Understanding the Dark Nature of Black Holes

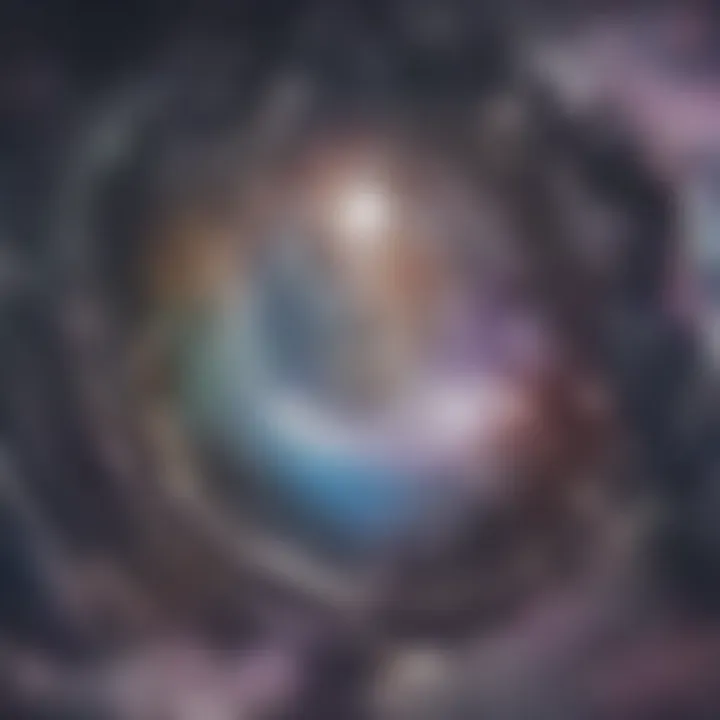
Intro
Black holes represent one of the most fascinating subjects in astrophysics. Their reputation as voracious cosmic entities has captured imaginations and stirred scientific inquiry for decades. Yet, the deeper one probes, the more complex the narrative of black holes becomes. Understanding why they appear black is just the beginning. To grasp their existence fully, we must delve into the interplay between gravity, light, and spacetime itself.
Despite their elusive nature, recent research has shed light on their formation processes and observable effects on surrounding matter.
This article seeks to unpack these concepts. The journey will navigate through key research findings, breakdown complex theories, and explore the significant implications these phenomena have on modern science.
Key Research Findings
Overview of Recent Discoveries
In recent years, significant advancements have been made in black hole research. The Event Horizon Telescope, for instance, enabled scientists to capture the first image of a black hole located in the center of the galaxy M87. This groundbreaking achievement not only confirmed theoretical predictions but also underscored the existence of supermassive black holes at the hearts of most galaxies.
Another pivotal discovery is the observation of gravitational waves, which emanate from black hole mergers. These waves provide a new means of studying black holes and the dynamics of their interactions.
Significance of Findings in the Field
The implications of these discoveries are profound. They not only strengthen the framework of general relativity but also open doors for new theories. The existence of black holes challenges traditional notions of space and time. Understanding their properties helps scientists formulate questions regarding the origin of the universe and the nature of dark matter.
In addition, observing black holes offers insight into the complexity of cosmic evolution, enriching our comprehension of the hierarchy of structures in the universe.
Breakdown of Complex Concepts
Simplification of Advanced Theories
Black holes are often discussed in the context of Einstein's general theory of relativity. Although complex, the principles governing black holes may be distilled into more digestible elements.
- Event Horizon: The boundary around a black hole beyond which nothing can escape.
- Singularity: The point at the center of a black hole where gravity is infinite and space-time curves infinitely.
- Hawking Radiation: Theoretical radiation proposed by physicist Stephen Hawking suggesting that black holes can emit energy and potentially evaporate over time.
Visual Aids and Infographics
Understanding black holes can be enhanced through visual aids. Diagrams illustrating spacetime curvature around massive objects help clarify their structure. Infographics that depict the life cycle of stars leading to black hole formation can also be beneficial.
"The study of black holes will not only further our understanding of gravity but may also reveal the underpinnings of quantum mechanics."
Intro to Black Holes
The topic of black holes is not just a fascinating aspect of modern astrophysics; it serves as a crucial element in understanding the universe's structure and dynamics. Black holes challenge our perceptions of space, time, and gravity. They force us to reassess fundamental principles in physics and metaphysics. As we dive into this topic, it is important to grasp the vast implications of black holes not only in scientific exploration but also in philosophical discourse.
Understanding black holes encourages critical thinking. They present a paradox where conventional wisdom often falls short. By studying these cosmic enigmas, researchers can unlock secrets of the universe, including the life cycle of stars and the nature of gravitational forces. The importance of this section lies in establishing a framework for readers to appreciate the complexity around black holes, setting the stage for deeper discussions.
