Understanding the Complexities of Dark Matter Theory
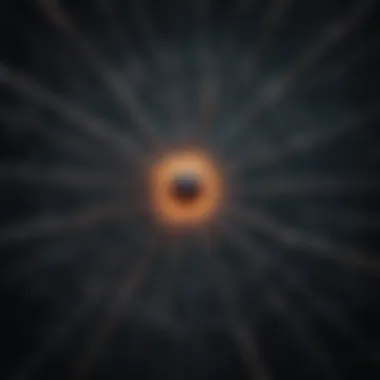
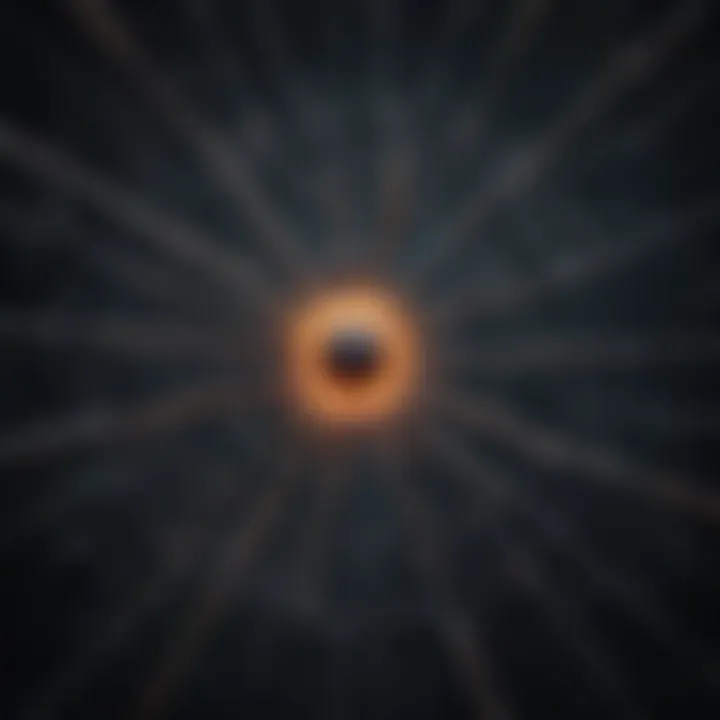
Intro
The notion of dark matter has been a source of intrigue and debate within the scientific community for decades. With visible matter making up only a small fraction of the cosmos, dark matter emerges as a hidden yet crucial player in the grand tapestry of the universe. It is much like a shadow that bends the light, a presence felt but hardly seen. As we embark on this exploration, let's take stock of where we stand in our understanding of this elusive entity.
Key Research Findings
Overview of Recent Discoveries
Recent advancements in astrophysics have marked significant milestones in our quest to understand dark matter. One pivotal piece of evidence emerged from measurements of galaxy rotation curves, which consistently showed that visible matter alone cannot account for the speeds at which stars orbit galactic centers. The gravity of an invisible substance, thus, is inferred to be at play.
Furthermore, projects like the Atacama Cosmology Telescope and experiments at the Large Hadron Collider have been essential in testing various dark matter hypotheses. Among these, the Weakly Interacting Massive Particles (WIMPs) model has gained traction, though it still lacks direct detection. Recent studies using gravitational lensing techniques have also bolstered evidence of dark matter's influence on the structure of the universe, revealing how it shapes galaxies and clusters at massive scales.
Significance of Findings in the Field
The implications of these findings are profound. Understanding dark matter not only challenges our previous models of physics but also offers insights into the formation and eventual fate of the universe. The notion that 27% of the universe’s mass-energy content is inaccessible to direct observation means that our knowledge of the cosmos is only scratching the surface.
As researchers delve deeper into this mystery, they have found that dark matter may play a role in cosmic inflation, a period of rapid expansion following the Big Bang. The relationship between dark matter and the cosmic microwave background radiation further underscores its foundational significance in cosmology.
"Dark matter might very well reshape our understanding of the universe's structure and dynamics."
Breakdown of Complex Concepts
Simplification of Advanced Theories
When discussing dark matter, it’s paramount to simplify advanced theories for broader understanding. One way to approach this is through the lens of potential candidates for dark matter. The WIMP theory postulates particles that interact weakly with normal matter; in simpler terms, think of these as shy particles that rarely mingle with their visible counterparts.
Another perspective is the Modified Newtonian Dynamics (MOND), which attempts to explain phenomena traditionally attributed to dark matter by modifying the laws of gravity at small accelerations. This introduces an interesting debate on whether dark matter is necessary at all, suggesting we might not have the full picture.
Visual Aids and Infographics
To solidify understanding, visual aids are invaluable. Infographics showcasing the distribution of visible versus dark matter in galaxies can provide clarity. For instance, consider a pie chart that highlights how only 5% of the universe is composed of normal matter. This stark contrast can visually illustrate the dominance of dark matter, making abstract concepts more tangible.
