Understanding the Basics of Physics Made Easy
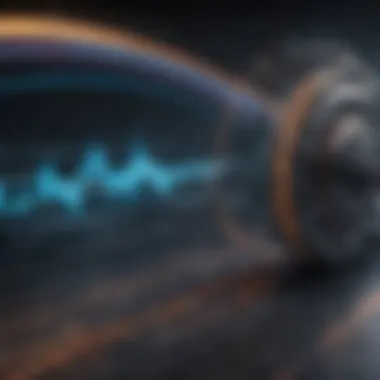
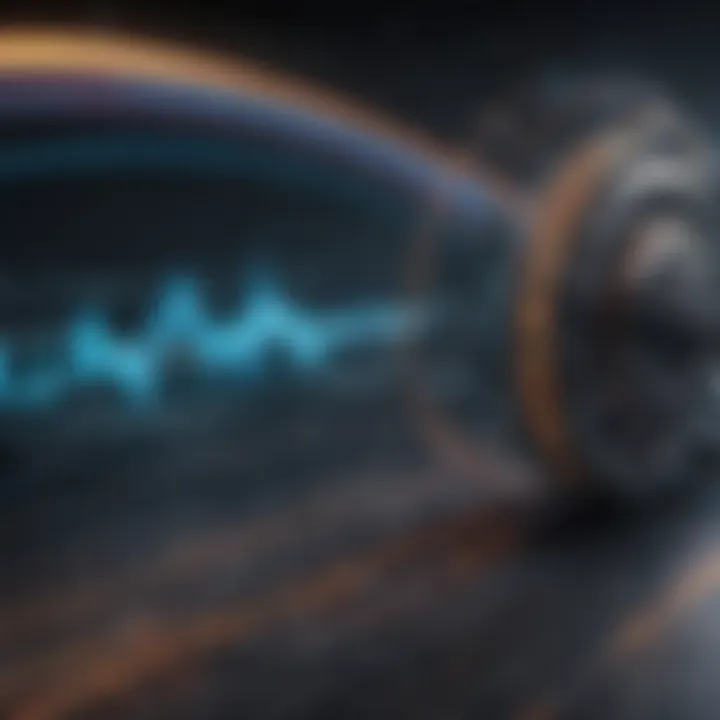
Intro
Physics is a field that often seems daunting to both new learners and even experienced individuals. It encompasses a variety of principles that explain how the universe operates. This article aims to uncover these basics, making the subject more approachable and understandable. Through exploring key concepts like motion, forces, and energy, readers will develop a clearer grasp of how these elements interact in everyday life.
By simplifying complex theories into digestible segments, we aim to remove the intimidation factor. The goal is to make physics not only accessible but also intriguing. In this journey, readers will encounter practical examples that demonstrate the relevance of physics in daily experiences.
Key Research Findings
Overview of Recent Discoveries
Recent studies in physics have advanced our understanding significantly. The discovery of gravitational waves is one example. These waves add a new dimension to our comprehension of the cosmos and relate directly to Einstein's theory of general relativity. Another important area is quantum mechanics. Research continues to reveal how particles behave in ways that defy traditional physics, challenging existing beliefs and prompting new theories.
Significance of Findings in the Field
The implications of these findings are far-reaching. The study of gravitational waves not only enhances astronomy but also opens doors for developing new technological tools. In quantum mechanics, insights can lead to breakthroughs in computing and security systems. Understanding these findings is crucial for anyone involved in physics, be it students, researchers, or educators.
"Understanding the key principles of physics allows individuals to appreciate the complexities of the universe."
Breakdown of Complex Concepts
Simplification of Advanced Theories
To truly grasp physics, one must first understand its core concepts. These include:
- Motion: This refers to the change in position of an object. It involves speed, velocity, and acceleration.
- Forces: A force is an interaction that causes an object to change its motion. Understanding types of forces like gravity, friction, and tension is essential.
- Energy: This is the ability to perform work. It can take various forms, including kinetic and potential energy.
By dissecting these ideas, we can unravel the complexities often associated with physics.
Visual Aids and Infographics
The use of visual aids can greatly enhance comprehension. Diagrams depicting gravitational forces or energy transformations simplify difficult material. Infographics that summarize important principles can serve as quick references that solidify understanding.
For example, consider a diagram illustrating Newton's laws of motion. Such visuals can clarify how forces act on objects, leading to better retention of the material.
Prelims to Physics
Physics serves as the foundational discipline in the natural sciences, addressing the fundamental principles that underlie the behavior of matter and energy. This article explores key concepts of physics, aiming to make these principles accessible and applicable to everyday situations. Understanding physics is not merely an academic pursuit; it has profound implications for technology, industry, and the environment.
Through clear explanations and relatable examples, the reader will gain insight into how physics shapes the world around us. By recognizing the role of physics in various aspects of life, from the functionality of household appliances to the mechanics of transportation, one can appreciate its relevance and importance. The goal of this section is to establish a strong foundation for readers, setting the stage for a deeper examination of classical mechanics, kinematics, forces, energy, and other critical areas of physics.
Definition and Scope
Physics can be defined as the study of matter, energy, and the interactions between them. It encompasses a broad range of topics, including mechanics, thermodynamics, electromagnetism, optics, and quantum mechanics. The scope of physics extends from the macroscopic level, which deals with everyday objects, to the microscopic scale that focuses on atomic and subatomic particles.
Significantly, physics is concerned not only with the forces and motions observed in large systems but also with the underlying principles that govern these phenomena. The discipline blends theoretical frameworks with empirical evidence gathered through experimentation. As such, physics is essential for anyone looking to understand how the universe functions at both a grand scale and a finer level.
Importance in Daily Life
The importance of physics in daily life cannot be overstated. Its principles provide the basis for technologies that enhance our quality of life and drive innovation. Here are a few key points highlighting its relevance:
- Technological Advancements: From smartphones to vehicles, many modern inventions rely on the principles of physics to function efficiently. Understanding these principles aids in further technological progress.
- Understanding Natural Phenomena: Knowledge of physics helps demystify everyday occurrences, such as why the sky turns blue, how rainbows form, and the mechanics behind flight.
- Safety and Engineering: Physics is crucial in engineering disciplines. Structural integrity, safety standards, and energy efficiency are all guided by physical principles.
"Physics seeks to uncover the laws of nature, making it a critical component of scientific inquiry across all fields."
