connections bound not just to terrestrial life, but to a broader universe full of potential.
Key Research Findings
Overview of Recent Discoveries
In recent years, the quest to identify Earth-like planets has gained momentum, courtesy of powerful telescopes and advanced observational techniques. For instance, the Kepler Space Telescope has transformed our understanding of planetary systems by locating thousands of exoplanets, some of which share similarities with Earth in terms of size and distance from their host stars. Notably, planets like Kepler-186f and Proxima Centauri b have sparked intense interest, both being located within their respective star's habitable zones.
“The findings not only reflect the diversity of planetary bodies but also hint at the possibility of life outside our own world.”
Significance of Findings in the Field
These discoveries have not only widened the scope of astronomical study but also deepened the implications for life beyond Earth. The existence of so many Earth-like candidates raises crucial questions: how might life have developed on these planets? What conditions are necessary for sustenance beyond our atmosphere? The search for answers drives research in astrobiology, offering insights into life's resilience and its potential adaptive strategies in various environments.
Breakdown of Complex Concepts
Simplification of Advanced Theories
To delve deeper into the essence of what makes a planet truly Earth-like, it’s important to break down complex theories regarding atmospheric conditions, planetary formation, and the biochemical requirements for life. Many might find themselves turning away if only confronted by jargon. But, we can simplify it: the key aspects revolve around three main areas—
- Size and Composition: An Earth-like planet typically has a rocky surface and a comparable size to our own, allowing for similar gravitational conditions.
- Location in the Habitable Zone: This is the Goldilocks zone—not too hot and not too cold, where liquid water can exist, which is crucial for life.
- Atmospheric Conditions: The planet needs a stable atmosphere that can protect life from cosmic radiation and facilitate necessary chemical reactions.
Visual Aids and Infographics
To aid in understanding these concepts better, it is recommended to refer to various visual aids and infographics that detail the processes of planetary formation and the characteristics of discovered exoplanets. The use of tolls like the interactive models found on sites like Wikipedia and resources such as Britannica can greatly enhance one’s grasp of these vast ideas.
In summary, exploring Earth-like planets pushes the boundaries of human knowledge and curiosity, shedding light on realms and realities that have, until recently, seemed far-fetched. As we continue to uncover more about these worlds, our understanding of life's potential permanence in the universe expands alongside.
Defining Earth-Like Characteristics
Understanding the characteristics that delineate Earth-like planets serves as the foundation for our exploration of the universe. These planets hold the potential for sustaining life, much like our own. By examining the defining traits, we can ascertain which celestial bodies may be suitable for habitation. Each element, from physical size to atmospheric composition, intertwines into a web of requirements critical for life as we know it.
Physical Attributes
Size and Mass
Size and mass are crucial determinants in defining an Earth-like planet. Generally, a planet needs to be similar in size and mass to Earth to maintain conditions suitable for life. A key characteristic of suitable size is that it allows the planet to hold a sufficient gravitational pull. This gravity enables it to retain an atmosphere and, thus, maintain surface pressure conducive for liquid water.
The distinct feature of mass is particularly interesting. If a planet is too massive, gravitational forces might lead to an excessively thick atmosphere, possibly resembling those found on gas giants. Conversely, a planet too small may lead to insufficient gravity, causing it to lose its atmosphere and making the development of life as we know it nearly impossible.
Distance from the Star
The distance from its parent star is another essential criterion for planetary classification. This position defines the temperature ranges on the planet's surface—especially whether it sits within the so-called Goldilocks zone, where conditions can support liquid water. A planet too close to its star may experience excessive heat, turning its surface into a veritable hellscape, while one located too far would be perpetually frozen, creating a frozen lifeless world.
The unique aspect here is that slight shifts in distance can markedly impact the potential for life, broadening the scope of what we consider when searching for Earth analogs. A beneficial choice in discussing this topic lies in emphasizing the delicate balance required for life-supporting conditions.
Surface Conditions
Surface conditions encompass a myriad of factors including temperature, atmospheric pressure, and geology, which together foster environments that can either enhance or inhibit life. A critical characteristic here is that a stable surface able to promote liquid water is vital. Without a reliable and stable surface temperature, life would struggle to gain a foothold.