Example of an infographic idea:
- Title: Distribution of Matter in the Universe
- 5%: Normal Matter
- 27%: Dark Matter
- 68%: Dark Energy
This simple representation leaves a lasting impression, reinforcing the enormity of dark matter’s significance.
As we further unravel the complexities of dark matter, it remains crucial to think critically about what lies beyond our current perceptions of the universe.
Prelims to Dark Matter
Dark matter is a term that often raises eyebrows and sparks curiosity among those who gaze into the cosmos. It's not just a technical detail for physicists; it's at the very core of our understanding of the universe's structure. Without grasping dark matter, one cannot truly appreciate the vastness and complexity of cosmic dynamics. This section serves as an entry point into the multifaceted world of dark matter, laying a foundation for deeper exploration.
Definition and Characteristics
Defining dark matter is, perhaps, like trying to grasp smoke with bare hands. At its essence, dark matter refers to a mysterious and invisible substance that exists in space, constituting approximately 27% of the universe. Unlike ordinary matter, which interacts via electromagnetic forces and can be seen in the form of stars, planets, and gas, dark matter does not emit, absorb, or reflect light, making it undetectable by traditional observational tools. Its existence is inferred primarily through its gravitational effects on visible matter, particularly in galaxies.
Some characteristics of dark matter include:
- Non-luminous: Dark matter does not emit light or radiation, making it invisible to all current detection methods.
- Weakly Interacting: It interacts very weakly with regular matter, making its detection an ongoing challenge.
- Clumpiness: Dark matter tends to cluster with itself, enhancing the gravitational wells that attract normal matter and forming structures like galaxies.
Moreover, various candidates have been proposed to explain what dark matter could actually be, from exotic particles to primordial black holes. Each potential candidate carries implications that extend beyond just dark matter itself, influencing our broader understanding of physics and cosmology.
Historical Context
To appreciate dark matter, one must journey back to the early decades of the 20th century. The notion that there is more to the universe than meets the eye didn't originate with modern technology. Take, for instance, the observations of Fritz Zwicky in the 1930s. While analyzing the motion of galaxies within the Coma cluster, he found that the visible mass was insufficient to account for the gravitational binding of the cluster. His conclusion was groundbreaking, proposing that an unseen mass was at play—a prediction that would resonate for decades.
However, it wasn't until the late 1970s, when Vera Rubin and her colleagues meticulously studied galaxy rotation curves, that dark matter gained significant traction within the scientific community. Rubin’s groundbreaking work demonstrated that stars at the outer edges of galaxies were orbiting much faster than expected, further corroborating the existence of this elusive substance.
Over the years, many scientists have contributed to the dark matter discourse, yet questions remain. It is regarded not just as a defect in existing theories but a compelling challenge that may usher in new paradigms in our quest to understand the cosmos. The historical progression of dark matter theory reflects humanity's persistent drive to look beyond the obvious, inviting deeper questions about the nature of reality itself.
Observational Evidence for Dark Matter
The exploration of dark matter is underpinned by a multitude of observational evidence that showcases its elusive presence in the universe. This section focuses on the pivotal contributions of observational findings, helping to fortify the theoretical frameworks that swirl around this enigmatic substance. Evidence for dark matter isn't merely academic; it shapes our understanding of cosmic structures and the life cycle of galaxies.
Each piece of evidence slices through various theories, offering a fascinating narrative about how the cosmos behaves. The tools of astronomy have unearthed numerous mysteries, and the far-reaching implications of these observations highlight the very fabric of the universe we live in.
Galaxy Rotation Curves
To wrap your head around galaxy rotation curves, consider how galaxies spin. Long ago, it was assumed that stars would orbit a galaxy's center similarly to planets around the sun, with their velocity decreasing as you moved further away from this core. Simple physics, right? But observational data tells a different story.
When astronomers began to measure the velocities of stars at varying distances from a galaxy's center, they found that those speeds remained surprisingly constant beyond the galaxy’s visible edge. This anomaly is telling us something significant: the presence of additional unseen mass, or dark matter, which holds the galaxies together.