In essence, physics is a valuable framework for comprehending the world, influencing nearly every sector and aspect of life. For students, educators, and professionals alike, grasping these concepts is vital to navigating both their personal and professional spheres.
Classical Mechanics
Classical mechanics is a foundational pillar in physics, providing insights into the motion of objects and the forces that influence this motion. It is essential to understand various physical phenomena in our daily lives. By studying classical mechanics, individuals equip themselves with the ability to analyze and predict how objects behave in different situations. This knowledge has vast implications, from engineering to daily activities like driving a car or playing sports.
Fundamental Concepts
Mass
Mass is a measure of the amount of matter in an object. It plays a crucial role in classical mechanics, as it directly affects the gravitational attraction and inertia of an object. A key characteristic of mass is that it is a scalar quantity, meaning it does not have a direction, only a magnitude. This simplicity makes mass an effective way to describe physical systems without unnecessary complexity.
In this article, mass is emphasized as a fundamental concept because it is inherently linked to other physical principles. Every object in motion has mass, making it essential for understanding concepts like force and acceleration. One unique feature of mass is its consistency; it does not change regardless of the object's location in the universe. This ensures that calculations remain reliable and straightforward, but does come with limitations when considered in relativistic contexts.
Force
Force is an interaction that causes an object to change its velocity or direction. In classical mechanics, force is represented by Newtonβs second law as the product of mass and acceleration. The key characteristic of force is that it is a vector quantity, possessing both magnitude and direction. This quality allows for a rich analysis of interactions in physical systems.
In this article, force is treated as a primary component for understanding motion. Each force contributes to the overall behavior of a system, helping to break down complex problems into manageable parts. A unique feature of force is its ability to combine with other forces; for example, when multiple forces act on an object, they can be summed to predict the resultant motion. This collective view is beneficial, yet it can complicate matters if too many forces are involved.
Acceleration
Acceleration describes how quickly an object's velocity changes over time. It is a key concept in classical mechanics and is fundamental to understanding motion. The characteristic that makes acceleration particularly significant is its direct relationship to both force and mass through Newtonβs second law.
Acceleration is beneficial in this article as it quantifies motion changes, helping readers visualize and predict scenarios. Its unique feature is that it can be both positive and negative, representing increases and decreases in speed, respectively. This duality can sometimes be confusing, but it adds depth to the understanding of motion dynamics.
Laws of Motion
Newton's First Law
Newton's First Law states that an object at rest stays at rest, and an object in motion stays in motion unless acted upon by an external force. This law is essential as it describes the concept of inertiaβthe resistance of any physical object to any change in its velocity. This law is considered beneficial due to its straightforward nature. It introduces the idea of equilibrium, which is crucial when analyzing static and dynamic systems.
A unique aspect of Newton's First Law is its implication in everyday scenarios. For example, when a car suddenly stops, the passengers lurch forward due to inertia. This example illustrates the law's real-world relevance but can be misleading if one does not consider the force at play.
Newton's Second Law
Newton's Second Law quantitatively relates force, mass, and acceleration in the equation F = ma. This law is significant as it allows predictions about the motion of objects under various forces. The characteristic of this law that stands out is its versatility across different contexts, making it invaluable in both theoretical and practical applications.
In this article, Newton's Second Law is a popular choice because it provides a clear framework for understanding how forces affect motion. Its unique feature comes from the ability to manipulate the equation to find unknown variables, which is beneficial for problem-solving. However, misunderstanding the vector nature of forces might lead to errors in applications.
Newton's Third Law
Newton's Third Law states that for every action, there is an equal and opposite reaction. This principle demonstrates the interactions between forces and is fundamental for understanding various physical systems. The key characteristic of this law is its emphasis on mutual forces acting between objects, highlighting the interconnectedness of systems.
In this article, Newton's Third Law is included because it emphasizes the duality of forces. It is a critical consideration in engineering and physics, influencing everything from vehicle safety designs to rocket propulsion. However, its unique nature can sometimes lead to misconceptions, especially when analyzing systems where forces seem to act independently.
Kinematics
Kinematics is an essential branch of mechanics that focuses on the motion of objects without considering the forces that cause that motion. This topic holds a significant place in physics, especially for beginners, as it lays the groundwork for understanding how and why objects move. Grasping kinematics helps in interpreting various phenomena in daily life, from the trajectory of a thrown ball to the movements of vehicles on a street. Recognizing patterns in motion is crucial when we analyze more complex systems later in our studies.
Understanding Motion
Understanding motion is a primary aspect of kinematics. It describes how objects change their position over time. Motion can be categorized into different types, which is a critical part of kinematics.
Types of Motion
Types of motion include linear, rotational, and periodic motion. Each type has unique characteristics that serve different contexts in physics. For instance, linear motion describes an object moving along a straight path, while rotational motion involves an object spinning around an axis. Periodic motion is when an object moves in a recurring pattern, such as swinging back and forth.
The key characteristic of types of motion lies in their patterns. By categorizing motion, students can apply the relevant equations and principles effectively. This is a beneficial choice for this article as it simplifies complex ideas into digestible concepts.
A unique feature of types of motion is their universal application in various fields, from engineering to robotics. Understanding whether motion is linear or rotational can influence how a problem is approached, highlighting the advantages of clear categorization.
Graphing Motion
Graphing motion is another vital concept within kinematics. It involves visually representing an object's position, velocity, or acceleration over time, which provides greater insight into its behavior.
A key characteristic of graphing motion is its ability to simplify complex relationships. For instance, a velocity-time graph can immediately show how an object accelerates or decelerates. This approach is beneficial for students learning how to interpret data and make predictions.
The advantage of graphing motion is that it transforms abstract numbers into visual insights, which makes understanding easier. However, one must still be careful with interpretationβmisreading a graph can lead to incorrect conclusions.