Defining a Black Hole
A black hole can be defined as a region in space where the gravitational pull is so strong that nothing can escape from it, not even light. This characteristic leads to their defining featureβtheir invisibility. The boundary around a black hole is known as the event horizon, beyond which anything is irretrievably lost. The existence of black holes is rooted in the solutions of Einstein's field equations from the theory of General Relativity. These solutions describe how mass and energy warp spacetime, creating the conditions for black holes to form.
There are several types of black holes, including stellar black holes, supermassive black holes, and primordial black holes. Each type varies in mass and formation process. Stellar black holes occur when massive stars undergo gravitational collapse at the end of their life cycles, while supermassive black holes reside at the centers of galaxies, with masses that can exceed millions of solar masses.
Historical Context
The historical exploration of black holes dates back to the 18th century, although the term βblack holeβ itself was coined in the 1960s. Early ideas about such cosmic phenomena can be attributed to thinkers like John Michell, who speculated about βdark starsβ that could trap light. However, it wasnβt until Albert Einsteinβs theory of General Relativity was established in 1915 that a mathematical foundation for understanding black holes emerged. John Wheeler, an influential physicist in the mid-20th century, popularized the term as scientific understanding progressed.
The notion of black holes was met with skepticism for decades. It faced significant challenges until technology developed, allowing for observations that supported their existence. The first indirect evidence of a black hole came with the discovery of cyclic X-ray sources and later through gravitational waves detected from merging black holes. Such advancements illustrate how our understanding has evolved, marking significant milestones in astrophysical research.
Studying these historical developments enhances appreciation for the journey of scientific inquiry leading to contemporary understandings of black holes. This history signals an ongoing dialogue between theoretical prediction and observational validation, which remains at the core of astrophysical pursuits.
The Nature of Light
The nature of light is fundamental to understanding black holes. Light, in its various forms, serves as a primary medium through which we perceive the cosmos. It influences not only our visual experience but also the mechanics of astrophysical phenomena. In the context of black holes, examining light's properties reveals critical insights into why these entities are perceived as voids in space. Furthermore, studying light helps us comprehend the relationship between energy, gravity, and matter.
Properties of Light
Light is a form of electromagnetic radiation, and it travels in waves. One of its defining characteristics is speed; in a vacuum, it moves at approximately 299,792 kilometers per second. The frequency and wavelength of light determine its color and energy levels. For example, ultraviolet light has a shorter wavelength than visible light and carries more energy.
Concrete aspects of light properties are:
- Wave-Particle Duality: Light exhibits both wave-like and particle-like properties. This dual nature leads to phenomena like interference and diffraction.
- Reflection and Refraction: Light changes direction when it bounces off surfaces or passes through different media, which is essential in various technologies.
- Spectrum: The electromagnetic spectrum includes visible light, infrared, ultraviolet, and more. Each section has different applications in science and technology.
These properties are not just abstract concepts; they play a crucial role in our understanding of black holes. When light interacts with extreme gravitational fields, its behavior changes fundamentally.
Light and Visibility
Light is essential for visibility, allowing us to detect and interpret our environment. In the case of black holes, however, visibility is paradoxical. Black holes do not emit light, making them seemingly invisible to the naked eye. This absence presents challenges in observing them directly.
Factors affecting visibility include:
- Emission of Light: Black holes themselves do not radiate any light; instead, they affect nearby matter in ways that can produce bright emissions.
- Accretion Disks: Material falling into a black hole often forms an accretion disk, heating up and producing X-rays before crossing the event horizon.
- Gravitational Lensing: Light from stars and galaxies behind a black hole may be distorted, creating a lensing effect. This allows scientists to infer a black hole's presence indirectly.
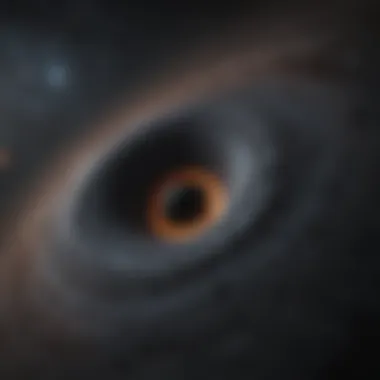
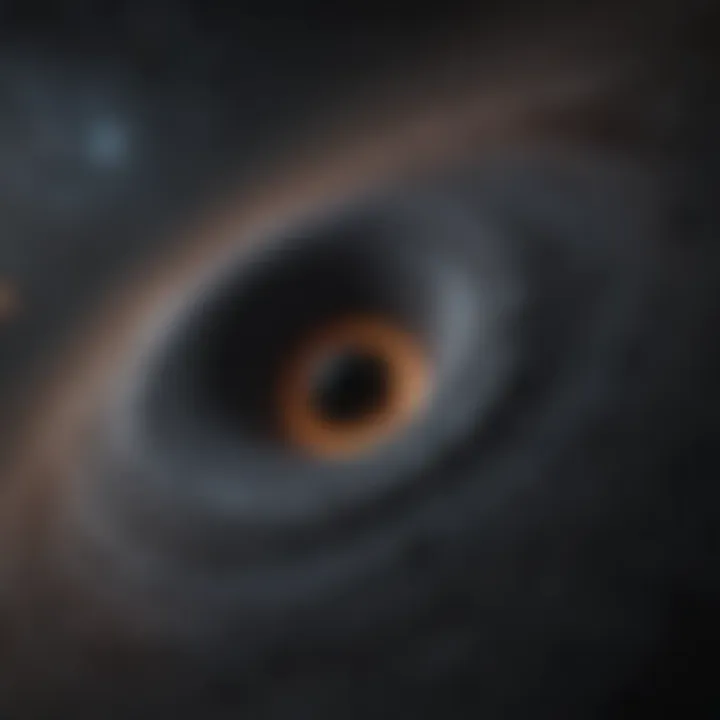
"Black holes are not black due to lack of light but rather due to their immense gravitational pull, which prevents light from escaping."