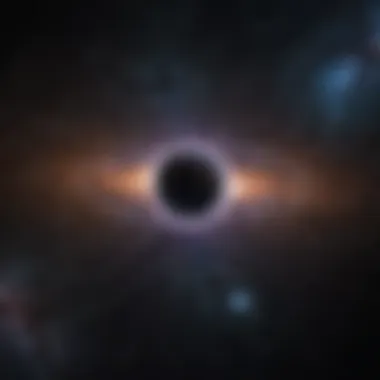
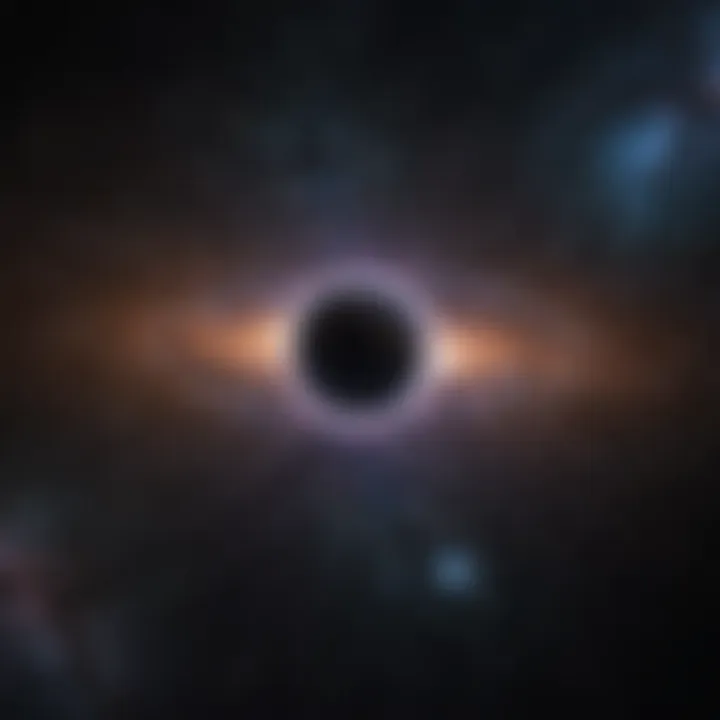
"The galaxy rotation curves hint that there’s much more lurking in the shadows than what we can see with our own eyes."
This aspect has led to the construction of dark matter halos around galaxies, indicating that it's not just the stars we observe that dictate gravitational behavior. Instead, dark matter shapes these cosmic dance floors.
Gravitational Lensing
Gravitational lensing opens another compelling chapter in the narrative of dark matter. According to Einstein’s theory of General Relativity, massive objects can bend light. When light from a distant galaxy passes near a massive object like a galaxy cluster, it can be distorted, creating arcs and multiple images of the same object.
This phenomenon gives scientists a unique view into the distribution of mass, both visible and hidden, in the lensing object. The observed distortion of light showcases areas of dark matter, which otherwise eludes direct detection.
Here’s the kicker: the total mass calculated from lensing often exceeds the mass visible through conventional means, suggesting that there’s unseen mass exerting a gravitational pull. Studies of clusters like the Bullet Cluster vividly illustrate this effect, reinforcing the existence of dark matter through the mismatched distribution of visible matter and gravitational mass.
Cosmic Microwave Background
The Cosmic Microwave Background (CMB) serves as a sort of cosmic wallpaper, behind which the story of dark matter unfolds. This faint glow, which fills the universe, is evidence of the Big Bang and serves to tell us how the cosmos has evolved over epochs. What’s truly fascinating is how the fluctuations in the CMB—tiny temperature differences—are closely linked to the density of matter in the early universe.
By examining these fluctuations, physicists can infer the ratio of normal (baryonic) matter to dark matter. The patterns observed in the CMB paint a clear picture: a significant portion of the universe's mass is non-baryonic and likely contextually dark. These insights feed into our understanding of cosmic inflation and structure formation, providing critical insight into the role dark matter plays in shaping our universe.
In summary, the observational evidence for dark matter is indispensable in both validating and refining our theories. Whether it’s the peculiar rotation of galaxies, the bending of light due to gravitational lensing, or the subtle imprints of the CMB, each lends credence to the idea that dark matter is a fundamental component of the cosmos, defining the architectural framework of our universe.
Theoretical Frameworks
Theories surrounding dark matter are central to comprehending its overarching role in the universe's architecture. These theoretical frameworks help to fill in the gaps where observational evidence sometimes falls short. They serve as a blueprint for understanding cosmic phenomena that challenge classic Newtonian physics, guiding researchers in both formulating hypotheses and designing experiments to hunt for dark matter.
Researchers primarily categorize dark matter theories into two vast umbrellas: modified gravity theories and particle physics models. Each framework brings its own insights and interpretations, allowing scientists to explore the universe's mysteries from different angles.
Modified Gravity Theories
Modified gravity theories propose alterations to Newton's laws or Einstein's general relativity to account for the effects traditionally attributed to dark matter. Rather than inventing new particles, these theories suggest that gravity's behavior is more complex than commonly thought.
One such example is MOND (Modified Newtonian Dynamics), which posits that gravity behaves differently under extremely low accelerations. Although MOND has had mixed success in explaining galaxy motions, it reveals the limitations of classical physics and pushes for innovative thinking on how forces interplay on cosmic scales.
Typically, modified gravity theories enjoy less popularity among researchers compared to particle models, primarily due to their inability to resolve phenomena across various scales effectively. Nonetheless, they encourage reevaluation of fundamental principles in physics, compelling the scientific community to reexamine our gravitational understanding.