The distinctive feature of surface conditions is that they can vary widely from one planet to another, creating environments potentially hostile to life. Mentioning these variations enriches the discussion, as it provides insight into potential adaptations organisms might exhibit in different surroundings.
Atmospheric Components
Essential Gases
Essential gases comprise the building blocks of an atmosphere that can support life. Among these gases, oxygen and carbon dioxide are particularly noteworthy. They not only facilitate the processes of respiration and photosynthesis but also contribute to greenhouse effects necessary for temperature regulation. A critical point here is that while oxygen is often lauded as essential for life, it requires a balance with other gases to prevent conditions like oxidative stress.
What stands out about essential gases is how they establish chemical balances that govern surface conditions. This makes it a beneficial focal point for understanding the viability of a planet for life.
Pressure and Density
Pressure and density are significant when evaluating a planet’s atmosphere. Sufficient atmospheric pressure is crucial for retaining liquid water on the surface. A key characteristic of planetary atmospheres is that they often exert a pressure that can range widely, influencing the boiling and freezing rates of water.
The unique feature of pressure is that it dramatically influences climate and weather patterns. A planet too low in density might result in atmospheric escape, while a dense one could lead to extreme weather conditions, both posing challenges for sustaining life.
Climate and Weather Patterns
Climate and weather patterns shape the environmental conditions on any given planet. Variations in these patterns influence the water cycle and nutrient distribution, which are essential for life. The key characteristic of these systems is that they are intrinsically linked—any shift in weather contributes to long-term climate changes.
The distinctive nature of climate is its interplay with biological systems. This connection serves as a potent reminder of how fragile life can be, relying on a delicate balance of conditions.
Presence of Water
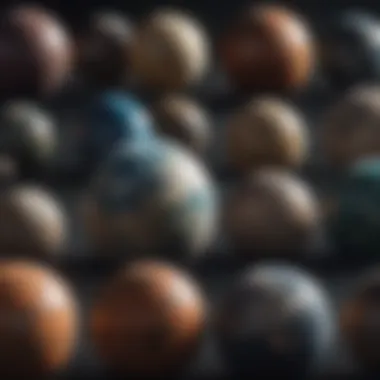
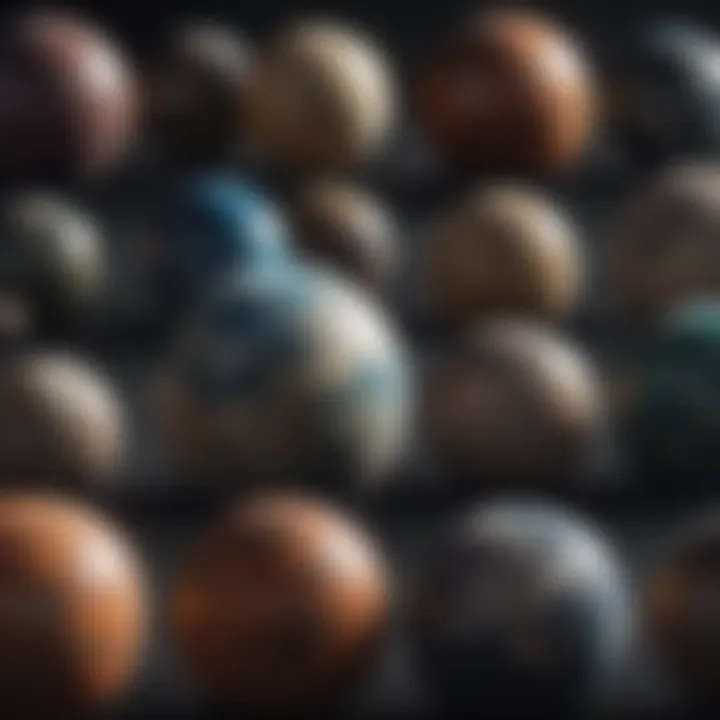
Surface Water Bodies
Surface water bodies are imperative for life, offering habitats where diverse ecosystems can flourish. The presence of oceans, rivers, and lakes facilitates nutrient cycling and sets the stage for biological activities. A notable aspect of these hydrological features is that they are often correlated with complex climates, making them vital to planetary ecosystems.
Shining a light on surface water bodies helps in illustrating why they are considered beneficial indicators for habitability. They are among the first characteristics scientists look for in the search for Earth-like planets.