Gravity and Its Role
Gravity serves as a central pillar in the understanding of black holes, deeply influencing their formation, behavior, and interaction with the universe. It is more than just a force; it is a fundamental component of the fabric of spacetime itself. Without gravity, the phenomenon of black holes would not exist, as they are the ultimate expression of gravitational collapse. Examining gravity's function reveals not only how black holes form but also their effects on surrounding matter and light. This exploration is crucial for comprehending the larger cosmic environment.
The Concept of Gravitational Pull
Gravitational pull is a fundamental force that governs the motion of celestial bodies. According to Newtonβs law of universal gravitation, every mass attracts every other mass in the universe, with a force proportional to the product of their masses and inversely proportional to the square of the distance between their centers. This simple yet profound idea underpins the behavior of planets, stars, and indeed, the creation of black holes.
As massive stars exhaust their nuclear fuel, they can no longer support their mass against the relentless pull of gravity. This imbalance leads to a catastrophic collapse, where gravitational forces dominate, ultimately forming a black hole. In this stage, gravityβs influence intensifies dramatically, leading to a singularity, a point of infinite density.
Understanding gravitational pull is essential for grasping how black holes interact with their environments, especially in terms of accretion processes. The accretion disk surrounding a black hole consists of gas and dust spiraling inward, all of which are governed by the gravitational forces at play.
How Gravity Affects Light
Gravity's influence extends beyond matter; it also profoundly affects light. Light, which travels at a finite speed, is not immune to gravitational forces. According to general relativity proposed by Albert Einstein, massive objects warp the fabric of spacetime around them. This curvature leads to gravitational lensing, where light bends around massive bodies.
In the context of black holes, light emitted from nearby stars can be bent and influenced by the intense gravitational field. If light crosses the event horizon, it cannot escape. This is what gives black holes their characteristic darkness.
- Gravitational Redshift: As light climbs out of a gravitational well, it loses energy, causing it to redshift. This effect has important implications for how we observe light near black holes.
- Photon Sphere: Above the event horizon, there exists a region where gravity is strong enough to bend light into orbit around the black hole. This photon sphere is crucial for the understanding of how light interacts with black holes.
"In the vicinity of a black hole, light behaves in ways that challenge our conventional notions of visibility and distance."
In summary, gravityβs role in black holes extends far beyond mere attraction. It not only facilitates their formation but also dictates the pathways and behaviors of light, emphasizing the complex interplay between gravitation and light that defines these cosmic enigmas.
Formation of Black Holes
The topic of black hole formation is crucial in understanding the broader aspects of these cosmic entities. Knowing how black holes form can help elucidate their characteristics and influence in the universe. This section will delve into stellar evolution and the complex processes leading to the formation of black holes. It will explore how different types of stars contribute to these enigmatic structures, and the physical phenomena involved, laying the groundwork for comprehending the dynamics surrounding black holes.
Stellar Evolution
Stellar evolution is the life cycle of stars, including their formation, development, and demise. Every star, including our Sun, evolves through various stages influenced by its mass, temperature, and chemical composition. The journey of a star from formation to its end is significant in understanding how black holes come into existence.
In the sequence of stellar evolution, stars undergo nuclear fusion. During this process, hydrogen atoms fuse to form helium, releasing energy in the form of light and heat. As stars exhaust their hydrogen, they begin to burn helium and other heavy elements. This phase is followed by phases of expansion and subsequent contraction, eventually leading to dramatic end states.
Massive stars experience relatively rapid evolution. After burning through their nuclear fuel, they can no longer balance gravitational collapse with outward pressure from fusion. As gravity takes over, it compresses the star's core, leading to a supernova explosion. This explosion can either leave behind a neutron star or, in the case of very massive stars, prepares the star to collapse into a black hole.
"The path a star takes in its life ultimately determines if it will become a black hole."
Collapse of Massive Stars
The collapse of massive stars is a critical process that leads directly to the formation of black holes. This collapse occurs when a star, having exhausted its nuclear fuel, can no longer support itself against its own gravitational pull. For massive stars, this process is especially violent.