Particle Physics Models
Particle physics models attempt to explain dark matter as a collection of particles that interact minimally with ordinary matter. These models provide potential candidates that could be detected through ongoing experiments. Three of the most discussed particle candidates are supersymmetry, axions, and WIMPs.
Supersymmetry
Supersymmetry is a theoretical framework that posits a partner particle for every known particle. This duality would not only stabilize the mass of the Higgs boson but could also account for dark matter through its lightest supersymmetric particle, usually referred to as the neutralino.
A key characteristic of supersymmetry is its elegant solution to issues surrounding unification of forces at higher energies. This aspect makes it a popular model among physicists, especially because it emerges naturally in string theory contexts. Currently, experiments like those at the Large Hadron Collider are on the hunt for diffraction patterns that could hint at supersymmetric particles.
However, there's an uphill battle: the energy scales required to produce supersymmetry are considerably high, making it tricky to observe in current experiments. Additionally, as of now, there’s been no conclusive evidence of its existence, raising skepticism within part of the scientific community.
Axions
Axions are hypothetical particles that arise from theoretical frameworks aiming to solve QCD (Quantum Chromodynamics) issues. They are incredibly light and weakly interacting. Their introduction offers an appealing solution to the nature of dark matter, particularly because they could also account for the strong CP (Charge Parity) problem in particle physics.
The unique feature of axions is their potential for being detected through specific astrophysical signals, like those from neutron stars. Their lightweight nature makes them a likely candidate for contributing to the universe's energy density without overcrowding the particle zoo. This feature adds to why axions are a favored topic of discussion in dark matter circles.
However, a significant challenge with axions lies in their elusive nature. They interact so weakly with matter that detecting them is a tedious endeavor, requiring advanced technology and creative experimental techniques.
WIMPs
WIMPs, or Weakly Interacting Massive Particles, have gained traction as prime candidates for dark matter. They would predominantly interact through the weak nuclear force and gravity, making them difficult to detect, similar to axions. The attraction of WIMPs boils down to their capacity to naturally arise from supersymmetry paradigms, adding coherence to the wider particle physics narrative.
A remarkable characteristic of WIMPs is that their predicted abundance could align with measurements from cosmological surveys, suggesting a plausible match in terms of dark matter density. This correlation makes WIMPs a favorite among researchers, who speculatively await significant findings from ongoing experiments such as those conducted at underground facilities.
Still, as with other theoretical candidates, conclusive evidence for WIMPs remains elusive, leading researchers to explore alternative avenues in their search.
Implications of Dark Matter
Dark matter isn’t just a curious concept tossed around in scientific discourse; it plays a pivotal role in our understanding of the universe's structure and evolution. Its implications extend beyond mere theory, affecting how we comprehend the cosmos, its formation, and even our place within it. By exploring the crucial impacts of dark matter, we can gain better insight into various phenomena observed in the universe, ranging from galaxy formation to the very dynamics of cosmic expansion. The lack of direct visibility of dark matter challenges researchers to grasp its influence via indirect methods and theoretical modeling, making these implications even more significant and compelling.
Structure Formation
Small-Scale Structures
When we talk about small-scale structures, we dive deep into the realm of dwarf galaxies and globular clusters, which are often found woven into the fabric of larger galactic systems. The intricate dance of these small entities highlights a key characteristic: their gravitational binding, which often seems to defy expectations based on visible matter alone. The interplay with dark matter governs their formation and stability. As they constantly coalesce and interact, their dynamics are influenced heavily by the unseen forces of dark matter.
This feature is particularly beneficial for our understanding because it sheds light on how less massive systems formed in the early universe. The existence of dark matter provides a scaffolding that supports these small-scale structures, allowing researchers to formulate hypotheses regarding their development and cohesion.
However, a unique disadvantage lies in the difficulty of studying these structures. Many small-scale entities are faint and challenging to observe, meaning that our information is often limited, leading to uncertainties in models and predictions about their life cycles.