Water Cycle Dynamics
Water cycle dynamics refer to the continuous movement of water within and between the atmosphere and surface of the planet. This cycle is fundamental for maintaining ecological balance and supporting life. The uniqueness of water cycles lies in their ability to regulate not just ecosystems, but also the climate itself.
Discussing the water cycle's intricacies allows for a greater understanding of how planets can sustain life, pointing out why researchers prioritize this feature when identifying Earth-like candidates.
Implications for Life
Finally, the implications for life itself underline the significance of examining planets that can support it. Understanding the interplay of factors such as size, atmosphere, and water can reveal the possible emergence of life forms.
The interesting element here is that Earth-like planets present a spectrum of opportunities, aiding the exploration of how various environments influence biological evolution. It becomes evident that focusing on these implications adds depth to our quest for understanding life beyond Earth.
Criteria for Earth-Like Classification
In the quest to identify planets similar to our own, defining the criteria for Earth-like classification becomes pivotal. This classification is not merely an academic exercise; it influences how we target our search for life beyond Earth. If we're looking for habitable worlds, we need to establish what conditions facilitate life as we know it. Key elements include locations in the star's habitable zone, a stable geological environment, and protective magnetic fields. These factors do not exist in isolation; they weave a complex web of interdependencies.
The Goldilocks Zone
The Goldilocks zone, often referred to as the habitable zone, is a region around a star where conditions are just right for liquid water to exist on a planet's surface. This concept plays a crucial role in our search for Earth-like planets.
Orbital Mechanics
Orbital mechanics dictates how a planet travels through space around its star, polishing off the requirements for it to be in the right spot. The key characteristic here is the equilibrium of forces acting on a planet, which influences its distance from the star. A stable orbit ensures that the planet doesn't veer too close, leading to extreme heat, or too far, where it becomes frozen.
The unique feature of orbital mechanics is its ability to demonstrate how planets can maintain suitable distances through various formations. However, differentiating between rocks in space or planets that might fall out of the habitable zone poses an ongoing challenge for astronomers.
Temperature Ranges
Temperature ranges within the Goldilocks zone detail how a planet can have liquid water and, by extension, potentially support life. The ideal range is typically between 0°C and 100°C. This specificity makes temperature a benchmark choice for assessing Earth-like characteristics because it holds significant implications for surface conditions.
Nevertheless, various external factors, such as a planet's atmosphere and its interaction with stellar activity, can skew these temperatures. Thus, while useful, these ranges must be contextualized rather than seen as a hard rule.
Habitable Conditions
Habitable conditions encapsulate the chemical and physical prerequisites for life to flourish. These include a stable atmosphere, geological stability, and, most importantly, the presence of liquid water. They are crucial to the overarching goal of identifying worlds that could bear life.
This condition has a unique feature; it not only defines potential habitats but also ties back to the biological ecosystems on Earth. However, the challenge lies in understanding that these conditions may vary vastly in different environments, complicating classifications.
Geological Stability
Geological stability is another essential criterion that governs the classification of Earth-like planets. This factor essentially refers to whether a planet can maintain its physical state over geological time frames.
Plate Tectonics
Plate tectonics is a hallmark of Earth's geological activity, driving the planet's climate regulation and recycling of carbon. Understanding this mechanism is beneficial because it informs researchers whether similar processes exist elsewhere.
Plate movement permits the formation of diverse geographic features, making it a significant aspect to highlight. Yet, the challenge is that not all planets have plates; some might maintain stability without tectonic activity, which leads to a potential gap in classification reliability.
Volcanism
Volcanism complements the discussion surrounding plate tectonics, as it allows for the continuous reshaping of a planet's surface. These processes help regulate atmospheric properties and temperatures which is crucial in sustaining potential life. The unique aspect here lies in the byproducts of volcanic activity, such as gases that can help form an atmosphere.
However, not every planet's volcanism indicates suitability for life; overactive volcanic worlds could signal instability instead of the reassuring presence of life-fostering conditions.
Seismic Activity
Seismic activity provides yet another lens to evaluate geological stability. A planet that experiences frequent quakes may indicate good reports of internal heat and energy flow, which can be indicators of active geophysical processes. This aspect is particularly important for understanding if a planet has a diverse ecosystem potential.
Nonetheless, extreme seismic events could lead to hazards detrimental to life, creating a complex relationship between activity levels and habitability.