As the core collapses, temperatures soar, and densities increase exponentially. If the original star is sufficiently massive, the core's mass may exceed the Tolman-Oppenheimer-Volkoff limit, which is about 2 to 3 solar masses. At this point, whatever support mechanisms exist (like neutron degeneracy pressure) fail to halt the collapse. The result is a singularity, a point of infinite density, surrounded by the event horizon.
The surrounding material that does not fall into the black hole often gets expelled in a brilliant flash of energy during a supernova. This exhalation can outshine entire galaxies for a brief moment, highlighting the dramatic nature of stellar demise. The collapse into a black hole is thus one of the universe's most profound transformations, reshaping matter and energy.
In summary, understanding the formation of black holes through stellar evolution and the collapse phase provides insights into their nature and how they influence cosmic structures. This knowledge is fundamental for researchers, educators, and students interested in astrophysics, as it connects the lifecycle of stars to the mysterious existence of black holes.
The Event Horizon
The event horizon plays a crucial role in the study of black holes. It is often regarded as the boundary surrounding a black hole, beyond which nothing can escape, not even light. This boundary is fundamental to understanding the extreme nature of black holes and their interaction with light and matter. The properties of the event horizon significantly influence our perception of black holes as regions of darkness in space.
When one considers the event horizon, a few key elements emerge:
- Definition: It is essentially the point of no return for any object approaching a black hole. Once crossed, escape becomes impossible.
- Significance in Physics: The event horizon represents a canceptual threshold within the framework of general relativity, illustrating the profound impact that intense gravitational fields have on spacetime.
- Observational Challenges: Due to its nature, the event horizon cannot be observed directly. Instead, its presence is inferred from the behavior of matter and radiation near the black hole.
Thus, the event horizon not only serves as a defining feature of black holes, but it also raises important considerations for scientific study and theory validation.
Understanding the Boundary
The event horizon can be viewed as a geometric surface in spacetime rather than a physical barrier. In simpler terms, it does not represent a solid boundary but rather a point beyond which the known laws of physics start to behave differently.
Several aspects are important to understand about this boundary:
- Schwarzschild Radius: For non-rotating black holes, the event horizon is defined by the Schwarzschild radius. This radius correlates directly with the mass of the black hole, indicating the exact point at which escape velocity equals the speed of light.
- Non-Perception: Any observer at a safe distance will never witness an object crossing this boundary due to the infinite time dilation experienced as one approaches the event horizon. This phenomenon solidifies the enigmatic quality associated with black holes.
- Formation and Stability: The characteristics of the event horizon are determined during the formation of the black hole. During this process, instability may lead to changes in its size or shape, depending on the surrounding environment.
The boundary of the event horizon represents both a limit and an enigma, pushing the boundaries of current scientific inquiry.
Implications for Light
The event horizon has profound implications for light and how it behaves in the vicinity of a black hole. Since the event horizon prevents light from escaping, it creates a region where light behaves unpredictably.
The following points highlight critical considerations:
- Redshift Effect: Light emitted from an object near the event horizon is significantly redshifted due to the intense gravitational field. As the light struggles to escape, it loses energy and shifts toward longer wavelengths, making it increasingly difficult to detect.
- Photon Sphere: Just outside the event horizon lies a region called the photon sphere, where gravity is strong enough that photons are compelled to travel in circular orbits. This creates areas of light concentration, which can assist in indirect observations of black holes.
- Gravitational Lensing: Light passing near the event horizon is bent due to gravitational lensing, impacting how we perceive distant celestial objects. This distortion allows astronomers to infer the presence of black holes by observing the light from nearby stars.
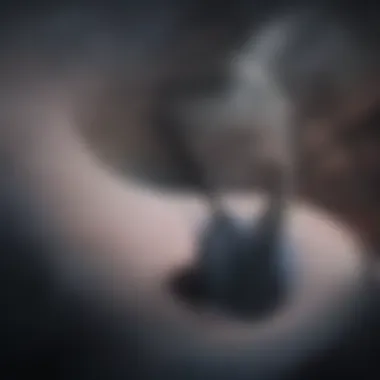
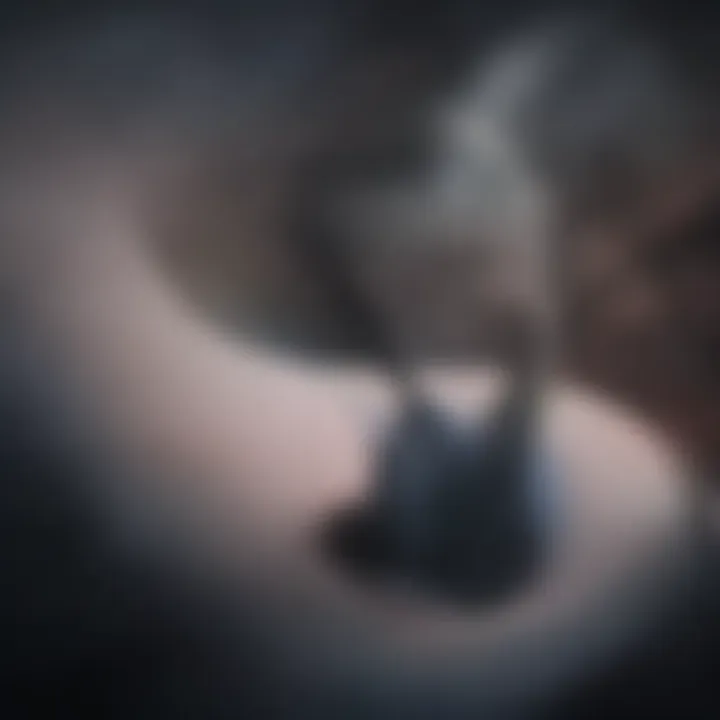
In essence, the event horizon is not merely a physical boundary, but represents the point at which our understanding of light, gravity, and the fabric of spacetime is put to the test.
