Exploring the Expansion of the Universe: Insights and Impacts
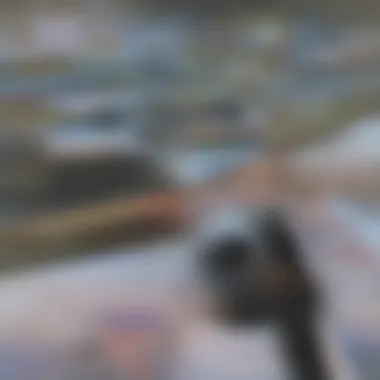
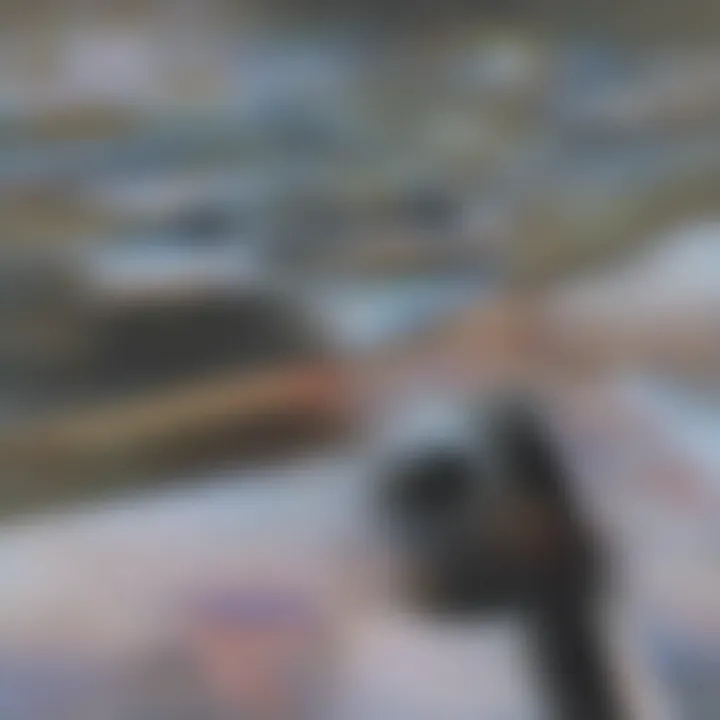
Intro
The expansion of the universe is a significant concept in cosmology. It describes how galaxies and other cosmic objects move away from each other over time. This phenomenon has profound implications for how we understand the cosmos. The theory of expansion arose from various observations and discussions among scientists over the past century.
Understanding this topic involves delving into historical progress, examining evidence, and exploring future implications. Also, we will discuss different models that provide insight into how this expansion functions. The aim is to enhance comprehension for students, researchers, educators, and professionals who seek clarity regarding this significant aspect of modern astrophysics.
Understanding the expansion of the universe helps us better grasp fundamental aspects of astrophysics and cosmology. This is not just an abstract theory; it is essential for explaining the overall structure and origin of the universe. In analyzing these ideas, we will outline key research findings that shape our current knowledge.
Prolusion to the Expansion of the Universe
Understanding the expansion of the universe is a cornerstone of modern cosmology. It is an essential concept that provides deep insights into the nature of our cosmos. The expansion process fundamentally alters our perception of space, time, and the overall structure of the universe.
Through this exploration, we find answers to many intriguing questions. How did the universe begin? What is its ultimate fate? These pivotal inquiries drive scientists to learn more about the dynamics of cosmic expansion.
Furthermore, studying this topic presents significant benefits. It enriches our knowledge about celestial phenomena and informs us about the conditions necessary for life as we know it. This understanding extends beyond theoretical discussions; it influences various scientific fields, including astrophysics and quantum mechanics.
In this section, we will first define what the expansion of the universe actually means, followed by an examination of its historical development. Understanding these elements assists researchers, educators, and students in comprehending the broader implications of cosmic expansion on our current scientific paradigms.
Defining the Expansion of the Universe
The expansion of the universe refers to the increase in distance between galaxies over time. This phenomenon was first observed in the early 20th century, leading to significant shifts in cosmological theories. The universe is not static; instead, it is continually evolving. In basic terms, as time passes, galaxies are moving away from each other. This observation suggests that the universe is not only expanding but that it has been doing so since its inception.
Mathematically, this concept can be expressed by the Hubble Law, which states that the recessional velocity of a galaxy is proportional to its distance from Earth. As galaxies recede, they appear redshifted due to the Doppler Effect, indicating that their light waves are stretched. This redshift serves as a critical piece of evidence for the expansion of the universe. This transformation in our understanding of cosmos invites further exploration into its implications and trends.