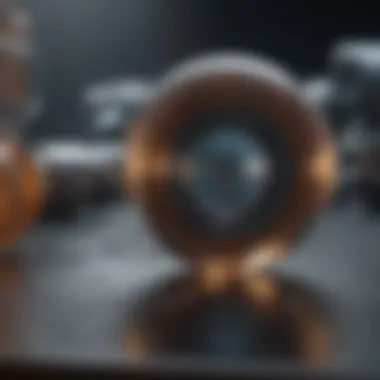
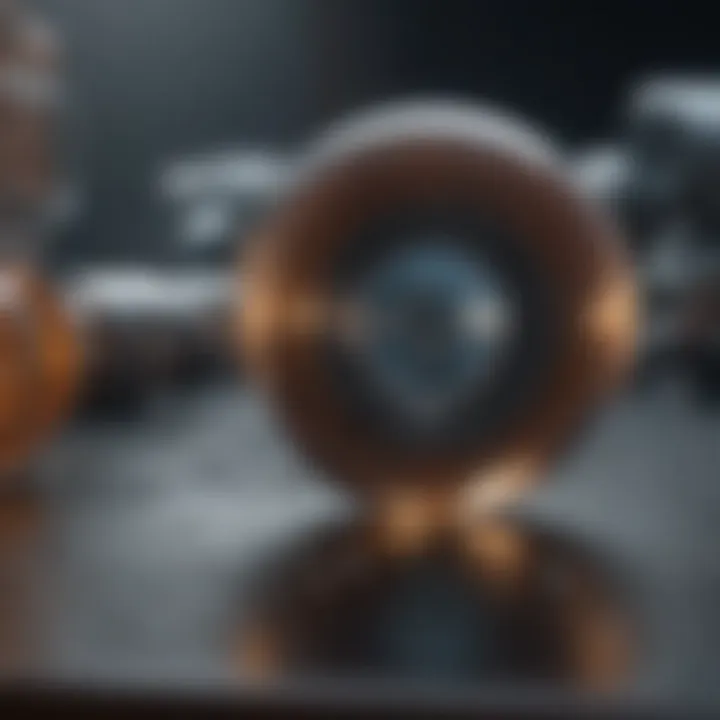
Equations of Motion
Equations of motion are fundamental to understanding kinematics. They allow physics students to compute variables like position, velocity, and time under various conditions.
Uniform Acceleration
Uniform acceleration refers to a situation where an object's acceleration remains constant. This straightforward concept is critical in many calculations, aiding students in their grasp of motion dynamics. The equations governing uniform acceleration are some of the first formulas that physics students encounter. They allow for the prediction of future positions based on current values.
The key aspect here is predictability. Uniform acceleration simplifies calculations, making it a beneficial section for learners. However, it is important to note that real-life scenarios often involve variable acceleration, which can complicate practical applications.
Free Fall
Free fall refers to the motion of an object that is falling solely under the influence of gravity. This principle is a direct result of the gravitational force acting on an object, and it applies universally, simplifying the study of motion.
The unique aspect of free fall is that whether an object is heavy or light, all objects fall at the same rate in a vacuum, assuming no air resistance. This characteristic makes it a popular and essential topic in kinematics. On the downside, studying free fall requires a controlled environment to eliminate other forces at play.
Forces and Interactions
Forces and interactions constitute core principles in physics, fundamentally influencing how objects behave in the universe. This section aims to clarify different types of forces, including gravitational and electromagnetic forces, as well as dissect the concept of friction. Understanding these aspects is crucial for approaching many practical applications, whether in engineering, astrophysics, or everyday mechanics. Grasping the nuances of forces enhances oneβs ability to apply these principles effectively in real-world scenarios.
Types of Forces
Forces can be classified into several categories based on their origins and effects. The major types include gravitational and electromagnetic forces, each with its own unique properties and implications in various fields.
Gravitational Forces
Gravitational forces are a pervasive element in physics. They relate to the attraction between masses, governed by Newton's law of universal gravitation. The key characteristic of gravitational forces is their tendency to act at a distance; they do not require direct contact between objects. This makes them a popular subject in studies aiming to explain celestial mechanics and motion of planets.
One beneficial feature of gravitational forces is their predictability. Their equations are straightforward, making calculations relatively easy. For example, the force can be determined using the formula:
[ F = G \fracm_1 m_2r^2 ]
where ( F ) is the gravitational force, ( G ) is the gravitational constant, ( m_1 ) and ( m_2 ) are the masses, and ( r ) is the distance between the centers of mass.
However, despite their predictable nature, gravitational forces have limitations. They are notably weak compared to other forces, which can sometimes make their effects seem negligible in small-scale interactions.
Electromagnetic Forces
Electromagnetic forces arise from the interaction between charged particles. They are among the most powerful forces in nature, responsible for the structure of atoms. The key characteristic of electromagnetic forces is their dual nature; they can both attract and repel. This property enables a variety of significant applications in technology, from electric circuits to magnetic storage devices.
A unique feature of electromagnetic forces is their range, which can be infinite unlike gravitational forces. This quality allows them to influence a vast array of phenomena, from the bonding of atoms to the propagation of light. However, these forces also have complexities. The mathematics governing them is often more intricate, involving calculus and vector analysis, which can pose challenges when applied to various systems.
Friction
Friction is another essential topic within the study of forces. It opposes motion between two surfaces that are in contact and is often a vital consideration in engineering and design.
Static and Kinetic Friction
The distinction between static and kinetic friction is crucial in physics. Static friction acts on objects at rest, preventing them from moving until a certain threshold is met. In contrast, kinetic friction occurs when two surfaces are sliding past each other. The key characteristic of static friction is its ability to adjust to the applied force, making it stronger than kinetic friction for a given pair of surfaces.
This aspect makes static friction a favorable choice in designing safety features in vehicles, where stopping power is critical. The unique feature of kinetic friction is its effect on moving objects, which typically results in energy loss in the form of heat. Each type of friction has its own advantages, such as traction in vehicles (static) versus energy dissipation in brakes (kinetic).
Applications of Friction
Friction has numerous practical applications across various fields. It is vital in everyday tasks, like walking or driving. Friction enables the brakes of a car to function properly, as it helps slow down the vehicle safely. A notable characteristic of friction is its presence in virtually all mechanical systems, thus making it a topic of significant importance.
However, friction also presents challenges. Excessive friction can lead to wear and tear on machinery, requiring regular maintenance. For engineers, optimizing friction helps balance safety and efficiency in design. In some cases, reducing friction can enhance performance, while in others, increasing it is essential to prevent slipping.
Energy Concepts
Energy is a fundamental principle that permeates every aspect of physics. Understanding energy concepts not only lays the groundwork for comprehending various physical phenomena but also connects diverse areas in science and technology. In this section, we will delve into two primary types of energy: kinetic energy and potential energy. We will also examine the notion of conservation of energy, which is a cornerstone of physics.
Types of Energy
Energy can manifest in different forms depending on the situation. In this discussion, two forms are particularly significant.