Why Are Black Holes Perceived as Black?
Black holes hold a unique place in astrophysics, primarily due to their distinctive characteristic of being perceived as completely black. This perception is not merely a visual phenomenon but is rooted in fundamental principles of physics. Understanding why black holes are seen this way is crucial for comprehending their nature and behavior in the universe. The following sections will delve deeper into the reasons, focusing on the absence of light emission and light trapping mechanisms.
Absence of Light Emission
One of the primary reasons black holes appear black is the lack of light emission from them. Unlike stars or other celestial bodies that radiate light, black holes do not emit light due to their immense gravitational forces. They absorb all incoming light, preventing it from escaping their event horizon, which is the boundary around a black hole beyond which no information or matter can return.
This absence of light creates a void in the surrounding space. The specific interplay between gravity and light is essential. When light encounters the strong gravitational pull of a black hole, it is not merely affected β it is drawn in, lost to the observer. Therefore, in the vacuum of space surrounding a black hole, there is no light to reflect back towards us, giving black holes their dark appearance.
The interplay between gravity and light is fundamental in understanding why black holes are perceived as black.
Light Trapping Mechanisms
Light trapping mechanisms further explain how black holes maintain their dark visage. When any form of light approaches a black hole, the extreme gravitational field causes the light to curve towards the black hole rather than scatter away. This phenomenon is a direct implication of Einstein's general relativity, which describes how massive objects can warp spacetime.
When light passes too close to a black hole, it reaches a point of no return known as the photon sphere. Here, the gravitational pull is so strong that photons β the fundamental particles of light β are unable to escape. Instead, they spiral into the black hole, contributing to its invisibility. This inability for light to escape leads observers to perceive a region of space where no light exists.
Moreover, black holes can also trap other forms of energy. The accretion disks around black holes, which consist of matter spiraling in, can emit X-rays and other high-energy radiation. However, this radiation does not reflect the black hole itself but the transforming matter around it. Thus, the black hole retains its dark essence, while its environment might illuminate due to falling matter.
In summary, the perception of black holes as black arises from their complete absorption of light and the complex interactions between light and gravity. This knowledge is not just a facet of astrophysics; it challenges our fundamental understanding of light and existence in the universe.
Key Takeaways:
- The lack of light emission makes black holes appear dark.
- Gravitational forces trap light, creating a βblackβ region in spacetime.
- Light and energy interactions with black holes shape their observable characteristics.
Observing Black Holes
Observing black holes presents a unique challenge in contemporary astrophysical research. Fundamental to this investigation is the understanding that black holes do not emit visible light, making direct observation practically impossible. Instead, scientists rely heavily on indirect methods to identify and study these enigmatic entities. The importance of observing black holes extends beyond mere curiosity; it is essential for grasping their role in the cosmos, their formation processes, and their impact on surrounding matter. Moreover, studying them aids in exploring the fundamental laws of physics, particularly in the realm of theoretical frameworks like Einsteinβs general relativity and quantum mechanics.
Indirect Detection Methods
Indirect detection methods are pivotal when it comes to observing black holes. These techniques enable astronomers to infer the presence of a black hole based on the behavior of nearby matter and radiation. Thus, when black holes interact with companion stars or infall material, their effects become detectable. Some common indirect methods include:
- Accretion Disks: Black holes often attract surrounding gas and dust, forming a disk-like structure around them. This material heats up due to friction and emits radiation, often in the X-ray spectrum, which can be detected by specialized instruments.
- Gravitational Influence: By studying the motion of stars in binary systems, astronomers can determine the mass and location of an unseen black hole based on its gravitational effects.
- Gravitational Waves: The merger of two black holes generates ripples in spacetime, known as gravitational waves. Detectors like LIGO can measure these waves, confirming the events of black hole collisions.
Radio Waves and X-ray Emissions
The detection of radio waves and X-ray emissions is vital for understanding black holes. When matter interacts with a black hole, it can emit various forms of radiation. X-ray emissions are particularly telling, as they arise from the extreme temperatures in an accretion disk. For instance, as matter spirals into a black hole, friction causes the disk to heat up, resulting in X-ray emissions detectable by satellite observatories.