Historical Context
The historical context of the expansion of the universe represents a significant chapter in science. Prior to the 20th century, the prevailing model in cosmology was a static universe. Great thinkers like Isaac Newton and Albert Einstein largely supported the notion of an unchanging cosmos. However, the acceptance of an expanding universe began to surface as empirical observations became available.
In the 1920s, Edwin Hubble conducted extensive observations of various galaxies. His findings revealed that most galaxies are moving away from us. This discovery fundamentally challenged previous theories. Hubble's work supported the notion of an expanding universe, an idea that Albert Einstein himself later recognized and utilized in his theory of general relativity.
Moreover, this historical evolution has led to numerous cosmological models, each attempting to describe and predict the various behaviors of the universe. Today, the expansion of the universe continues to be a vibrant area of research, guiding scientific inquiry into the great unknowns of our time.
"The expansion of the universe shapes not only the cosmos but also our understanding of existence itself."
In summary, grasping the definition and historical context of the universe's expansion lays the foundation for deeper explorations into its various implications.
Key Theories in Cosmology
The exploration of the universe's expansion fundamentally alters our understanding of its structure and behavior. This section focuses on key theories that underpin modern cosmology. Understanding these theories allows us to grasp not only the origins of the universe but also the ongoing processes that shape its destiny.
The Big Bang Theory
The Big Bang Theory remains the dominant cosmological model explaining the early development of the universe. Its core premise asserts that the universe began as an extremely hot and dense point, approximately 13.8 billion years ago. From this singularity, the universe began to expand rapidly in an event termed the "Big Bang."
The significance of the Big Bang Theory lies in several aspects:
- Temperature and Density: In the moments after the Big Bang, the universe was a hot plasma, filled with particles and radiation. As it expanded, the universe cooled, allowing protons and neutrons to form atomic nuclei.
- Cosmic Microwave Background Radiation: An essential piece of evidence supporting this theory is the detection of the Cosmic Microwave Background (CMB) radiation. The CMB is a remnant of the early universe, providing a snapshot of its condition about 380,000 years post-Big Bang.
- Nucleosynthesis: The theory successfully explains the observed abundance of light elements, such as hydrogen, helium, and lithium, formed during the first few minutes of the universeβs existence.
The Big Bang Theory also invites questions about the nature of the universe's expansion. What drives this expansion? How does it relate to the ultimate fate of the cosmos?
Steady State Theory
In contrast to the Big Bang Theory, the Steady State Theory presents an alternative model emphasizing a universe that is eternal and unchanging in large scale structure. Introduced in the late 1940s by Fred Hoyle, Thomas Gold, and Hermann Bondi, this theory posits that, as the universe expands, matter is continuously created to maintain a constant overall density.
Key elements of this theory include:
- Continuity: As new matter is formed, old structures (like galaxies) move farther apart, yet the universe appears the same at any point in time. This idea counters the notion that there was a specific beginning.
- No Cosmic Background Radiation: The theory struggled to account for the CMB, which was seen as a critical supporting evidence for the Big Bang.
- Philosophical Implications: The Steady State Theory raises profound questions about the nature of time and existence. If the universe is eternal, what does that imply about its ultimate fate?
Supporting Evidence
The concept of the expansion of the universe is bolstered by a strong foundation of supporting evidence. This evidence not only lends credibility to the fundamental theories of cosmology but also enhances our understanding of the universe as a dynamic entity. Various observational data points provide insight into how the universe is not static but continuously evolving. Understanding these evidences is crucial for students, researchers, educators, and professionals in the field.
Notably, supporting evidence for cosmic expansion can be divided into several pivotal observational discoveries. These include the redshift phenomena observed in distant galaxies, as well as the relic radiation left from the very early universe. Both present noticeable implications for how we perceive the size, age, and eventual fate of the cosmos.
The presence of this evidence offers astronomers and cosmologists critical insights into the nature of cosmic expansion and its broader implications. It serves not just as foundational support for existing theories but creates avenues for further inquiry and understanding.
Hubble's Observations
Edwin Hubble's observations in the early 20th century fundamentally altered our understanding of the universe. By studying the light from distant galaxies, Hubble discovered that most of them are moving away from us, implying that the universe is expanding. This phenomenon, known as the redshift, occurs when the wavelength of light stretches due to the motion of the source. Hubble quantified this relationship through Hubble's Law, which states that the speed at which a galaxy recedes is proportional to its distance from us.
Hubble's discovery not only provided evidence of an expanding universe but also suggested a starting point, often referred to as the Big Bang.