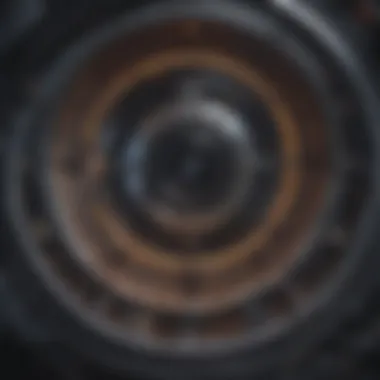
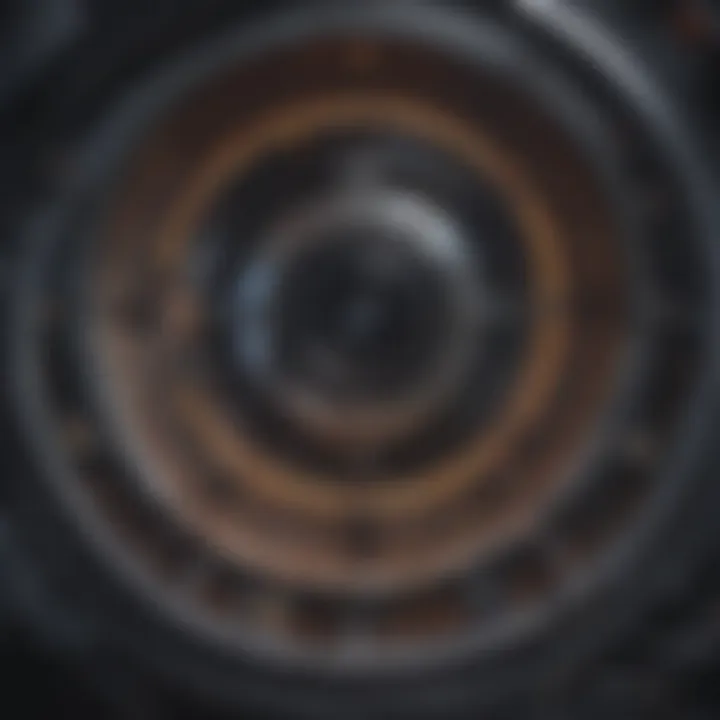
Large-Scale Structures
Switching gears to large-scale structures, we find ourselves in a landscape dominated by clusters of galaxies stretching across vast cosmic distances. These enormous conglomerations are the backbone of the universe, influenced profoundly by dark matter via its gravitational pull. Their presence helps explain why galaxies congregate in the ways they do, revealing a pattern that is fundamentally tied to dark matter’s distribution.
The compelling aspect of large-scale structures is their ability to reveal dark matter’s influence in a much clearer light compared to small-scale entities. By observing how galaxies cluster and the cosmic web delineates itself, we can infer dark matter's gravitational properties, which is why it’s considered a popular focus in dark matter studies.
A unique characteristic is how these formations show us the universe's age and expansion rate. Yet, the downside to this is that while they provide significant insights, they also depend heavily on the accuracy of our models and observations. Miscalculations in estimating distances or densities can lead to erroneous conclusions about the dark matter content within these structures.
Effects on Cosmic Evolution
Dark matter has an undeniable effect on cosmic evolution. From the inception of the universe to its far-reaching future, dark matter’s presence has guided the evolution of cosmic structures at different scales. Research suggests that without dark matter, the formation of galaxies would likely follow a different trajectory, one that may not result in the richness of structures we see today.
To encapsulate, the interplay between dark matter and various cosmic structures helps illuminate our understanding of not only how the universe evolved but also what we might expect in the eons to come. The ongoing investigation into dark matter ensures that we keep our understanding of the universe dynamic and evolving, always pushing the boundaries of human comprehension.
"The exploration of dark matter leads us to deeper questions about existence itself, challenging our perceptions and prompting us to look beyond the visible."
Ultimately, the implications of dark matter are vast and reach into both theoretical and observational realms, encouraging continuous study and inquiry.
Challenges in Dark Matter Research
Research into dark matter presents numerous hurdles that scientists must navigate. These challenges not only complicate the effort to understand this elusive substance but also shape the direction of contemporary cosmological studies. Engaging with these challenges holds not just academic importance but practical significance, as resolving the mysteries of dark matter could revolutionize our grasp of the universe.
Detection Challenges
The quest for dark matter is akin to looking for a needle in a haystack; it’s about finding that invisible thread in a cosmos teeming with visible matter. Detection challenges are paramount in this field, primarily because dark matter does not emit light or any form of detectable radiation. Researchers aim to identify its presence through its gravitational influences, but this approach has several complications:
- Sensitivity Limits: Current detection technologies struggle to discern the weak signals from dark matter interactions. Powerful detectors are required to pick up potential events, which often yield low data yield against high backgrounds.
- Background Interference: Various natural processes can mimic the signals researchers expect from dark matter particles, leading to false positives. For instance, cosmic rays can produce similar effects as those anticipated from dark matter interactions.
- Location of Experiments: Many detectors are installed deep underground to shield them from cosmic rays. While this helps, the need for extreme locations often limits access and increases costs.
Even with advancements in technology, the challenge remains of how to build more sensitive detectors that can improve data quality while filtering out the noise effectively.
Theoretical Controversies
The theoretical landscape surrounding dark matter is rife with debates and diverse viewpoints. This plethora of theories not only reflects the ongoing struggle to grasp what dark matter is but also reveals deeper philosophical and scientific questions.
- Existence of Dark Matter: Some scientists propose alternatives to dark matter, positing that modifications to General Relativity could account for observed phenomena. This raises the question—are we truly witnessing the effects of an unseen substance, or is it a reflection of our incomplete understanding of gravity?