Kinetic Energy
Kinetic energy refers to the energy an object possesses due to its motion. It is defined mathematically as:
[ KE = \frac12mv^2 ]
where m is the mass of the object and v is its velocity. A key characteristic of kinetic energy is its direct relation to both mass and velocity. The greater the mass or speed of the object, the more kinetic energy it has. This concept is beneficial for understanding the motion of vehicles, projectiles, and even particles in physics.
A unique feature of kinetic energy lies in its convertibility. For instance, when a moving car comes to a stop, its kinetic energy converts into heat energy due to friction. This conversion can illustrate real-world applications, such as in regenerative braking in electric cars, where the kinetic energy is captured and reused. However, the downside is that kinetic energy can lead to dangerous consequences if not controlled properly, as seen in high-impact collisions.
Potential Energy
Potential energy refers to the stored energy in an object due to its position or state. A common example is gravitational potential energy, which can be calculated using:
[ PE = mgh ]
where m is the mass, g is the acceleration due to gravity, and h is the height above the ground. The key characteristic of potential energy is that it is associated with positionβhence, an object at a higher elevation possesses more gravitational potential energy.
In this article, potential energy serves as an important concept because it emphasizes stability and the potential for energy transfer. For example, a drawn bow has potential energy waiting to be converted into kinetic energy when the string is released. This conversion showcases how energy is interlinked across different forms, allowing us to understand various applications in engineering and building systems. The disadvantage of potential energy, however, is its dependence on an object's state or configuration; a structure that is not properly designed may lead to energy loss or instability.
Conservation of Energy
The law of conservation of energy states that energy cannot be created or destroyed, only transformed from one form to another. This principle is vital in all realms of physics, guiding how we analyze systems, from simple machines to complex ecosystems. Understanding this law allows for predictions and calculations that are essential in scientific research and engineering.
In daily life, conservation of energy is seen in multiple scenarios, such as energy-efficient buildings, where thermal energy is conserved. In a closed system, the total energy remains constant, although forms may change. For example, when you drop an object, its gravitational potential energy is transformed into kinetic energy as it falls.
"Energy cannot be created or destroyed, it can only be changed from one form to another."
This foundational concept highlights the interconnectedness of motion, heat, and work across various settings. Thus, mastering energy concepts not only enhances oneβs comprehension of physics but also provides insights into practical applications that significantly impact modern life.
Waves and Sound
Waves and sound are fundamental aspects of physics that play a significant role in various scientific and practical disciplines. Understanding these elements is crucial for grasping more complex physical concepts later. In this section, we will delve into the nature of waves, sound waves, their properties, and their applications. This topic is essential not only for students and researchers but also for educators and professionals seeking to enhance their knowledge.
Nature of Waves
Waves are disturbances that transfer energy from one point to another without transferring matter. They can be categorized into two main types: transverse waves and longitudinal waves. Each type of wave has distinct characteristics that make it suitable for different applications.
Transverse vs Longitudinal Waves
Transverse waves are waves where the displacement of the medium is perpendicular to the direction of wave propagation. A common example is waves on a string or electromagnetic waves like light.
In contrast, longitudinal waves are waves where the displacement of the medium is parallel to the direction of wave propagation. Sound waves in air are typical examples of longitudinal waves.
The key characteristic of transverse waves is their ability to travel through a vacuum, making them integral to understanding phenomena such as light and radio waves. Longitudinal waves, however, are beneficial because they can travel through different media, like solids, liquids, and gases, which showcases their versatility and practical applications in acoustics and engineering. An advantage of longitudinal waves is that they tend to transmit energy more efficiently in media like air.
Wave Properties
Understanding wave properties is crucial for multiple areas in physics, including acoustics and optics. Key properties of waves include amplitude, wavelength, frequency, and speed.
Amplitude measures the maximum displacement of a wave from its equilibrium position. Wavelength is the distance between two consecutive points in phase on a wave, such as crest to crest. Frequency refers to the number of wave cycles that pass a given point per unit time.
The characteristic of waves being able to propagate energy is particularly important for many applications, including communication technologies and sound engineering. A unique feature of waves is their ability to interfere and produce complex patterns. This property can be beneficial in designing systems like noise-canceling headphones, which utilize the principle of constructive and destructive interference.
Sound Waves
Sound waves are a specific type of longitudinal wave that propagates through various mediums. They are critical to many everyday experiences and technologies, such as music, speech, and communication devices.
Production of Sound
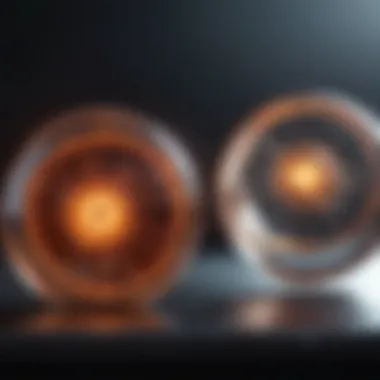
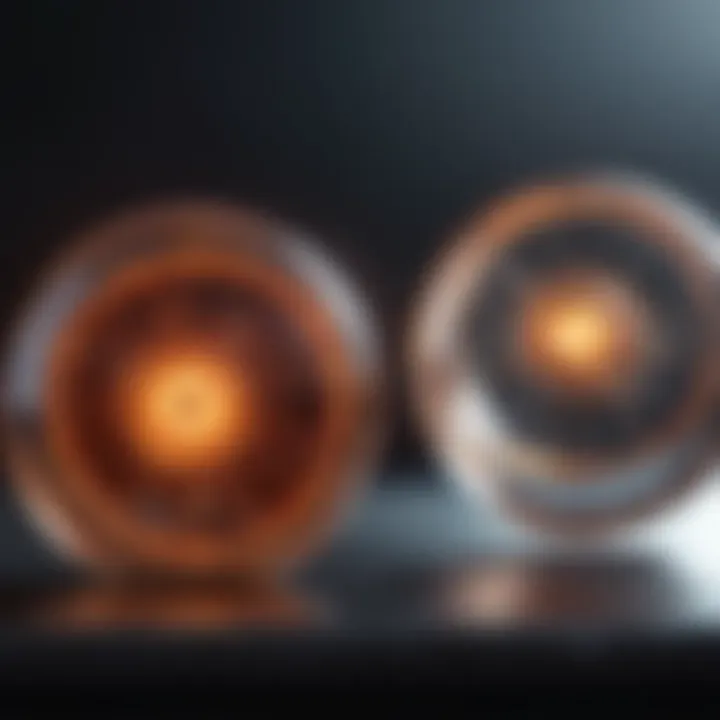
Sound production involves the vibration of an object, which creates pressure waves in the surrounding medium. These vibrations cause particles in the medium, such as air, to move, resulting in the propagation of sound waves.