Magnetic Field and Protection
Assessing a planet's magnetic field is integral, as it serves as a barrier against cosmic radiation. A protective magnetic field can signal a planet's potential to maintain an atmosphere conducive to life.
Role in Atmospheric Retention
The role of a magnetic field in atmospheric retention cannot be overstated. Essentially, this field helps to anchor the atmosphere and shields it from being stripped away by solar winds. Its significance ties back to maintaining essential gases like oxygen and nitrogen, crucial for life. This characteristic is beneficial because it directly links to planetary longevity and stability. However, measuring magnetic strength across different planets introduces complexity in understanding their atmospheres, as not all fields offer equal protection.
Protection from Cosmic Radiation
Protection from cosmic radiation is yet another aspect that reinforces planetary habitability. While a strong magnetic field can shield a planet, weak or absent fields leave celestial bodies vulnerable to high radiation levels, fundamentally affecting potential biological development. This factor is essential for astrobiological studies and underscores the factors shaping planetary atmospheres.
Nonetheless, varying levels of radiation could also spur mutations, leading to an interesting interplay in the developmental outcomes of life forms, should they arise.
Comparison with Earth
In comparing a new planet's magnetic field with Earth's, researchers can gauge its potential to support life. Earth’s magnetic field is robust, allowing it to retain its atmosphere effectively and protect against harmful solar radiation.
The unique feature in this comparison lies in the insights it offers about other planets. However, key factors such as distance from their respective stars also come into play, making it essential to assess multiple variables collaboratively.
The Search for Earth-Like Planets
Finding planets that resemble Earth is not just a matter of curiosity but a critical step in understanding our place in the cosmos. The quest for these Earth-like worlds raises important questions about our existence and the potential for life beyond our blue planet. Identifying habitable zones, where life as we know it could flourish, could lead to significant discoveries about our solar system and others beyond it. This section unpacks the historical advancements, cutting-edge technologies, and remarkable discoveries that illuminate the road ahead in finding Earth-like planets.
Historical Context
Early Theories
In the beginning, theories about planets and their potential to harbor life were mostly speculative. Early astronomers like Giordano Bruno and Johannes Kepler imagined that planets could harbor life. Their ideas sparked imaginations and set the stage for scientific inquiry. The core characteristic of these early theories lay in their philosophical approach; they were often derived more from deductive reasoning than observational evidence. This exploratory mindset has been a beneficial choice for this article, as it highlights the fundamental human inclination to look beyond immediate understanding.
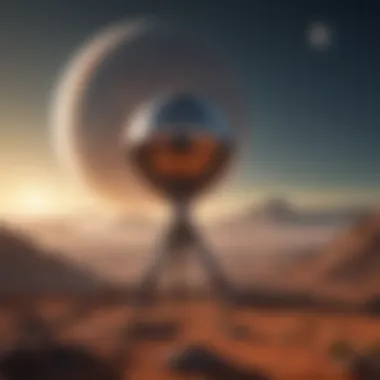
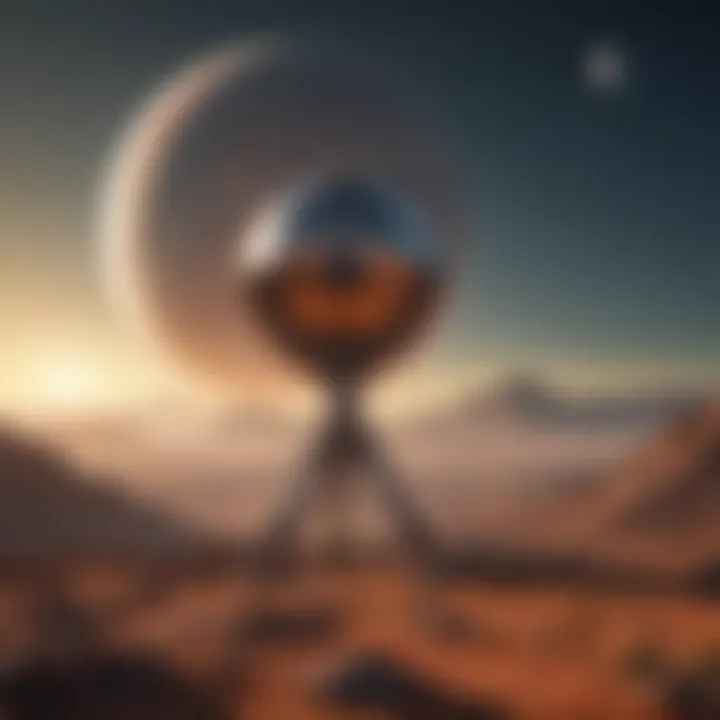
However, while inspiring, these theories had their limitations. The lack of evidence led to speculation that wasn’t always scientifically grounded.