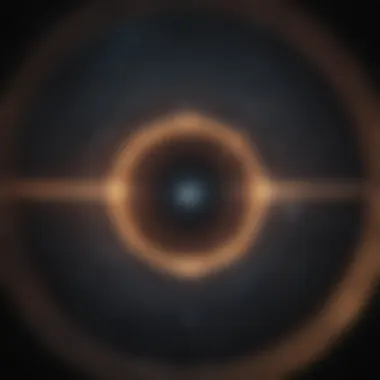
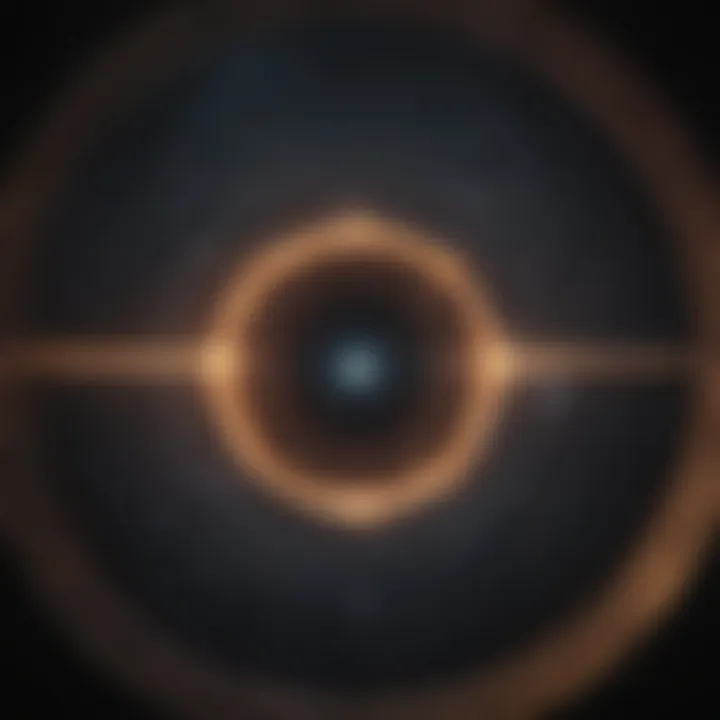
Hubble's observations laid the groundwork for the field of observational cosmology. They catalyzed the idea that galaxies are not isolated objects but part of a larger, evolving structure. His work has been fundamental in the quest to understand the universe's true nature and continues to influence modern cosmological research.
Cosmic Microwave Background Radiation
The Cosmic Microwave Background Radiation (CMB) is perhaps one of the most compelling pieces of evidence in support of the expansion of the universe. It is the afterglow of the hot, dense state of the universe just moments after the Big Bang. Discovered accidentally by Arno Penzias and Robert Wilson in 1965, this radiation fills the universe and is remarkably uniform, indicative of the early state of the cosmos.
CMB serves several important purposes in understanding cosmic expansion:
- It provides a snapshot of the universe approximately 380,000 years after the Big Bang, allowing scientists to glimpse its origins.
- The tiny fluctuations present in the CMB correspond to the seeds of structure that would eventually evolve into galaxies and clusters of galaxies.
- Its uniformity supports a model of a rapidly expanding universe.
The study of CMB has been pivotal in refining our models of cosmology, including how we view the universeβs structure and growth over time. As researchers analyze the CMB data further, they uncover implications about the universeβs composition, age, and fate.
In summary, both Hubbleβs observations and the CMB form a coherent narrative that supports the idea of an expanding universe, which is essential for deeper understanding in cosmology.
Mathematical Frameworks
Mathematical frameworks serve as the backbone for understanding the expansion of the universe. These frameworks provide precise tools to model complex cosmic phenomena, allowing scientists to interpret data and draw meaningful conclusions about the nature of the cosmos. Without the application of mathematics, the laws that govern the universe would remain abstract concepts, incapable of supporting empirical observations. Thus, it becomes evident that these frameworks are essential in advancing our comprehension of the universe and its vastness.
The significance of mathematical frameworks can be seen in several ways:
- They translate physical theories into quantifiable terms.
- They enable accurate predictions about cosmic behaviors.
- They enhance our understanding of the universe's structure and its dynamics.
By weaving mathematics with cosmological principles, researchers can formulate theories that explain the expansion, identify anomalies, and refine models. This intersection is where theory meets empirical observation, and it fosters the continuous exploration of the universe's mysteries.
Friedmann Equations
The Friedmann Equations present a set of equations derived from General Relativity that describe the expansion of the universe. These equations factor in the effects of matter and energy content of the universe, establishing a foundation for modern cosmological models. They represent how the scale factor of the universe changes over time. Specifically, the equations can indicate whether the universe is expanding, contracting, or remaining static.
- The first Friedmann equation relates the rate of expansion to the energy density of the universe.
- The second Friedmann equation provides insights into how pressure influences the dynamics of expansion.