The key characteristic of sound production is that it requires a medium to travel, which differentiates it from electromagnetic waves. This aspect is relevant since it limits the transmission of sound in outer space, where no air exists. The unique feature of sound waves is that they can be manipulated through pitch and volume changes, which are fundamental in music and audio engineering. However, sound can also be attenuated through absorption and scattering, which are disadvantages in certain applications such as long-distance communication.
Characteristics of Sound
Characteristics of sound waves include pitch, loudness, and timbre, all of which contribute to our perception of sound.
Pitch is determined by the frequency of the sound wave, while loudness relates to the amplitude. Timbre is the quality or color of the sound that allows us to distinguish different sounds.
Understanding these characteristics is essential for creating effective audio systems and enhancing communication technologies. The ability to manipulate sound for various effects, such as in music production, illustrates the practical benefits of studying sound waves. However, factors like distance and environmental obstacles can affect sound quality and transmission, which poses challenges in certain contexts.
The study of waves and sound is not merely an academic pursuit; it is a gateway to understanding the intricate relationships that govern our interactions with the world.
Light and Optics
Light and optics play a crucial role in the study of physics, as they govern how we interact with our world. Understanding light allows us to delve into a variety of phenomena, from the way we perceive objects around us to advanced technologies used in communication and imaging. In this section, we will examine the nature of light and the functionality of lenses and mirrors, providing essential insights into these fundamental concepts.
Nature of Light
Wave-Particle Duality
Wave-particle duality is a significant characteristic of light, encapsulating the idea that light exhibits properties of both waves and particles. This duality is not just a theoretical concept; it has practical implications in various fields of physics. The key characteristic of this concept lies in its ability to explain phenomena such as interference and diffraction, which are inherently wave-like behaviors, as well as the photoelectric effect where light interacts as particles, called photons.
The inclusion of wave-particle duality in this article is beneficial as it addresses a core debate in physics and provides a basis for understanding light's behavior in different contexts. It opens the door to discussions about quantization and energy levels in atoms, which are fundamental for comprehending more complex theories in modern physics. However, one disadvantage of wave-particle duality is that it can introduce confusion for beginners, as it challenges the classical view of light being solely a wave or solely a particle.
Speed of Light
The speed of light is another critical aspect of light that influences many areas of physics. Light travels at approximately 299,792 kilometers per second in a vacuum, a fact that has profound implications for relativity and time dilation. Understanding the speed of light is essential for grasping how distance and time are interconnected in the universe. The key characteristic of the speed of light is that it represents a universal constant that applies under various conditions.
This concept is useful for our article as it provides a foundation for advanced topics such as Einstein's theory of relativity. The unique feature of the speed of light is that it is immutable in a vacuum, but it slows down when passing through different mediums like glass or water. This variability can complicate certain calculations in optics but is crucial for applications such as fiber optics communication, where light speed influences data transfer efficiency.
Lenses and Mirrors
Types of Lenses
Lenses are optical devices that manipulate light to form images. The two main types of lenses are convex and concave. Convex lenses converge light rays to a focal point, making them useful in applications like magnifying glasses and cameras. Concave lenses, on the other hand, diverge light rays, which can be helpful in corrective glasses for nearsightedness.
The inclusion of this topic in the article is instrumental, as it highlights various applications of lenses in everyday life. A unique feature of lenses is their ability to alter light paths based on shape, which can be both advantageous and disadvantageous. For instance, while convex lenses can magnify images, they can also produce distortions if not properly designed.
Reflection and Refraction
Reflection and refraction are two fundamental principles regarding light behavior. Reflection occurs when light bounces off a surface, while refraction involves the bending of light as it passes from one medium to another. This section is essential in understanding how we see the world, as both phenomena dictate how images are formed and perceived.
The key characteristic of reflection is its dependability on the surface texture. A smooth surface generates a clear reflection, while a rough surface scatters light. Refraction, distinguished by its dependence on medium density, is critical for understanding how lenses work in optical devices. Both processes present opportunities and challenges in various fields, such as designing efficient optical instruments, where achieving clarity and minimizing distortion is paramount.
Thermodynamics
Thermodynamics is a critical area of physics that deals with heat, energy, and their transformations. Understanding thermodynamics is essential for grasping how energy works in the physical world, and it influences many practical applications. It provides insight into systems ranging from tiny engines to weather patterns. The relevance of thermodynamics in daily life cannot be understated as it plays a role in many phenomena we observe.
Laws of Thermodynamics
The laws of thermodynamics are principles that describe how energy moves and changes within a system. There are four primary laws, usually numbered from zero to three:
- Zeroth Law of Thermodynamics: If two systems are each in thermal equilibrium with a third system, then they are in thermal equilibrium with each other.
- First Law of Thermodynamics: This law states that energy cannot be created or destroyed. It can only be transformed from one form to another, which emphasizes the conservation of energy.
- Second Law of Thermodynamics: This law introduces the concept of entropy, indicating that in any energy transfer, the total entropy of a system will either increase or remain the same.
- Third Law of Thermodynamics: As temperature approaches absolute zero, the entropy of a perfect crystal approaches a constant minimum.
These laws establish the foundation for understanding energy interactions, which is crucial for various fields, including engineering, biology, and environmental science.
Applications in Real Life
Refrigeration
Refrigeration is a practical application of the principles of thermodynamics. It involves the transfer of heat from one location to another, effectively cooling a space or substance. The key characteristic of refrigeration is its ability to remove heat from a cold space, maintain low temperatures, and preserve food and other perishables. This is a beneficial choice in many households and industries, allowing for food safety and storage convenience.
A unique feature of refrigeration systems is the use of refrigerants, chemicals that absorb and release heat efficiently. This method of heat transfer has the advantage of maintaining consistent temperatures, but it comes with disadvantages such as the environmental impact of certain refrigerants.
Heat Engines
Heat engines are devices that convert thermal energy into mechanical work, illustrating another important application of thermodynamics. The key characteristic of heat engines is their efficiency in transforming energy from fuels into useful work. This characteristic makes them a cornerstone of transportation and electricity generation.
One unique feature of heat engines is their reliance on cyclical processes, such as the Carnot cycle, to maximize efficiency. While they are widely used and effective, heat engines also face challenges including waste energy and pollution, which are increasingly important as energy discussions evolve.