Technological Innovations
As time passed, technological leaps broadened our understanding of the universe. Innovations in telescope design allowed astronomers to gaze deeper into the cosmos than ever before. Equipment like the Hubble Space Telescope transformed our capability for observation, revealing galaxies that were previously invisible, thus paving the way for serious scientific inquiry into exoplanets. The pivotal feature here is the resolution increase in imaging technologies alongside the launch of space-based observatories, making it a popular choice for discussing the search for Earth-like planets.
On the downside, such technology often requires massive investments and might not always yield immediate results. With new advancements come new challenges, like the need for persistent funding and maintenance of sophisticated machinery.
The Role of Telescopes
Telescopes serve as the eyes to the universe, drawing a direct link between the vast unknown and our understanding of planetary formations. The Critical characteristic of telescopes lies in their ability to gather light from distant stars and planets. This ability enhances our knowledge of celestial bodies, thus greatly assisting the search for Earth-like planets.
Each advancement brought about greater clarity on the atmospheric compositions of these worlds. However, despite the benefits, telescopes also face limits; they can struggle to differentiate between the light from a star and the faint glow from an orbiting planet.
Current Technologies
Transit Method
The Transit Method has revolutionized how we identify exoplanets. By observing the dimming of a star's light as a planet passes in front, astronomers can deduce the planet's size and orbit. It's an accessible and straightforward approach, making it an attractive choice for inclusion in this article.
However, its reliance on alignment between star and planet limits its effectiveness and may result in missed discoveries.
Radial Velocity Method
The Radial Velocity Method measures the gravitational effect a planet has on its parent star, resulting in subtle shifts in the star's spectrum. This characteristic has made it valuable for confirming the existence of many previously discovered planets. Its sensitivity and reliability render it a good candidate for this article, exposing the nuanced dynamics between stars and their orbiting planets.
Nonetheless, one disadvantage is its relative complexity, requiring precise instruments and advanced algorithms for accurate readouts.
Direct Imaging Techniques
Direct Imaging Techniques involve capturing the images of planets rather than relying on indirect measurements. This method opens the door to studying the atmospheres of distant worlds, a feature that is fundamental for astrobiological inquiries. Its innovative approach to planetary observation makes it an exciting component for discussion in this article.
On the flip side, the challenges of distinguishing a planet's light from its host star’s glare make it a painstaking process that can yield limited results.
Notable Discoveries
Kepler Mission Findings
The Kepler mission profoundly expanded our understanding of the universe. By continuously monitoring a fixed patch of sky, researchers identified thousands of exoplanets, many of which sit within the Goldilocks zone. This wealth of data has made the Kepler findings essential in any discussion regarding the search for Earth-like planets.
However, Kepler's indirect methods meant certain characteristics of the planets like their atmospheres remained unknown.
TESS Contributions
Transiting Exoplanet Survey Satellite (TESS) has built upon the foundations laid by Kepler, focusing on nearby stars. The key characteristic of TESS is its ability to survey large swathes of the sky quickly. This efficiency makes it a beneficial tool for quickly scanning potential candidates. Yet, the sheer volume of data generated necessitates rigorous analysis, which can introduce delays in confirming findings.
Emerging Exoplanets
The influx of discoveries dubbed emerging exoplanets often derive from innovative methods and analyses undertaken by research teams around the world. As these planets are being confirmed, they offer perspectives on the diversity of world types that could exist. Notably, many of these emerging exoplanets might be gas giants or icy worlds, which poses questions about their potential for supporting life. Their unique nature provides a fresh narrative for the ongoing discussion surrounding Earth-like planets, although their characteristics can often lead to speculation rather than concrete conclusions.
