The Groundbreaking Discovery of CRISPR in 1987
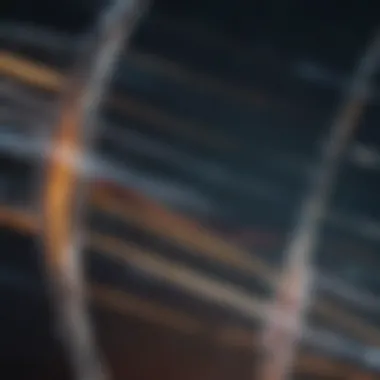
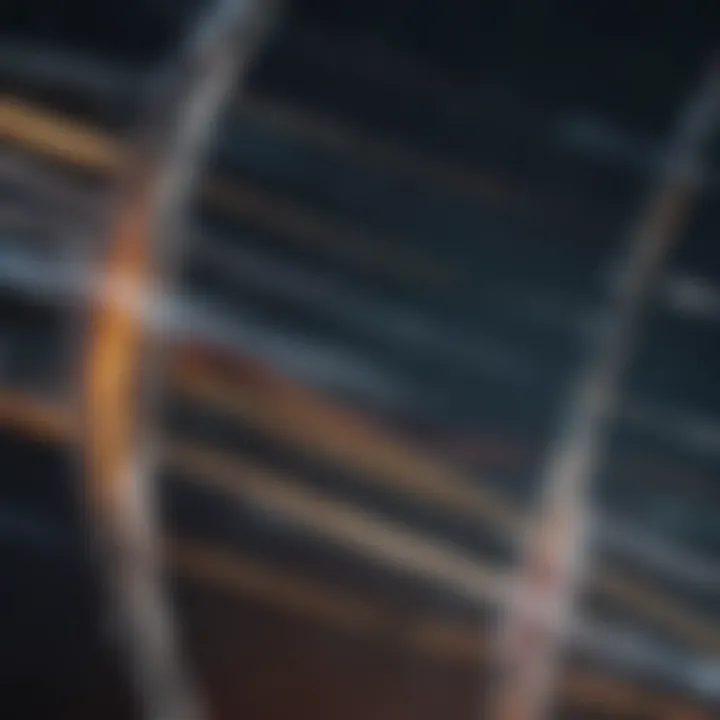
Intro
In the realm of molecular biology and genetics, few developments have proved as pivotal as the discovery of CRISPR in 1987. The term CRISPR stands for Clustered Regularly Interspaced Short Palindromic Repeats. This breakthrough opened new frontiers in genetic research, enabling unprecedented capabilities for gene editing and manipulation.
The foundational studies conducted during this period revealed the existence of unique DNA sequences in bacteria. These sequences functioned as a form of adaptive immunity, providing bacteria the ability to recognize and defend against invading viruses. Researchers at the time, notably Francisco Mojica, laid the groundwork for what would become a transformative technology. Understanding these natural processes has since led to innovations with capabilities that extend beyond theoretical interest. Consequently, the CRISPR technology has begun reshaping diverse fields, from biotechnology to medicine.
In this article, we will explore the key findings and implications of this discovery, along with the contributions from the scientific community. Through a comprehensive analysis, the article aims to bridge the knowledge gap regarding CRISPR's significance and its ongoing evolution.
Prolusion to CRISPR
The introduction of CRISPR technology represents a significant leap in the fields of genetics and molecular biology. Initially discovered in 1987, this groundbreaking mechanism has shaped our understanding of gene editing, offering a robust tool for scientists. Its unique ability to target specific DNA sequences makes it invaluable for research and practical applications. The discovery has opened new avenues in biotechnology, allowing for precise modifications in various organisms.
CRISPR stands for Clustered Regularly Interspaced Short Palindromic Repeats. This system has evolved to function as an adaptive immune response in bacteria, where it recognizes and eliminates viral DNA. A deeper understanding of this natural defense mechanism led scientists to explore its potential in genetic engineering and medicine.
Benefits of CRISPR technology include:
- Precision: CRISPR can specifically target DNA sequences, reducing off-target effects compared to older technologies.
- Efficiency: The process is generally quicker and more cost-effective, making it accessible for various research purposes.
- Versatility: It can be tailored for use across different species, from plants to humans.
Despite its potential, CRISPR also raises important considerations related to ethics and safety. As its applications grow, there is a need for careful regulatory frameworks to ensure responsible use.
In summary, the introduction of CRISPR marks a pivotal milestone in genetic research. Its implications extend beyond academia, touching on ethical dilemmas and societal impacts. As research continues to unfold, understanding CRISPRโs foundational aspects becomes essential for appreciating its ongoing evolution and future prospects.
Overview of CRISPR Technology
CRISPR technology has revolutionized how scientists approach genetic modification. At its core, CRISPR employs a guide RNA that directs the Cas9 enzyme to specific locations within the genome. This mechanism enables precise cuts in DNA, allowing researchers to add, remove, or alter genetic material. Its simplicity in design and application contrasts sharply with earlier gene-editing methods, making it a preferred choice in labs worldwide.
The significance of the CRISPR-Cas9 system cannot be underestimated:
- Improved Targeting: High specificity minimizes unintended changes in the genome, a common issue with previous techniques.
- Adaptable Control: Researchers can design custom guide RNAs to target nearly any sequence, easing the burden of extensive modification.
- Broad Applications: Beyond basic research, CRISPR has implications for agriculture, medicine, and environmental science, showcasing its potential to address critical issues in each field.