These equations have profound implications for understanding how the universe evolves. They lay the groundwork for models that explain the present phase of cosmic acceleration, driven largely by dark energy and other components.
General Relativity and Cosmology
General Relativity, formulated by Albert Einstein, reshaped our understanding of gravity and its implications on cosmic scales. This theory posits that matter warps spacetime, and this curvature dictates how objects move within it. In the context of cosmology, General Relativity provides the principles needed to study the large-scale structure and dynamics of the universe.
By applying General Relativity, cosmologists can explore:
- The nature of cosmic expansion.
- The formation and evolution of structures within the universe.
- The relationship between energy and curvature of spacetime.
General Relativity is fundamentally linked with cosmological principles. It allows scientists to model conditions shortly after the Big Bang, providing a framework to describe the universe's journey through time. However, it also challenges our understanding, especially in regions of extreme density and curvature, such as black holes.
General Relativity and the Friedmann Equations together establish a framework that unifies theoretical predictions with observational data in cosmology.
Observable Implications
The observable implications of the expansion of the universe provide a crucial foundation for our understanding of cosmology. This section examines significant phenomena that arise from the expansion and how it shapes our perception of the cosmos. The expansion is not merely a theoretical notion; it has real consequences for galactic formations and the dynamics of the universe itself. By scrutinizing these implications, researchers gain insights into underlying physical processes and the conditions that govern the universe.
Redshift Phenomena
Redshift is a vital observable consequence of the universe's expansion. It refers to the increase in the wavelength of light emitted from distant galaxies. As these galaxies move away from us, the light they emit stretches, leading to longer wavelengths. This shift to the red end of the spectrum is observable through powerful telescopes. Edwin Hubble first identified this phenomenon in the 1920s, which became known as Hubble's Law.
- Importance of Redshift:
- It serves as the primary method for measuring the distances to far-off galaxies.
- It offers critical evidence for the expanding universe, confirming that galaxies are receding from us.
"The redshift not only connects distance and velocity but also illustrates the expanding nature of the universe."
Additionally, redshift plays a role in understanding the universe's acceleration. As more distant galaxies exhibit greater redshift values, it indicates that the rate of expansion is not constant. This observation shifts the focus to dark energy, a mysterious force believed to be driving this accelerated expansion.
Large Scale Structure of the Universe
The large scale structure of the universe pertains to the distribution of galaxies and galaxy clusters across cosmic distances. As the universe expands, the arrangement of these structures reveals critical aspects of its evolution and composition.
- Key Considerations:
- Cosmic Web: The universe appears as a vast cosmic web made of filaments and voids. These structures form due to gravitational interactions and the nature of dark matter.
- Galaxy Clusters: Studying the formation and distribution of galaxy clusters informs theories on the evolution of the universe.
It is crucial to recognize how the expansion influences the large scale structure. As space itself stretches, gravitational forces also act on matter, leading to the complex arrangement of galaxies we see today. Research in this area continues to be dynamic. Advances in observational astronomy help reveal more about how the cosmos is structured and how it will evolve further into the future.
Understanding the observable implications of expansion enriches our knowledge significantly. It is essential for students, researchers, and professionals in cosmology to grasp these concepts. This comprehension not only informs current theories but also paves the way for further discoveries in the ambit of astrophysics.
Cosmic Acceleration
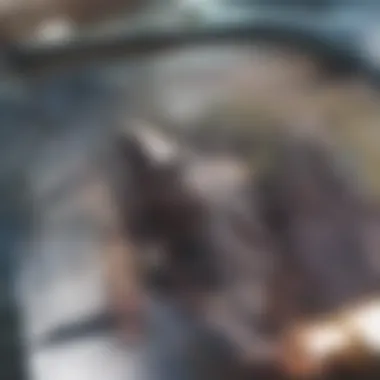
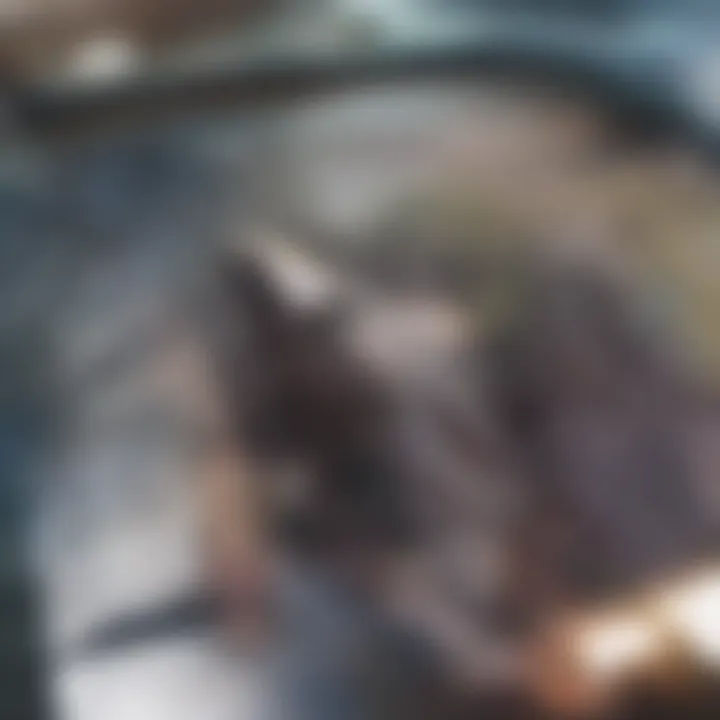
The phenomenon of cosmic acceleration is vital for understanding the current state and future direction of the universe. As observations indicate that the universe is expanding at an increasing rate, this raises intriguing questions about the underlying mechanisms that drive this acceleration. Such themes are essential in modern astrophysics, as they encourage a deeper investigation of the universe's makeup.