- Nature of Dark Matter Particles: If dark matter exists, what is its composition? Various theoretical models propose entities like WIMPs or axions, yet no definitive answers have emerged. Each idea comes with its own implications for particle physics and might redefine established concepts.
- Experimental Validation: Even with a prominent theory in place, the pathway to experimental validation can be convoluted. The overlap of theoretical predictions with observational data often leads to disagreements among scientists about how to interpret findings.
"Understanding dark matter isn't just about knowing what it's made of, but also about questioning the frameworks we think we understand."
In summary, the challenges in dark matter research present a labyrinth of complexities that both hinder and fuel scientific inquiry. From difficulties in detection to rich theoretical debates, each aspect shapes the fabric of modern astrophysics and will guide the future directions of inquiry into one of the universe's greatest mysteries.
Current Experimental Efforts
Current experimental efforts play a pivotal role in our quest to understand dark matter. With the universe being predominantly composed of this elusive substance, scientists are continually innovating ways to uncover its secrets. This section dives into the different strategies used to detect dark matter, each bringing its own strengths and challenges to the table.
Direct Detection Experiments
Direct detection experiments aim to spot dark matter particles as they interact with ordinary matter. These experiments are crucial because they probe the fundamental properties of dark matter directly, providing vital clues to its nature.
Underground Detectors
Underground detectors are one of the most popular approaches to direct detection of dark matter. These setups are often located deep beneath the Earth’s surface to shield them from cosmic radiation that could interfere with the sensitive measurements they need to take. What stands out about underground detectors is their ability to minimize background noise, which is crucial for identifying the faint signals of dark matter interactions.
The primary distinguishing feature of underground detectors is their sheer volume and isolation. Systems like the LUX-ZEPLIN experiment utilize large volumes of liquid xenon to search for interactions. This choice of material is particularly beneficial, as xenon can theoretically accommodate various dark matter particles, from weakly interacting massive particles (WIMPs) to others yet to be defined.
However, there are downsides to these efforts. While the depth offers safeguards from external interferences, it limits accessibility for maintenance and upgrades. Moreover, the sensitivity of these detectors must be amplified continuously, which requires advancements in technology and significant investment.
Superconducting Detectors
Superconducting detectors are another method with great potential in dark matter research. These detectors rely on the principles of superconductivity to detect energy from dark matter interactions. One key characteristic of superconducting detectors is their extreme sensitivity, which can detect minuscule energy deposits that would go unnoticed by other types of detectors.
Their unique feature lies in the ability to measure single photons or phonons—these are tiny packets of light or sound that can be created when a dark matter particle interacts with a material. This makes superconducting detectors exceptionally well-suited to capture weak signals, which is often the case with potential dark matter interactions.
However, these detectors are not without their challenges. Maintaining the superconducting state requires ultra-cold conditions, which adds complexity to the experiment setups. Moreover, the dependency on advanced materials and fabrication techniques can increase costs and limit deployment.
Indirect Detection Methods
Indirect detection methods look at the byproducts of dark matter interactions instead of trying to catch them directly. This can include observing cosmic rays or other particles that may hint at dark matter’s presence. By focusing on secondary effects, these methods open up a different avenue in dark matter research.
Observations of Cosmic Rays
Observations of cosmic rays provide a way to infer dark matter interactions through the high-energy particles that reach Earth from space. One of the key aspects of cosmic rays is their abundance and energy; they bombard the Earth’s atmosphere from all directions, making them a consistent area for observation.
The significant characteristic of these observations is that they can be conducted using ground-based or space-based telescopes. Facilities like the Pierre Auger Observatory gather data on cosmic rays which can offer hints toward dark matter collisions in regions of space where it might gather at larger concentrations. This indirect method can lead to discoveries that are otherwise unattainable through direct detection.