Understanding thermodynamics helps us to appreciate the energy systems around us, from everyday appliances to industrial processes.
In summary, the significance of thermodynamics extends into everyday life, influencing both the technology we use and our understanding of energy conservation and efficiency. By studying thermodynamics, we gain insights that apply to both practical and theoretical aspects of physics.
Modern Physics
Modern physics is not just an extension of classical physics; it represents a transformation of how we understand the universe at fundamental levels. The concepts within modern physics challenge traditional views, and they provide insights that have wide-ranging implications in both theoretical and practical realms. Understanding modern physics is essential as it lays the groundwork for various technologies and innovations that permeate our daily lives.
Among its most critical achievements are the theories of relativity and quantum mechanics, two pillars that not only redefine how we look at space, time, and matter but also propel advancements in various industries. Modern physics informs fields such as electronics, telecommunications, and medicine, merging theoretical knowledge with practical applications. Moreover, the exploration of modern physics continues to inspire curiosity and investigation, serving as a catalyst for scientific developments and philosophical discussions alike.
Preface to Relativity
Albert Einstein's theory of relativity revolutionized the understanding of time and space. It comprises two main components: special relativity and general relativity. Special relativity, introduced in 1905, posits that the laws of physics are the same for all observers in uniform motion relative to one another, hinting that the speed of light is constant. This leads to concepts such as time dilation, where time appears to pass more slowly for an object moving at significant speeds relative to a stationary observer.
General relativity, published in 1915, expands these ideas to include gravity. It describes gravity not as a force but as a curvature of spacetime caused by massive objects. The implications of this are profound, offering a framework that explains gravitational phenomena and making accurate predictions about the behavior of celestial bodies. This theory has been confirmed through numerous experiments and observations, reinforcing its importance in modern physics.
"The theory of relativity is a cornerstone of modern physics, providing deeper insight into the universe."
The relevance of relativity extends beyond theoretical constructs; it enables technologies such as GPS, which relies on precise timing adjustments made possible by relativity. Without this understanding, inaccuracies in navigation and timing would lead to significant errors in everyday life.
Quantum Mechanics Basics
Quantum mechanics is another foundational aspect of modern physics, dealing with phenomena at microscopic scales. It presents a world that is inherently probabilistic rather than deterministic, challenging the classical intuitions we hold about nature. Key principles include wave-particle duality, which asserts that particles can exhibit properties of both waves and particles, and the uncertainty principle, which states that certain pairs of physical properties cannot be simultaneously measured with arbitrary precision.
Quantum mechanics explains various phenomena, from the behavior of electrons in an atom to the principles behind lasers and semiconductors. This field has led to remarkable innovations such as quantum computing and quantum cryptography, which promise to reshape technology and enhance security.
As we delve deeper into quantum discussions, concepts such as superposition and entanglement reveal the complexities of the quantum realm. These principles not only underscore the richness of quantum theory but also highlight the ongoing research necessary to fully harness quantum mechanics for practical applications.
Popular references and educational platforms discuss these concepts, providing insights for those interested in modern physics. For more information, you can visit Wikipedia or Britannica.
In essence, modern physics intertwines with the fabric of technology and scientific understanding, making it a vital field of study for the future.
Scientific Methodology
Scientific methodology is critical for understanding and applying the principles of physics. This section explores the systematic approach that scientists use to study natural phenomena, which is essential for fostering accurate knowledge and innovation in the field. The importance of scientific methodology lies in its structured framework that guides researchers through observation, hypothesis formation, experimentation, and validation.
Nature of Scientific Inquiry
Scientific inquiry is the process through which observations lead to questions, and ultimately to knowledge. It starts with identifying phenomena in the physical world and forms the basis for further exploration. In the realm of physics, this means meticulously observing behaviors of materials or energy under specific conditions.
Different types of inquiries can lead to unique discoveries. One approach may investigate the relationships between force and motion, while another might focus on the properties of light. Thus, recognizing the nature of scientific inquiry allows physicists to formulate hypotheses that can be tested and refined over time. This iterative process benefits both new learners and established experts in the field.
Experiments in Physics
Experiments in physics form the backbone of empirical research. They are essential for testing hypotheses derived from scientific inquiries. Well-designed experiments must be repeatable, controlled, and based on precise measurements.
Designing Experiments
Designing experiments involves careful planning to ensure that the outcomes can provide valid insights into the phenomena being studied. A well-structured experiment includes a clear objective, properly defined variables, and systematic methods for data collection. The key characteristic of designing experiments is the necessity for control, as it helps isolate the effects of the variable being tested.
This methodology is beneficial because it allows researchers to eliminate bias and ensure the reliability of results. One unique feature of designing experiments is the use of control groups, which serve as a benchmark for comparison. However, it does present challenges, such as the need for precise materials and equipment that can impact the overall outcome.
Analyzing Results
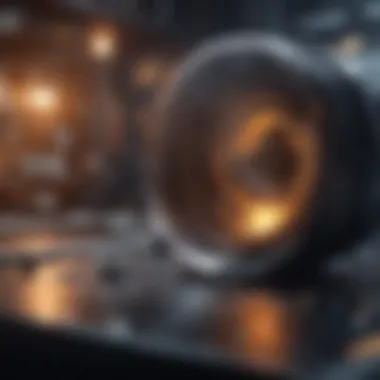
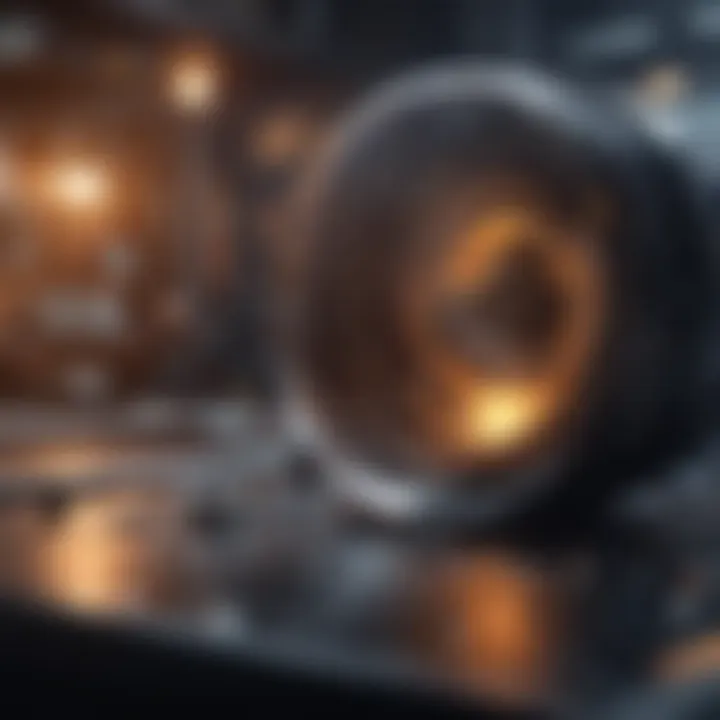
Analyzing results is equally important in the scientific methodology. Once data is collected, it requires careful examination to draw meaningful conclusions. The key characteristic of analyzing results is the use of statistical methods to interpret data accurately. This approach enhances the credibility of findings and allows for greater clarity in understanding complex concepts.