Dark Energy: An Overview
Dark energy is believed to be the primary force behind cosmic acceleration. It contributes approximately 68% of the total energy density of the universe. Despite its vast influence, dark energy remains one of the more elusive components of cosmology. This mysterious energy is thought to have a repulsive effect, exerting a negative pressure that causes galaxies to drift further apart.
Several theories attempt to explain the nature of dark energy. One of the most widely accepted is the cosmological constant, introduced by Albert Einstein. This constant maintains a uniform density throughout space, suggesting that even as the universe expands, dark energy continues to exert its force. Research continues to find new insights into its characteristics and why it dominates the energy composition of the universe.
Role of Dark Matter
While dark energy drives the cosmic acceleration, dark matter plays a critical role in the large-scale structure of the universe. Dark matter, which makes up about 27% of the universe's total mass-energy content, does not emit light or energy, making it invisible and detectable only through its gravitational effects.
The presence of dark matter influences how galaxies and clusters of galaxies form and evolve. Its gravitational pull helps bind large structures, counteracting the effects of cosmic acceleration on smaller scales. This interaction is crucial to maintaining the stability and arrangement of cosmic structures across time. Understanding dark matter is intertwined with unraveling the mystery of dark energy, as both components contribute fundamentally to the universe's behavior and layout.
"The study of cosmic acceleration requires an integration of dark energy and dark matter to fully comprehend the universe's expansion and structure."
In summary, cosmic acceleration represents a significant shift in our understanding of universal dynamics. Dark energy and dark matter interrelate intricately, shaping the cosmos we observe today. As research pushes the boundaries for answers about these elusive concepts, scientists hope to unveil the universe's ultimate fate.
Models of the Universe
Models of the universe are crucial in understanding our cosmological landscape. They provide frameworks that help scientists interpret observations and predict future behaviors of cosmic structures. At their core, these models aim to address fundamental questions about how the universe is shaped and how it evolves over time. By examining different models, researchers can gather insights into the universe's geometry, density, and eventual fate. In this section, we discuss two prominent types of models: open and closed models, as well as the flat universe perspective.
Open and Closed Models
Open and closed models present two contrasting views of the universe's overall shape and destiny. An open model suggests that the universe will continue to expand indefinitely. In this scenario, the density of the universe is lower than a critical value, leading to a geometrically hyperbolic space. Because of this, gravitational forces are not strong enough to halt expansion, allowing galaxies to drift apart over time.
On the other hand, a closed model implies that the universe has a finite volume and will eventually stop expanding and begin contracting. In this model, the density exceeds the critical level, creating a positively curved, spherical universe. Eventually, all matter in the universe will collapse into a dense state in a hypothetical event known as the Big Crunch.
Each model has significant implications. The choice between them shapes our understanding of cosmic evolution and the long-term behavior of galaxies and other cosmic structures. Additionally, the ongoing measurements of cosmic microwave background radiation and galaxy cluster distributions continue to inform which of these models align more closely with the real universe.
Flat Universe
The flat universe model occupies a unique position between open and closed scenarios. It suggests that the universe is geometrically flat and that its expansion will eventually stabilize. In this model, the density of the universe is precisely at the critical point, causing gravitational forces to balance with the outward momentum of cosmic expansion. Consequently, it neither collapses nor expands indefinitely.
This model has gained prominence due to observations made by various astronomical surveys, including the project by the Wilkinson Microwave Anisotropy Probe. The findings support claims that our universe is very close to flat, aligning with current understanding of dark energy and its role in the cosmos. A flat universe suggests that, over vast timescales, structures will evolve but remain relatively stable, providing a constant fabric for the galaxy formation processes.