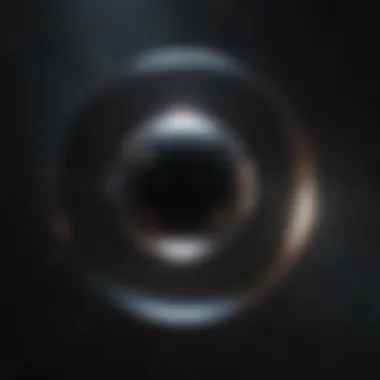
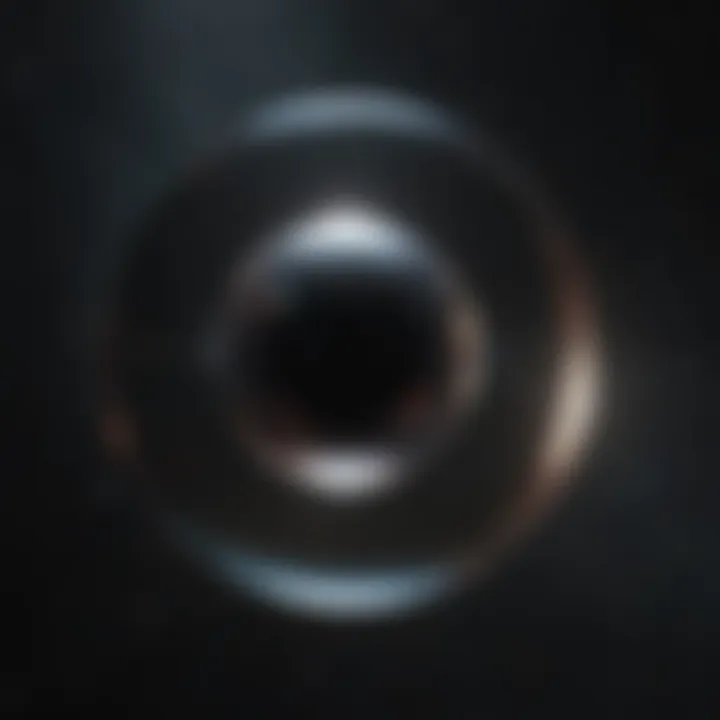
However, tracing specific signals back to dark matter interactions is complicated by the multitude of processes that contribute to cosmic ray production, including but not limited to supernovae and black hole activity. Thus, while the data can point towards dark matter, interpreting the signals is not straightforward, necessitating further models and theoretical work.
Gamma Rays and Dark Matter Annihilation
Gamma rays are another indirect method of detecting dark matter. Dark matter annihilation, likely occurring in dense areas of the universe, can produce high-energy gamma rays. This method is of particular interest because gamma rays travel vast distances unimpeded, providing a clearer idea of high-energy events in the universe.
The main advantage of focusing on gamma rays is their piercing nature, which allows them to escape dense environments such as the centers of galaxies. Space-based observatories like the Fermi Gamma-ray Space Telescope have been pivotal in identifying regions where dark matter might be interacting based on the observations of excess gamma ray emissions.
The challenge, however, lies in differentiating between gamma rays that result from dark matter interactions and those from standard astrophysical sources. This conundrum means that while the gamma rays can signal dark matter presence, careful interpretation and correlation with other data are necessary to confirm the findings.
In summary, both direct and indirect detection methods are essential to the ongoing pursuit of understanding dark matter, each carrying unique benefits and obstacles.
Through these current experimental efforts, researchers hope to piece together the puzzle of dark matter, gaining insights not only into this mysterious substance but also into the fundamental workings of our universe.
Future Directions in Dark Matter Research
The exploration of dark matter is far from being a finished chapter in the cosmic narrative. Research in this area continues to push boundaries, embracing both innovative ideas and sophisticated technologies. Understanding the future directions in dark matter research is crucial for multiple reasons: it offers a fertile ground for groundbreaking discoveries, propels technological advancement, and may even reshape our fundamental understanding of physics itself. By exploring what lies ahead, the scientific community prepares itself for both potential, unexpected challenges and rewards that can unravel the very fabric of our universe.
Upcoming Experiments
One of the pivotal elements that drives the search for dark matter is the series of upcoming experiments that scientists have lined up. These efforts aim not only to detect dark matter particles directly but also to further examine their properties through various indirect means. Here’s a rundown of some notable experiments on the horizon:
- LUX-ZEPLIN (LZ): This cutting-edge facility in South Dakota aims to employ a two-phase xenon time projection chamber to detect potential signals from WIMPs, one of the leading candidates for dark matter.
- PICO: Developed in Canada, this experiment seeks to identify dark matter by using superheated fluids that bubble in the presence of these elusive particles.
- DUNE: The Deep Underground Neutrino Experiment will utilize a large detector to study neutrino interactions that may provide insights on dark matter scenarios.
In addition to these, several more proposals are being discussed, constantly expanding the toolkit available to researchers. New methodologies may shed light on dark matter in ways previously deemed impossible.
The Role of Artificial Intelligence
In recent years, artificial intelligence has emerged as a transformative force across various scientific domains. Its role in dark matter research is an exciting frontier. AI's capabilities to analyze massive datasets, recognize complex patterns, and predict possible interactions can significantly enhance both detection and theoretical modeling efforts. Here’s how artificial intelligence contributes:
- Data Analysis: With experiments generating vast quantities of data, AI tools can quickly sift through and identify patterns that might otherwise go unnoticed. For instance, machine learning algorithms can recognize signals of dark matter interactions amidst numerous background noise events.
- Predictive Modeling: AI can assist in simulating dark matter's behavior under various theoretical frameworks, guiding researchers toward the most promising lines of inquiry. This helps in formulating testable hypotheses that can be validated by future experiments.
- Optimization of Detectors: AI can help optimize the design and operation of detection experiments. By analyzing performance data, machine learning models can suggest adjustments that may improve sensitivity and accuracy.