The unique nature of analyzing results involves not just the immediate data but also the context and implications of those findings in the broader scope of physics. Moreover, strong analytical skills are crucial for distinguishing between correlation and causation.
Key advantages of a thorough analysis include the ability to derive comprehensive insights that can lead to further research and innovation. Conversely, it can be challenging due to potential errors in data collection and interpretation that must be meticulously addressed.
"The true excitement of physics lies in designing experiments and analyzing the results that lead to new understanding of natural laws."
Adopting robust scientific methodology enhances the learning experience, igniting curiosity and advancing the field of physics. Engaging with these processes prepares students, researchers, and educators to contribute effectively to ongoing discoveries and innovations.
Practical Applications of Physics
Physics is not just an abstract field of study; it has tangible impacts on daily life and modern society. Understanding the practical applications of physics is crucial for students, researchers, educators, and professionals. This section emphasizes the significance of applying physics principles to real-world scenarios. It will explore how physics underpins advancements in technology and medicine while highlighting its role in shaping our environment.
Technology and Innovation
Physics has been a driving force behind numerous technological innovations. From communication systems to energy-efficient devices, the applications of physical principles are vast. Here are some key areas where physics has significantly influenced technology:
- Electromagnetic Devices: Devices like smartphones and computers rely on electromagnetic theories. Advances in magnetism and electricity lead to miniaturization and enhanced performance of electronic components.
- Renewable Energy: Understanding thermodynamics and fluid dynamics aids in the development of solar panels and wind turbines. Physicists work to optimize these technologies for better efficiency and sustainability.
- Nanotechnology: Physics plays a crucial role in manipulating materials at the molecular level. Innovations like nano-coatings improve product durability and functionality.
Innovation in technology often follows research in physics. The synergy between theoretical models and practical applications leads to groundbreaking discoveries, which enhance our quality of life. Thus, grasping physics concepts is essential for anyone entering fields related to engineering, computer science, or environmental studies.
Medical Physics
The domain of medical physics highlights the intersection of physics and healthcare. It focuses on the delivery of safe and effective medical care through various technologies. Its applications range from imaging techniques to treatment methodologies:
- Diagnostic Imaging: Techniques like X-rays, MRI (Magnetic Resonance Imaging), and CT (Computed Tomography) scans rely on principles of radiation and wave physics. Medical physicists ensure that these imaging methods are both effective and safe, guiding doctors in accurate diagnoses.
- Radiation Therapy: For cancer treatment, understanding radiation physics is pivotal. Medical physicists develop treatment plans that maximize tumor dose while minimizing exposure to healthy tissue.
- Instrumentation and Calibration: Medical equipment must meet stringent standards. Physicists play a vital role in calibrating tools used in therapies and diagnostics, ensuring precision and reliability.
In summary, practical applications of physics span both technology and medicine. This knowledge empowers professionals to innovate and improve existing systems, addressing current challenges in society. Through the lens of physics, real-world problems are approached with analytical thinking and empirical methods, fostering progress and sustainability in various fields.
Environmental Physics
Environmental physics studies the interaction between physical processes and the environment. This branch of physics is essential for understanding the impact of human activities on nature. It encompasses a wide range of topics including climate change, renewable energy sources, and sustainable practices. The study of environmental physics contributes to the development of solutions aimed at reducing the ecological footprint.
Concepts of Sustainability
Sustainability refers to meeting the needs of the present without compromising the ability of future generations to meet their own needs. Environmental physicists analyze how various forces, such as thermodynamics and energy transfer, affect sustainability. This analysis helps in developing systems that optimize resource use, minimize waste, and reduce emissions.
Key areas of focus within sustainability include:
- Renewable Energy: Technologies such as solar, wind, and hydropower offer alternatives to fossil fuels, lowering greenhouse gas emissions.
- Energy Efficiency: Using less energy to perform the same function and improving building designs can significantly reduce overall energy consumption.
- Waste Management: Understanding waste processes helps in recycling efforts and minimizing landfill use.
These principles are crucial in creating policies that promote environmental health and safety.
Impact of Physics on Nature
Physics plays a pivotal role in understanding the natural world. From weather patterns to ecosystems, physical laws govern the dynamics of our planet. The knowledge of these laws helps in predicting environmental changes.
For example:
- Climate Change: The laws of thermodynamics explain how energy from the sun influences Earth's climate. Understanding heat transfer processes helps in predicting temperature rises and impacts on global weather.
- Ecosystem Dynamics: Physical forces such as gravity and motion shape landscapes and affect species distribution. For example, the flow of rivers is vital for nutrient distribution in ecosystems.
"Physics not only elucidates natural phenomena but also provides tools for safeguarding our environment."
Thus, understanding the intersection of physics and nature is vital for developing effective environmental policies and conservation strategies. Whether it's through renewable energy innovations or understanding climate dynamics, environmental physics is essential for sustainable development.
Education in Physics
Education in physics is critical for many reasons, impacting not just scientific knowledge but also the overall intellectual growth of individuals. Engaging with physics education promotes an understanding of fundamental principles that govern the natural world. This understanding serves as a foundation for critical thinking and problem-solving skills, which are valuable in various disciplines.
Through structured education in physics, learners gain insights into concepts such as motion, energy, and forces. This allows them to connect theoretical knowledge with real-world applications. For instance, comprehending how forces interact helps in various fields, including engineering, environmental science, and technology.
Additionally, physics education encourages curiosity. It shifts the way students perceive challenges and equips them with the tools needed to address complex issues. Students often find themselves relating physics to daily experiences, such as understanding how vehicles move, the principles behind household appliances, and even natural phenomena.