Philosophical Implications
The expansion of the universe raises significant philosophical questions. It touches upon fundamental aspects of existence, reality, and the nature of knowledge itself. Understanding these implications is essential not only for scientists but also for anyone interested in the deeper meaning of cosmic phenomena. The exploration of these themes evokes a sense of wonder, inviting individuals to contemplate their place in the cosmos.
The Nature of Infinity
One key consideration is the nature of infinity. The concept of an infinite universe challenges traditional notions of space and time. When we discuss the universe expanding, we must consider what infinity truly means. Is the universe infinitely large? If so, what does that imply about our understanding of physics and the universe's limits? This idea creates a paradox where the infinite can seem unimaginable. It forces thinkers to confront their assumptions about existence.
Many theories revolve around this idea, such as the concept of multiple universes or multiverses. If our universe is just one of many, then what defines its uniqueness? The implications of infinity in cosmological discussions raise essential questions about reality and existence itself. Some posit that infinity may not be an absolute quantity but rather a conceptual tool to aid in understanding.
"Infinity is not just a number. It is a concept that reshapes our understanding of the universe."
Human Understanding of the Cosmos
Human understanding of the cosmos is also intricately linked to philosophical implications. The expansion hints at our quest for knowledge. As we learn more about the cosmos, we are simultaneously questioning our own significance. Are we mere specks in a vast expanse, or does our existence hold more meaning?
Throughout history, humanity has attempted to understand our place in the universe. The advancements in cosmology have encouraged us to reconsider philosophical foundations. This relationship between science and philosophy becomes apparent when exploring concepts such as the anthropic principle, which suggests that the universe exhibits physical properties that allow for the existence of life. This principle invites discussions about purpose and the nature of our reality.
Additionally, the way we interpret the findings of cosmology can deepen our understanding of ethical considerations. As we expand our cosmic knowledge, we should think about how that knowledge impacts our behavior and decisions, not just on Earth but in the broader universe. Our responsibility may extend beyond our planet to a universal perspective.
Current Research Directions
Research in the field of cosmology is continuously evolving, especially regarding the expansion of the universe. Understanding current research directions is crucial for grasping how scientists aim to expand our knowledge of cosmic processes. This section delves into two primary facets of ongoing research: observational astronomy advances and theoretical physics innovations. Each of these elements contributes significantly to our overarching comprehension of the universe and its complex dynamics.
Observational Astronomy Advances
Observational astronomy plays a pivotal role in studying the expansion of the universe. As technology advances, so does our capability to observe distant galaxies and cosmic phenomena.
Some recent developments include:
- Next-Generation Telescopes: Instruments like the James Webb Space Telescope are providing unprecedented views of cosmic structures. They allow astronomers to gather vast amounts of data on distant galaxies, helping determine their motions and distances accurately.
- Gravitational Wave Detection: Facilities such as LIGO (Laser Interferometer Gravitational-Wave Observatory) have opened a new avenue for understanding cosmic events. Gravitational waves provide insights into mergers of black holes and neutron stars, which can affect cosmic expansion metrics.
- Wide-Field Surveys: Projects like the Sloan Digital Sky Survey (SDSS) help create comprehensive maps of the universe. These maps reveal how galaxies are distributed, their movements, and how they interact. Such extensive data is essential for testing theoretical models against observational reality.
These advancements allow for a more precise measurement of the Hubble constant and other key parameters that define the universeβs expansion rate. They enhance our ability to make predictions about cosmic evolution.
Theoretical Physics Innovations
Alongside observational improvements, theoretical physics continues to push the boundaries of our understanding regarding cosmic expansion. Research in this area often intersects with ongoing debates about fundamental cosmological concepts.