Implications for Astrobiology
The study of Earth-like planets goes hand in hand with the fascinating field of astrobiology, which seeks to understand the possibilities of life beyond our own planet. This complex interrelationship provides insight not only into what defines life but also into the conditions necessary for its existence. This section delves into the nuances of astrobiology, examining key characteristics and considerations surrounding the potential for extraterrestrial life.
Life as We Know It
Definitions of Life
To grasp the concept of life on Earth-like planets, we need to first establish what we mean by life. Definitions vary, but a common trait among them is the ability to grow, reproduce, and respond to stimuli. These core principles provide a solid framework for inquiries into astrobiology. Such definitions are useful since they offer a measurable criterion for identifying life elsewhere in the universe. However, one must consider that life might manifest in forms drastically different from our experiences. When exploring Earth-like conditions, sticking strictly to biochemical definitions could unnecessarily limit our understanding. Therefore, an umbrella term encompassing a variety of life forms might expand our scope of potential discoveries.
Biochemical Requirements
Another essential aspect of astrobiology is the biochemical requirements necessary for life to thrive. Most Earth-based life relies on water, carbon, nitrogen, and a handful of other vital elements. This reliance shapes our search for life on other planets. Since water is widely considered a necessity, planets that boast liquid bodies become prime candidates for exploration. Nevertheless, the fact that life uses specific biochemical processes doesn’t preclude the existence of alternative life forms that might thrive on different compounds. Thus, while focusing on familiar biochemical markers serves us well, it is worth remaining open to the possibility of novel chemistry sustaining life in off-world environments.
Evolutionary Scenarios
Understanding evolutionary scenarios offers insightful perspectives on how life could potentially emerge on Earth-like planets. Evolution, driven by environmental pressures and random mutations, leads to incredible biodiversity here on Earth. When theorizing about life on other planets, one key characteristic of evolutionary theory is the adaptability of organisms. The question arises: how might life evolve in radically different environments? Exploring these scenarios can lead to unique insights about resilience and adaptability, which might inform our understanding of both Earth-native and extraterrestrial organisms. Ultimately, acknowledging various evolutionary trajectories opens avenues for discovery, highlighting the complex interplay between biology and planetary environments.
Alternative Life Forms
Extreme Environments on Earth
Earth itself serves as a model for understanding potential life forms that exist in extreme environments. Organisms have been found thriving under high pressure, temperature extremes, and acidic or alkaline conditions. These extremophiles highlight that life can survive in places once deemed inhospitable. This characteristic is particularly compelling in the context of astrobiology. By investigating these organisms, researchers can postulate the possibility of life on planets with conditions vastly different from our own.
Implications for Astrobiology
Understanding extreme life on Earth lays the groundwork for astrobiological research. The adaptation strategies of extremophiles enable scientists to explore environments with conditions they may have previously dismissed. For instance, the possibility of life on a Mars-like planet could greatly benefit from the lessons learned through these hardy organisms. They challenge the very notion of habitability, reshaping our expectations and expanding the horizons of our search for life among the stars.
Potential Discovery of Novel Life Forms
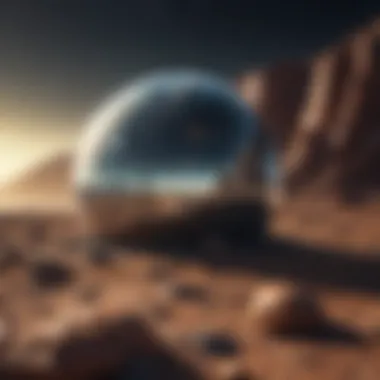
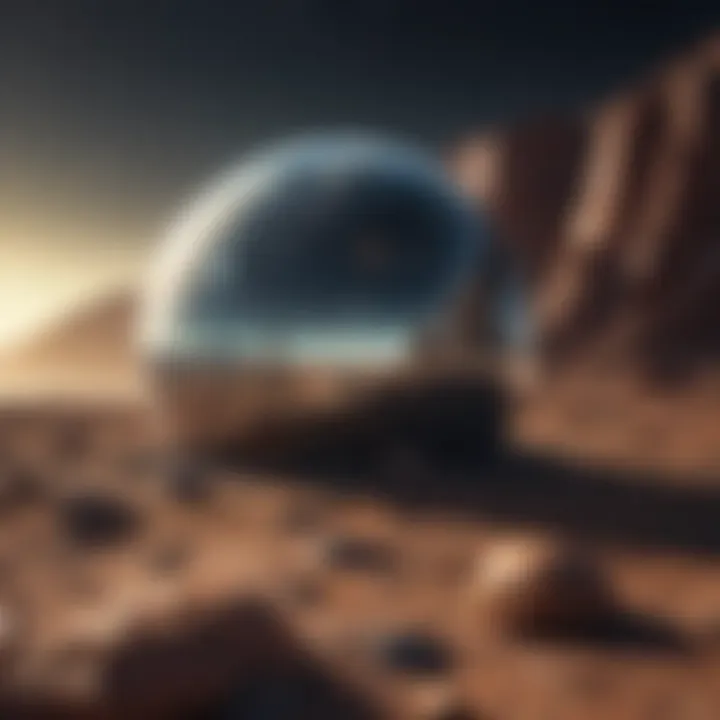
The study of extreme environments naturally leads to the exploration of potential novel life forms. If Earth’s extremophiles are any indication, life might emerge in forms we can hardly imagine. The idea that different biochemical pathways could give rise to living entities is both thrilling and humbling. These considerations pave the road for new methods of detection. Rather than exclusively hunting for conventional biosignatures, scientists are encouraged to broaden their search parameters, looking for signs that might suggest life forms operating under unfamiliar biological rules.