Radio waves can also provide insight. Some black holes, especially supermassive ones at the centers of galaxies, produce jets of charged particles that travel at nearly the speed of light. These jets emit significant radio waves, allowing scientists to trace their origin back to the black hole itself.
Theories Associated with Black Holes
The study of black holes is shaped by various theoretical frameworks. These frameworks are crucial for comprehending the complex nature of black holes and their place in modern physics. Understanding these theories offers insights into gravity, the behavior of light, and the nature of spacetime. Among the significant theories associated with black holes are Einstein's General Relativity and the principles of Quantum Mechanics.
By exploring these theories, we can appreciate the interplay between large-scale cosmic events and quantum phenomena. Each theory provides a unique perspective, allowing scientists to refine models and predictions about black holes.
Einsteinβs General Relativity
Einsteinβs General Relativity is perhaps the most influential theory regarding black holes. Proposed in 1915, this theory reformulated our understanding of gravity. Instead of viewing gravity as a force between masses, it depicts gravity as the curvature of spacetime caused by mass. Black holes, in this framework, are regions where this curvature becomes so intense that not even light can escape.
- Fundamental Concepts: General Relativity explains how massive objects like stars curve the space around them, influencing the paths of nearby objects and light. This curvature leads to concepts such as gravitational time dilation, where time flows slower in strong gravitational fields.
- Event Horizon: According to General Relativity, the boundary surrounding a black hole is known as the event horizon. Beyond this point, the escape velocity exceeds the speed of light. This concept is essential for understanding why we cannot observe what lies inside a black hole.
- Gravitational Waves: The theory also led to the prediction of gravitational waves, ripples in spacetime caused by the acceleration of massive objects. Their detection has been instrumental in confirming aspects of General Relativity and providing insights into black hole mergers.
Quantum Mechanics and Black Holes
Quantum Mechanics introduces another layer of complexity to our understanding of black holes. While General Relativity governs large-scale cosmic phenomena, Quantum Mechanics deals with subatomic particles and the fundamental forces of nature.
- Black Hole Entropy: One of the most compelling intersections is the concept of black hole entropy, proposed by Stephen Hawking. Hawking theorized that black holes possess entropy and can emit radiation, known as Hawking radiation. This finding implies black holes are not entirely black and can eventually evaporate over incredibly long timescales.
- Information Paradox: The interplay between Quantum Mechanics and black holes also brings forth the information paradox. This paradox questions whether information that falls into a black hole is lost forever or if it can be recovered, challenging our understanding of quantum theory and information conservation.
- Unified Theories: The search for a unified theory of Quantum Gravity aims to merge General Relativity and Quantum Mechanics into a cohesive framework. Finding a solution could revolutionize our understanding of black holes and the fundamental laws of the universe.
"Theories of black holes are at the forefront of modern physics, bridging the gap between our understanding of gravity and quantum mechanics."
The exploration of these theories holds great significance. It shapes the future research and understanding of black holes, offering avenues for new discoveries that could underpin our understanding of the universe.
Black Holes and Cosmology
The study of black holes has profound implications for cosmology, fundamentally reshaping our understanding of the universe. Black holes are not merely cosmic oddities but pivotal components in the cosmic framework that governs structure, evolution, and destiny of the universe itself. Their immense gravitational influence extends beyond their immediate vicinity, dictating the movement and arrangement of stars, galaxies, and clusters.
In this section, we will explore how black holes contribute to the universe's structure. We will also discuss the fate of matter as it interacts with these enigmatic entities. Understanding these concepts yields insights into cosmic phenomena, such as galaxy formation and dark matter, and may unlock secrets about the universe's future.
Role in the Universe's Structure
Black holes play a crucial role in shaping the structure of the universe. They are often situated at the centers of galaxies, including our Milky Way, where they can exert tremendous gravitational force. This force can help hold stars and other celestial objects in orbit, thereby maintaining the cohesion of galactic structures.
Research suggests that supermassive black holes exist in most large galaxies, with masses millions to billions of times greater than that of the Sun. Their presence influences star formation, galaxy mergers, and the overall dynamics of galaxies. Essentially, the gravitational pull of black holes helps regulate the flow of matter within galaxies and contributes to the large-scale structure of the cosmos.