"Physics is not only a body of knowledge, but also a way of thinking."
Investing in education in physics also fosters innovation. Understanding the laws of physics is integral for future technological advancements. As learners grasp the concepts, they often contribute to research and development within their respective fields.
In summary, education in physics plays a significant role in broadening one's perspective, enhancing analytical abilities, and fostering innovation. The critical nature of physics in many scientific endeavors ensures that its study remains relevant and impactful over time.
Modern Teaching Techniques
Modern teaching techniques in physics focus on making the subject more accessible and engaging. Traditional methods often rely on rote learning, which does not cater well to all learning styles. In contrast, contemporary strategies emphasize interactive learning, experimentation, and collaborative projects.
Techniques such as flipped classrooms allow students to learn at their own pace. They can review materials outside of class, enabling more effective use of classroom time for discussions and hands-on activities. This approach also helps to foster a deeper understanding of the material.
Another effective method is the incorporation of technology. Using simulations and digital tools helps students visualize complex concepts. Software like PhET simulations presents an interactive way to explore physics phenomena, making learning more enjoyable and effective.
Ultimately, employing modern teaching techniques enhances student engagement and helps to demystify physics, transforming it into a subject that is not just learned but experienced.
Online Resources and Communities
The rise of online resources has revolutionized the way physics is taught and learned. A wealth of information is available, from video lectures to online courses that cater to various learning styles. Websites like Khan Academy provide free resources that simplify complex topics in physics, making them accessible to learners worldwide.
In addition to structured courses, online forums and communities offer platforms for collaboration and discussion. Websites like Reddit host communities where students, educators, and enthusiasts can share knowledge, seek advice, and engage in conversations about physics. This peer interaction can significantly enhance understanding and retention of material.
Social media platforms such as Facebook also facilitate learning by connecting individuals with similar interests. Groups focused on physics can provide support and resources, fostering a collaborative learning environment.
Future Directions in Physics Research
The exploration of future directions in physics research is crucial for understanding how the discipline can evolve to address complex questions and problems. With rapid advancements in technology and a growing interplay between physics and other scientific fields, the importance of this area cannot be overstated. As we strive to dissect the nature of our universe, insights gained from upcoming research will be pivotal in shaping both scientific knowledge and real-world applications.
Emerging Technologies
Emerging technologies in physics promise to unlock a new spectrum of possibilities. These innovations can enhance our understanding of existing principles and lead to groundbreaking discoveries. Key technologies that are gaining attention include:
- Quantum Computing: This technology aims to perform calculations that classical computers cannot easily handle. By exploiting the principles of quantum mechanics, these computers could revolutionize industries like cryptography and drug discovery.
- Nanotechnology: Physics plays a significant role in the development of materials at the nanoscale. This opens up avenues for better drug delivery systems, energy storage solutions, and more efficient solar cells.
- Renewable Energy Technologies: Advancements in fields like solar energy, wind energy, and battery technology rely heavily on physical principles. Understanding these can contribute significantly to sustainable solutions for our energy needs.
- Particle Physics Experiments: Facilities like CERN are fundamental to discovering new particles or forces in the universe, potentially leading us to understand unexplained phenomena such as dark matter.
Emerging technologies not only advance scientific research but also bridge gaps between theory and practical application, highlighting the essential role of physics in future innovations.
Contributions from Underrepresented Fields
It is undeniable that contributions from underrepresented fields enrich physics research and expand its horizons. Areas that may have been previously overlooked are gaining traction, leading to diverse perspectives and enhanced creativity. Notable aspects include:
- Interdisciplinary Approaches: Merging knowledge from fields such as biology and environmental science with physics creates new research opportunities. For example, biophysics explores biological systems through physical principles, thereby aiding developments in medical technologies.
- Feminist Physics and Gender Studies: An emphasis on gender diversity encourages the examination of how social factors influence physics research and education. This empowers new generations of physicists and fosters an inclusive atmosphere.
- Global Perspectives: Researchers worldwide contribute unique insights, particularly from regions facing distinct challenges. Engaging with these perspectives enriches our understanding of physics and stimulates global collaboration.
By integrating contributions from these underrepresented fields, the future of physics becomes more inclusive and innovative. This leads to revealing new theories and methodologies that could reshape our comprehension of the universe.
As we look toward the future, the fusion of emerging technologies and diverse perspectives in physics research is essential. Together, they will drive progress, inspire new avenues of inquiry, and refine our understanding of the cosmos.
End
In this article, we have navigated the intricate landscape of physics, examining key principles that form the foundation of this scientific field. The Conclusion serves as a pivotal segment, tying together the various threads discussed in earlier sections. Its importance lies in providing readers with a clear understanding of essential concepts and the broader implications of physics in everyday situations.
The summary of key concepts discussed thus far highlights the interconnectedness of different topics. For instance, understanding laws of motion enhance one's grasp of forces and energy principles. This synthesis ensures that each reader can build upon their knowledge progressively. It not only consolidates their learning but also reinforces the relevance of physics in diverse applications.
Summary of Key Concepts
Throughout the article, we explored several fundamental topics:
- Classical Mechanics: Understanding motion, forces, and their interactions.
- Energy Concepts: Differentiating between kinetic and potential energy, reinforcing the law of conservation of energy.
- Waves and Sound: Grasping the basic nature of waves and sound characteristics.
- Thermodynamics: Discussing the laws governing energy transfer and its practical applications.
- Modern Physics: Introducing concepts like relativity and quantum mechanics.
Each of these sections contributes to a greater overall understanding of how physics explains the natural world. The narrative connects theoretical frameworks to tangible experiences, making it relatable and engaging.
Encouragement for Further Learning
To truly appreciate physics, ongoing exploration is vital. Readers are encouraged to delve deeper into specialized subjects that piqued their interest. Resources such as Wikipedia and Britannica provide substantial information for those eager to expand their understanding.
Joining communities on platforms like Reddit can also elevate learning experiences. Discussing topics with peers fosters a deeper comprehension and can lead to insightful discoveries. Moreover, engaging with modern teaching techniques and resources can facilitate better learning outcomes.
In summary, the significance of physics extends beyond the classroom and into real-world applications. It empowers individuals by enhancing critical thinking skills and problem-solving capabilities. Continuous learning and curiosity are keys to unlocking the vast universe of physics. Embrace the journey, as it enhances intellectual growth and understanding of the world around us.