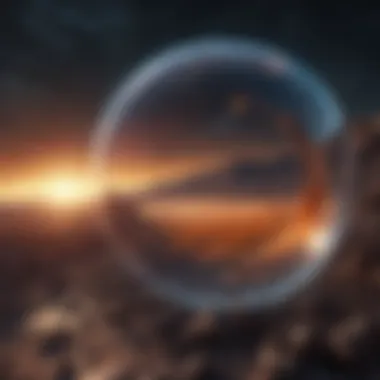
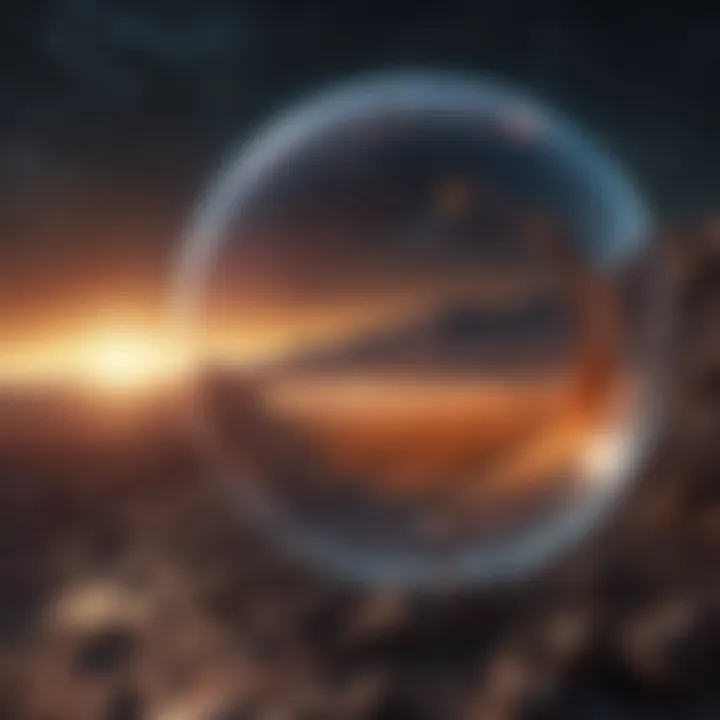
Key areas of innovation include:
- Dark Energy Models: As dark energy plays a significant role in the universe's expansion, researchers are developing various models to understand its properties and implications. Studies focus on how dark energy influences the acceleration of cosmic expansion.
- Modified Gravity Theories: Several cosmologists are exploring modifications to Einstein's general relativity. These models aim to account for observed cosmic acceleration without resorting to dark energy, proposing alternatives that might better explain current observations.
- Quantum Gravity: The integration of quantum mechanics with gravity remains an open problem. New theories in this domain could lead to revolutionary insights about the fundamental mechanics of the universe and possibly challenge existing paradigms related to its expansion.
Advancements in theoretical physics not only enrich our comprehension but also guide observational strategies, ensuring a symbiotic relationship between theory and practice.
The interplay between observational data and theoretical frameworks is essential for a comprehensive understanding of the universe's expansion.
The ongoing research directions in cosmology highlight a dynamic and multifaceted approach to understanding the expansion of the universe. These efforts continually reshape our knowledge and fuel further inquiries into the nature of the cosmos.
Future of the Universe
Understanding the future of the universe is crucial in the field of cosmology. It enables scientists to infer how cosmic structures, galaxies, and the universe itself will evolve over time. Various factors play significant roles in this future. Cosmological models, the influence of dark energy, and gravitational interactions between celestial bodies are just a few. The theories related to the universe's fate help lay a foundation for predicting long-term cosmic phenomena.
The future is not just a query of how things end but also about understanding the connective threads that bind various aspects of astrophysics. Grasping these connections can assist in refining calculations. This understanding may eventually give rise to novel perspectives on the universeβs structure, development and lifecycle.
The Fate of Cosmic Structures
Cosmic structures such as galaxies, clusters, and superclusters are complex systems that evolve. Their fate is determined by gravitational forces, dark energy, and the total matter density of the universe. Over time, galaxies may drift apart due to the continuous expansion. Eventually, they may become isolated, leading to a dynamic alteration in their features.
In the far future, based on current models, it's theorized that some galaxies will collide. For instance, the Milky Way and the Andromeda galaxy will merge within about 4.5 billion years. This merger will influence star formation, leading to an active period of new star data.
Additionally, as the universe expands, we can expect galaxy clusters, groups of galaxies held together by gravity, to become more dispersed. The gravitational pull that once clustered them together will decrease over billions of years, reshaping cosmic geography into a more sparse array of celestial bodies. Here, structures could maintain some stability for a significant period before becoming too dispersed.
Long-Term Predictions
Making long-term predictions about the universe involves integrating various theoretical models. One prevailing hypothesis is the "Heat Death" of the universe. This notion suggests that as stars exhaust their nuclear fuel, the universe will approach a state of maximum entropy where no usable energy remains.
Other possibilities include the "Big Crunch," where gravitational forces could eventually pull matter back together in a chaotic collapse, or the "Big Rip," a scenario dominated by dark energy which would ultimately tear apart galaxies, stars, planets, and even atomic structures.
In summary, the long-term predictions provide a framework for understanding the fate of the universe. They show us a continuum of scenarios based on physical laws and current observations. These predictions, rooted in rigorous scientific methodology, foster an environment for future research, prompting questions about the very nature of existence in an ever-evolving cosmos.