Societal and Ethical Considerations
Impact on Philosophy
The quest for Earth-like planets and potential life beyond our solar system has profound implications on philosophical discourse. As we delve deeper into astrobiology, questions concerning humanity’s place in the universe arise. What does it mean if life exists elsewhere? How does that shape our understanding of life’s value? These philosophical inquiries highlight the importance of integrating scientific exploration with ethical considerations. Such reflections can enrich societal dialogues, encouraging deeper contemplation on our collective future.
Ethics in Space Exploration
Tied closely with philosophical implications is the ethical dimension of space exploration. As we stand on the brink of potential discovery, questions arise about the treatment of extraterrestrial life forms. How should humanity approach these encounters? Ethical frameworks need to evolve in tandem with technological advancements, ensuring that exploratory missions reflect respect and humility toward any forms of life we may encounter.
Potential First Contact Scenarios
Lastly, the prospect of first contact with alien intelligence warrants serious consideration. Imagining such scenarios forces us to confront uncomfortable truths about ourselves. How we respond to contact could reshape our society and our values. Even the most optimistic scenarios require careful planning and consideration. This imagined encounter could lead us towards greater collaboration and understanding across global communities. Engaging in these dialogues prepares us for a future brimming with possibility, whether that is through cooperation or confrontation.
As we explore the cosmos, we not only seek understanding of other worlds, but perhaps a deeper understanding of our own human existence.
Future Directions in Planetary Science
The exploration of Earth-like planets across the cosmos is not merely an academic fascination. It's a profound pursuit that challenges our understanding of life's potential beyond our blue planet. As technology advances and our capabilities expand, we stand on the brink of exciting developments that could reshape our perception of the universe. The future directions in planetary science have the power to yield insights not just about distant worlds but also about our own existence and evolutionary pathway.
Interstellar Missions
Proposed Missions
The proposed missions to explore neighboring star systems spotlight the ambition that fills the scientific community. Organizations like NASA and private enterprises such as SpaceX have ambitious goals to reach other stars, including the Alpha Centauri system. While ambitious, these missions aim to gather data on potentially habitable exoplanets. The key characteristic of these missions lies in their innovative propulsion systems, which are designed to shorten travel times to years instead of centuries. This is a significant advantage for the feasibility of human exploration in our lifetime.
Such missions generally face unique challenges including funding, international cooperation, and the technological hurdle of efficient propulsion. They could offer pivotal advantages in our understanding of celestial bodies akin to Earth and help determine their potential to support life.
Technological Challenges
Technological challenges loom large in the landscape of interstellar exploration. The vast distances involved necessitate advancements in spacecraft technology, propulsion mechanisms, and life-support systems. The key characteristic of these technological hurdles is their complexity; for instance, the energy required for a spacecraft to reach even the nearest star is mind-boggling. Overcoming these obstacles is crucial because it would significantly influence the feasibility of such missions.
On one hand, advancements in propulsion technologies, like light sails and nuclear thermal rockets, offer potential solutions. The disadvantage, however, lies in the uncertainty of development timelines and costs associated with these technologies. Striking a balance between risk and innovation is critical as we chart a course towards interstellar missions.