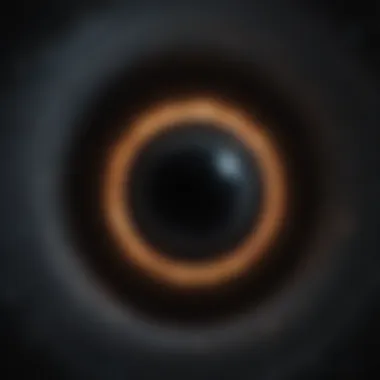
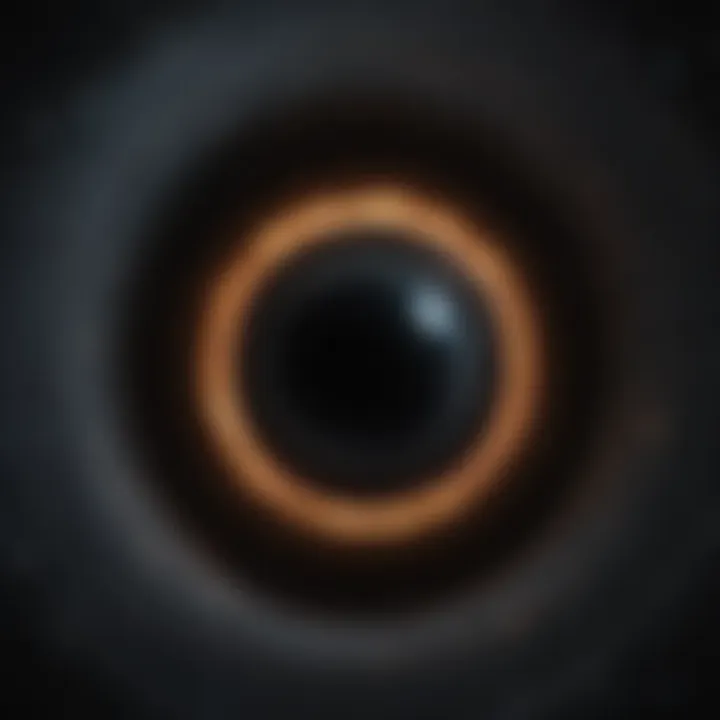
"Supermassive black holes connect the local to the cosmic scale, revealing the intricate dance of galaxies and the evolution of cosmic filaments."
Some of the notable aspects of their role in cosmic structure include:
- Galactic Dynamics: Black holes aid in stabilizing galaxies, influencing rotational speeds and interactions with neighboring galaxies.
- Star Formation: They can either inhibit or enhance star formation through their gravitational influence, shaping the lifecycle of galactic elements.
- Accretion Disks: Matter falling into black holes forms an accretion disk, heating and radiating energy, which can lead to the formation of new stars.
The Fate of Matter
The fate of matter in the presence of black holes is one of the most intriguing aspects of astrophysics. As matter approaches a black hole, it undergoes intense gravitational forces, leading to various physical phenomena, some of which are still not fully understood. The ultimate destination of this matter remains a subject of great debate among physicists.
When matter crosses the event horizon of a black hole, it becomes irretrievably bound. This leads to questions about what happens to the information contained within that matter. According to the laws of quantum mechanics, information cannot be destroyed. This has led to theories such as the holographic principle, suggesting that information about matter may be preserved on the event horizon.
Black holes may also act as a recycling mechanism for matter within the cosmos. Matter can be pulled in, compressed, and potentially expelled back into the universe in various forms, such as through jets emitted during the accretion process.
Important considerations include:
- Singularity: The point where matter is infinitely dense, and current physical laws break down. What happens at the singularity? This question remains largely unanswered.
- Hawking Radiation: Proposed by Stephen Hawking, this theoretical radiation might eventually lead to black holes losing mass and ultimately evaporating over astronomical timescales.
- Cosmic Rebirth: Matter consumed by black holes may contribute to the birth of new stars and potentially new galaxies elsewhere in the universe.
In summary, black holes are integral to cosmological understanding. Their role in the universe's structure and the fate of matter provides critical insights into fundamental questions about the cosmos. The ongoing research in this field promises to unravel many mysteries, shaping our understanding of the universe in unprecedented ways.
Current Research and Discoveries
Current research into black holes is essential for understanding their complex nature and impact on the universe. Ongoing studies provide vital insights into the physics of these celestial entities, revealing their influence on galactic formations as well as on fundamental theories of physics.
Recent Imaging of Black Holes
Recent advances in imaging technology have transformed how scientists perceive black holes. The Event Horizon Telescope (EHT) project, which successfully captured the first direct image of a black hole in 2019, marked a monumental achievement in astrophysics. This black hole, located at the center of the M87 galaxy, was visualized as a shadow surrounded by a ring of light.
The imaging process involved coordinating multiple radio observatories around the world to act as a planet-sized telescope. This collaboration allowed for unprecedented resolution, enabling astrophysicists to study the black hole's characteristics more closely. The implications of this work are profound. It not only confirms previous theories of black hole existence, but also opens new avenues for research in understanding the surrounding accretion disk and relativistic jets. With technology continuously evolving, further high-resolution images of black holes can refine our grasp of their behavioral patterns, magnetic fields, and the influence of extreme gravity.
Future Predictions and Studies
The future of black hole research holds exciting possibilities. As new technologies develop, scientists anticipate uncovering more about the dynamics of black holes. One area of focus is gravitational waves, ripples in spacetime caused by the collision and merger of black holes. The Laser Interferometer Gravitational-Wave Observatory (LIGO) has already detected multiple events, providing a new method to study black holes that cannot be observed through traditional means.