Technological Advances
Technological advances play a crucial role in the continuing exploration of the universe. In cosmology, these advancements enhance our ability to observe, analyze, and comprehend the vast complexities of the cosmos. As we strive to understand cosmic expansion, we rely on innovative tools and methodologies that expand our reach beyond the confines of Earth. This section will focus on the importance of space telescopes, probes, and computational tools in advancing our knowledge of the universe's expansion.
Space Telescopes and Probes
Space telescopes and probes are pivotal in the direct observation of celestial phenomena. Instruments like the Hubble Space Telescope and the James Webb Space Telescope have revolutionized our capacity to look deeper into space than previously possible. These telescopes function above Earth's atmosphere, minimizing light distortion and allowing for clearer imagery of distant galaxies, stars, and other cosmic entities.
The placement of telescopes in space means they can gather data in various wavelengths beyond the optical spectrum. This includes infrared and ultraviolet light, which are critical for studying many aspects of cosmic expansion. For example, the James Webb Space Telescope's ability to observe infrared light enables it to detect distant galaxies that are moving away from us, contributing to our understanding of redshift phenomena. Furthermore, space probes like the Parker Solar Probe give insights into our solar systemβs dynamics, informing us about the influences that may affect cosmic structures.
Key Benefits of Space Telescopes and Probes:
- Enhanced Imaging: Higher resolution images due to minimal atmospheric interference.
- Diverse Wavelength Observation: Ability to analyze different spectrums that provide varied information.
- Deep Field Surveys: Capability to examine areas of space previously thought to be empty, revealing new galaxies.
Computational Tools in Cosmology
Computational tools have emerged as essential components in understanding cosmology. With the increasing complexity of data collected from telescopes and probes, researchers now depend heavily on simulations and modeling to analyze this information. These computational efforts allow for predictions about cosmic behavior based on fundamental physics principles.
Tools like numerical simulations enable scientists to visualize and create models of cosmic expansion. They can simulate scenarios of galaxy formation, cosmic background radiation, and dark energy interactions, leading to a more accurate depiction of the universe's evolution over time.
Additionally, machine learning algorithms are becoming important in processing vast datasets derived from observations. By using pattern recognition, these algorithms can assist researchers in identifying anomalies or features that human observation might miss. This can prove crucial in understanding nuances in cosmic expansion patterns.
Considerations Regarding Computational Tools:
- Data Analysis: Managing large datasets from multiple sources and extracting meaningful insights.
- Model Complexity: Balancing between realistic models and computational resources.
- Integrating New Discoveries: Continuously updating models as new findings emerge is vital for accuracy.
Technological advances such as space telescopes and computational tools not only enhance our observational capacity but also deepen our understanding of the universe's fundamental nature and its expansion.
In summary, the integration of cutting-edge technology in cosmology directly influences how we perceive the universe. These advances open new avenues for exploration and understanding, ultimately illuminating the complex tapestry of natural laws governing cosmic expansion.
The End
The topic of the expansion of the universe is crucial for understanding modern cosmology. This article has presented a comprehensive overview of the foundational principles, historical context, and key evidence surrounding this subject. Recognizing how the universe is expanding not only informs scientific theories but also shapes our perception of the cosmos itself.
Summarizing Key Points
The central ideas covered in this article include:
- Definition and Historical Development: The expansion of the universe began as a theoretical concept, gaining momentum through the works of astronomers like Edwin Hubble.
- Key Theories: The Big Bang Theory and Steady State Theory offer diverse frameworks for understanding how the universe evolves. These theories underscore that our universe is not static but dynamic.
- Supporting Evidence: Observational data, such as Hubbleβs findings and the Cosmic Microwave Background Radiation, provide substantial support for the expansion theory.
- Mathematical and Philosophical Implications: The Friedmann Equations and concepts from general relativity reveal deep mathematical insights into the nature of spacetime, while philosophical implications engage with notions such as infinity and the human quest for understanding.
- Future Directions: Current research in observational astronomy and theoretical physics continues to explore the expansion, shaping our future understanding.
Implications for Future Research
As we look ahead, several considerations arise:
- Advancements in Technology: Future technological innovations, like more powerful space telescopes and advanced computational tools, will enhance our ability to observe and model the universe.
- Research on Dark Energy: Understanding dark energy remains critical, as it plays a significant role in cosmic acceleration and overall universe behavior.
- Expanding Theoretical Horizons: New theories that integrate quantum mechanics with cosmological principles could revolutionize our understanding of cosmic expansion.
- Interdisciplinary Approaches: Collaborations across fields, including physics, philosophy, and astronomy, may yield richer insights into the universeβs nature.
In summary, the expansion of the universe not only encapsulates fundamental aspects of the cosmos but also presents ongoing opportunities for research and discovery. Understanding this concept equips scientists and thinkers to appreciate the complexities of our universe and inspires future investigations into its many mysteries.