Objective and Goals
The objectives and goals of future missions to Earth-like planets transcend mere exploration. They aim to answer pressing questions about life in the universe and provide critical data on planetary atmospheres and climates. Understanding whether these planets can foster life is paramount, as it would shift our perspective on our place in the universe.
A unique feature of these objectives is their interdisciplinary nature. Teams of astrobiologists, geologists, and astronomers work together, reinforcing that the search for life is a collective effort. The challenges lie in aligning the diverse objectives of various disciplines, yet this diversity also serves as a strength, opening up various avenues for innovation and discovery.
Habitat Simulation on Earth
Testing Earth-like Conditions
In the face of exploring other worlds, testing Earth-like conditions here on our planet becomes essential. Simulating environments that mirror those expected on the surface of exoplanets allows scientists to study potential biological processes in a controlled setting. The key characteristic of these simulations is adaptability; they can mimic diverse climates and atmospheric compositions.
Such experiments offer critical insights, helping refine what we consider essential for life. However, they may be limited by the challenges of accurately replicating every aspect of another planet’s unique conditions. The balance of realism and controllability remains a focal point in these efforts.
Research Facilities
Research facilities dedicated to planetary simulation play a central role in advancing our understanding of Earth-like conditions. These facilities often incorporate advanced technologies, such as vacuum chambers and precision climate control systems. Their key characteristic is their versatility, enabling experiments that probe into atmospheric compositions, climate patterns, and even the presence of water.
Unique features may include the capability to measure and manipulate variables at micro-scale levels, providing researchers with detailed data. The downside can be high operational costs and the requirement for specialized personnel, which may limit accessibility for broader scientific inquiry.
Insights on Future Colonization
The insights gained from simulating Earth-like conditions point to the feasibility of colonization on other planets. Scientists can gather information about the resources needed and the challenges that might arise when establishing a human presence in extraterrestrial environments. The key characteristic here is the predictive nature of these studies—estimating what humans would require to thrive on another world.
One significant feature is the potential for identifying sustainable practices that could support life long-term. The downside could be that while simulations provide valuable data, they may not capture unforeseen variables that real extraterrestrial environments could present, thus remaining a challenge in predictive accuracy.
Collaboration in Scientific Research
Global Research Initiatives
Global research initiatives play an instrumental role in poolng together diverse knowledge and expertise for the common goal of exploring our universe. By encouraging collaboration across national and institutional boundaries, scientists can tackle complex challenges more effectively. The key characteristic of these initiatives is synergy; when countries and institutions collaborate, they create a melting pot of ideas and diverse methodologies.
Unique features of these collaborative efforts might include shared resources such as telescopes and satellite data, promoting efficiency. However, bureaucratic hurdles and differing priorities among countries can pose challenges that slow down progress.
Interdisciplinary Approaches
The interdisciplinary approach to planetary science integrates insights from various fields like biology, chemistry, and physics. This melding of expertise is a hallmark of contemporary research and serves as a driving force behind innovative ideas. The key characteristic of interdisciplinarity is its depth; it enables a more rounded understanding of the multifaceted issues surrounding planetary conditions.
Such strategies present the advantage of discovering previously unconsidered perspectives, enriching research. Yet, it can create discrepancies in methodologies and language that may hinder efficient collaboration. Balancing distinct fields is crucial to maximize these benefits.
Public Engagement and Education
Lastly, public engagement and education are vital components in fostering interest and understanding of planetary exploration. By communicating scientific advancements and their relevance to everyday lives, researchers can inspire the next generation of scientists and adventurers. The key characteristic is accessibility, making complex concepts understandable for a lay audience.
Unique initiatives might include outreach programs in schools and interactive exhibits in museums, which can demystify planetary science. However, the risk lies in making oversimplifications that could distort the complexity of scientific research, leaving misinformation rather than understanding. Tackling this challenge is essential to maintain the integrity and excitement of scientific exploration.
A comprehensive approach that considers technological innovation, greater collaboration, and effective public engagement is key to advancing in planetary science towards encountering and understanding Earth-like planets.
These future directions not only promise to enhance our knowledge of potentially habitable worlds but also ignite the human spirit of exploration that has fueled our progress throughout history.