Another promising direction is the potential for gravitational wave astronomy to reveal the properties of black holes' population in the universe. This might enhance understanding of their formation and evolution. Furthermore, researchers are examining the implications of black holes for quantum mechanics, attempting to bridge concepts of gravity with quantum theory. Such studies could reshape our understanding of the universe's fundamental fabric.
"The importance of current research into black holes cannot be overstated. It not only expands our knowledge but bridges gaps between established theories and new scientific questions."
In summary, both recent imaging and future studies are crucial for unpacking the dark nature of black holes. They contribute to a deeper grasp of cosmic phenomena and fundamentally challenge our understanding of physics.
Philosophical Implications
The study of black holes raises profound questions about the nature of reality and existence. Black holes are not just cosmic phenomena; they challenge our understanding of physics and the fundamental laws that govern the universe. Their existence forces scientists and philosophers alike to debate various concepts that shape our understanding of the cosmos.
One significant aspect of this philosophical discourse is the relationship between observation and reality. If black holes cannot be seen directly, how do we know they exist? This parallels discussions in epistemology about the limits of human knowledge and the invisible structures of reality. Intuitively grasping the concept of a black hole can feel unnatural, as it defies our everyday experience. Yet, the implications of black holes are not confined to astrophysics; they resonate across various fields, including metaphysics and even ethics.
Nature of Reality and Existence
The enigmatic nature of black holes prompts inquiries into what constitutes reality. Can something exist if it cannot be perceived with our senses? Black holes challenge our perceptions by having enormous mass and gravitational pull while remaining invisible. This paradox invites us to reconsider our definitions of existence and reality itself. It raises philosophical questions about the fabric of our universe and the importance of empirical evidence in validating existence.
Scholars often reflect on the idea of existence beyond human comprehension. For example, the existence of dark matter and dark energy rounds out our understanding of the universe, suggesting that there are aspects of reality we have yet to grasp fully. The absence of light and information from within a black hole may symbolize areas of ignorance in human knowledge, leading to discussions about the limits of science.
Black holes may be the universe's ultimate metaphor for the unknown, challenging our assumptions and expanding the frontier of human understanding.
Time and Space Considerations
Black holes influence our comprehension of time and space, often redefining those very concepts. According to Einstein's theory of general relativity, the extreme gravitational forces surrounding a black hole warp spacetime significantly. This curvature influences how time behaves as one approaches the event horizon. For an observer far away, time appears to slow down for objects nearing a black hole, creating fascinating dilemmas regarding simultaneity and the flow of time itself.
Furthermore, this affects our understanding of causality. If time stretches infinitely near a black hole, can events overlap? Such inquiries lead to reconsiderations of fate and destiny, suggesting that our reality operates under rules we are only beginning to understand. The possibility of time behaving differently in such extreme conditions pushes us toward an intellectual crossroads, raising the question of how these phenomena align with our current theories and philosophies.
Concluding Remarks
The concluding section of this article serves as an essential wrap-up, reinforcing the critical concepts discussed throughout. Black holes are not just fascinating astronomical entities; they challenge our understanding of physics, time, and existence itself. Summarizing the complexities of their nature encourages rational reflection and comprehension among students and enthusiasts alike. This section also propels consideration of future research avenues, emphasizing the ever-evolving landscape of astrophysics.
Among key takeaways, we explore the formation of black holes from massive stars and the intricate relationship between gravity, light, and space. These elements highlight the fundamental principles that govern not only black holes but also cosmic phenomena at large.
Additionally, the implications of black holes stretch beyond mere observation; they touch upon philosophical questions regarding reality and our place within the universe.
This verdict reiterates the expansive significance of black holes in modern science. Their study not only enhances comprehension of the universe's architecture but propels advancements in technology and theoretical physics. For those invested in this field, recognizing the relevance of black holes is key to the pursuit of scientific knowledge.
Summary of Key Points
- Black holes result from the gravitational collapse of massive stars.
- The event horizon defines their boundary, beyond which light cannot escape.
- Black holes impact the structure of the universe and influence cosmic evolution.
- Future research aims to deepen our understanding of their existence and properties.
Future of Black Hole Research
Future research on black holes holds promise for monumental discoveries. The advent of advanced imaging technologies has already granted humanity a glimpse into the shadowy recesses of these phenomena. Scientists aim to further analyze the interactions between black holes and their surrounding environments, shedding light on how they shape galaxies.
Key areas of focus include:
- Gravitational Waves: Detecting ripples in spacetime could offer insights into the behaviors of black holes.
- Quantum Gravity: Understanding how quantum mechanics relates to black holes is essential for unifying physics.
- Black Hole Information Paradox: Researchers continue to explore how information is preserved in these enigmatic objects.
In summary, the exploration of black holes is a frontier of modern astrophysics. As we enhance our methodological approaches, we can expect groundbreaking revelations that have the potential to redefine our comprehension of the universe. Engaging with this field not only deepens our knowledge but also invigorates the challenges we face in understanding the nature of reality and existence.