"The intersection of artificial intelligence and dark matter research exemplifies how traditional fields can benefit from interdisciplinary approaches."
The Philosophical Implications of Dark Matter
The concept of dark matter extends beyond mere scientific inquiry, pulling at the threads of philosophical thought. Its existence necessitates a reevaluation of fundamental beliefs about the universe, reality, and our position within it. Questions about what we can know and how we can understand nature come to the forefront when considering that a vast majority of the universe remains unseen and undetectable.
Importantly, the implications of dark matter resonate through the corridors of human thought, raising considerations about our knowledge of the cosmos. How does one reconcile the visible and invisible? What does it mean to seek understanding in a universe that contains more mystery than certainty? Such inquiries are particularly salient in the realm of cosmology and serve to both challenge and expand human insight.
"The universe is under no obligation to make sense to you." - Neil deGrasse Tyson
Nature of Reality
The existence of dark matter fundamentally alters our understanding of reality. As scientists investigate the cosmos and its intricacies, they uncover that only 5% or so of the universe is visible matter, including stars and planets. The remaining 95% includes dark energy and dark matter, making a compelling case that the empirical reality we observe is but a fraction of the broader universal scheme.
This observation positions dark matter as a pivotal player in shaping our cosmic narrative, compelling philosophers and scientists alike to rethink established truths. The notion of a universe populated predominantly by unseen forces forces a confrontation with the boundaries of human perception and the reliability of our senses. It pushes us to grapple with abstract concepts that elude direct observation and understanding.
Here are some key questions that arise in this context:
- What implications does dark matter have for our ontological beliefs?
- To what extent does our visual-centric understanding of reality limit our comprehension of the universe?
- How does the interplay of visible and invisible matter challenge established epistemological frameworks?
The Limits of Human Understanding
Dark matter also shines a light on the intellectual boundaries we navigate as we aspire to comprehend the cosmos. Despite rapid advancements in technology and theoretical frameworks, genuine knowledge remains tantalizingly out of reach. In a world where dark matter and dark energy play leading roles, we must confront the reality that our grasp of the universe is inherently incomplete.
Philosophically, this raises questions about the nature of scientific endeavor. Are we, as a species, doomed to perpetually chase shadows in our quest for knowledge? Or could this persistent obscurity serve as the catalyst for deeper inquiry and understanding? The limitations of human cognition become even clearer when considering that much of our technological advancement hinges on a relational understanding of the universe, often rooted in that same visible 5%.
This is reflected in the following ideas:
- Science thrives on continually questioning and testing existing beliefs.
- Intrigue and wonder can fuel further exploration, even when answers remain elusive.
- A humility rooted in the unknown may drive collective intellectual progress.
Conclusively, the implications of dark matter weave through both the fabric of reality and the limits of our understanding. As we delve deeper into cosmic mysteries, this journey invokes not only scientific inquiry but also a series of philosophical reflections that can redefine our place in the universe.
The End
In summarizing this exploration of dark matter theory, we arrive at a pivotal juncture in our understanding of the cosmos. Dark matter, despite being invisible to the naked eye and detectable only through its gravitational effects, plays a crucial role in shaping the structure and behavior of galaxies and larger cosmic systems. The evidence we’ve examined—from galaxy rotation curves to the cosmic microwave background—illuminates the profound implications of dark matter for both theoretical frameworks and observational astronomy.
Summary of Key Points
As we reflect on the journey through the complexities of dark matter, certain key points emerge as paramount:
- Presence and Influence: Dark matter constitutes about 27% of the universe, influencing the formation and motion of galaxies across cosmic time scales.
- Variety of Evidence: Multiple lines of evidence, including gravitational lensing and cosmic microwave background radiation, reinforce the existence of this elusive substance.
- Theoretical Models: Various theoretical approaches, such as supersymmetry and modified gravity theories, seek to explain the fundamental nature of dark matter and its interactions, fueling ongoing research.
- Detection Efforts: While detection remains a formidable challenge, the continual development of advanced technologies and methodologies showcases the resilience of the scientific community in uncovering the mysteries surrounding dark matter.
Overall Importance of Dark Matter Theory
The significance of dark matter theory extends far beyond mere academic inquiry. Understanding dark matter is fundamental to several critical areas:
- Cosmological Models: The standard model of cosmology—Lambda Cold Dark Matter—relies heavily on dark matter to explain the universe’s large-scale structure.
- Future Innovations: With the role of artificial intelligence within experimental research, potential breakthroughs in understanding dark matter may redefine our approach to astrophysics and particle physics.
- Philosophical Considerations: Dark matter challenges our perception of reality, edging us closer to existential questions about the universe and our place within it.
Thus, studying dark matter not only informs our understanding of the cosmos but also nurtures an insatiable curiosity about the universe's fundamental nature. It pushes the boundaries of science, inviting both scholars and novices alike to journey deeper into the fabric of reality.