"The potential of CRISPR in transforming industries is undeniable, providing solutions to challenges that were once deemed insurmountable."
As an emerging technology, CRISPR continues to attract significant interest from the scientific community. New innovations, including improvements in delivery methods and accuracy, expand its usability, solidifying its position at the forefront of genetic research. Understanding the principles underlying CRISPR is essential for any stakeholder involved in biotechnology today.
Historical Context
The historical context surrounding the discovery of CRISPR is essential to understand. This period serves as a backdrop that highlights the state of genetics and molecular biology in the late 20th century. Before the breakthrough of CRISPR in 1987, the field was making significant strides but was limited in its tools and understanding.
Genetics and Molecular Biology in the 1980s
In the 1980s, genetics and molecular biology were undergoing rapid development. Scientists were just beginning to unlock the complexities of DNA, genes, and the mechanisms underpinning heredity. Various technologies were emerging that enabled researchers to manipulate genetic material, providing a foundation for future innovations.
- Polymerase Chain Reaction (PCR), developed by Kary Mullis in 1983, allowed for the amplification of specific DNA sequences. This innovation propelled genetic research by enabling researchers to generate enough DNA for analysis.
- The introduction of recombinant DNA technology spurred excitement within the scientific community. Researchers could now combine DNA from different organisms, leading to increased understanding of gene function and regulation.
- Genetic mapping initiatives gained momentum. Projects like the Human Genome Project, although started in the early 1990s, were influenced by research efforts in the 1980s.
Despite these advancements, the field lacked a comprehensive understanding of bacterial immune systems. Scientists were curious about how bacteria defended themselves from viral infections, yet the mechanisms remained mostly obscure. This gap in knowledge set the stage for the groundbreaking findings of Yoshizumi Ishino and his team in 1987.
As Ishinoโs research progressed, it began to shine a light on an uncharted aspect of microbial life: the sequences of DNA that later became known as CRISPR. Recognizing the significance of this discovery required a laid foundation of existing knowledge in genetics, which had been evolving through previous decades.
With the stage properly set, the subsequent sections of this article will cover the discovery of CRISPR, analyzing how it interconnected with earlier developments in genetics and how it ultimately revolutionized the entire field.
Discovery of CRISPR by Yoshizumi Ishino
The discovery of CRISPR by Yoshizumi Ishino represents a pivotal moment in the realm of genetics. In 1987, Ishino and his colleagues identified unique repeated sequences in the DNA of Escherichia coli. This initial finding laid the groundwork for the intricate understanding of CRISPR that emerged later on. The implications of this discovery extend far beyond mere scientific curiosity; they open new avenues for biotechnology and genetic engineering. As researchers began to realize the potential of CRISPR sequences, the field rapidly evolved, leading to innovative applications in medicine, agriculture, and beyond.
Key Research Publication
In their seminal paper, published in 1987, Ishino and his team detailed their findings regarding the unusual sequences in E. coli. They noticed that these sequences were unique and consistently repeated, which raised questions about their function. While at that time the significance of these sequences was not fully understood, the publication served as a cornerstone for future research. This study, therefore, became a touchstone within the geneticist community, inspiring subsequent investigations into the nature and role of CRISPR.
The researchers, including include Yoshizumi Ishino, Takafumi Morita, Miki H. Shinagawa, and others, emphasized the significance of these sequences in their research, despite not grasping their potential use in gene editing. The sheer novelty of their findings set the stage for an explosion of interest in CRISPR, which would follow in upcoming years.
Ishino's Research Environment
Yoshizumi Ishino worked in a research environment that was both supportive and intellectually stimulating. The late 1980s marked a time of rapid advancements in molecular biology and genetics. Many scientists were collaborating on various projects, sharing ideas, which catalyzed their discoveries. In this environment, Ishino found opportunities to explore the intersection of microbiology and genetic research.
Working at the University of Tokyo, his laboratory was equipped with the latest technology of that era, which allowed for detailed genetic analysis. This setting led Ishino to investigate the genetic components of E. coli extensively. Although the significance of CRISPR sequences would not be fully recognized until later, the groundwork laid in Ishino's lab was critical for future breakthroughs in the understanding of bacterial immunity and genetic engineering.
Overall, the discovery of CRISPR by Yoshizumi Ishino is a reminder of how foundational research often evolves into groundbreaking scientific advancements. The journey from recognition of repeated sequences to the current applications in CRISPR-based gene editing illustrates the long arc of scientific inquiry and innovation.
Characterization of CRISPR
The characterization of CRISPR represents a pivotal moment in comprehending the mechanics of bacterial genetics. This section delves into critical aspects of CRISPR that reveal not just its structure but also its function and various contributions to genetic science. Understanding CRISPRโs mechanisms is integral for applications spanning biotechnology, medicine, and other areas of research.
Identifying Repeated Sequences
Repeated sequences are a hallmark of the CRISPR structure. Found within a bacterium's genome, these sequences are termed Clustered Regularly Interspaced Short Palindromic Repeats (CRISPR). Each repeat stands as a mirror image, often involving short DNA sequences that are identical or closely similar. The repetitions can vary between different strains of bacteria, reflecting the diversity within microbial communities.
Identifying these sequences is crucial for several reasons:
- Genetic Mapping: Recognizing the pattern of repeated sequences aids in mapping bacterial genomes. This is fundamental for both basic research and clinical applications.
- Functional Insights: Through studies, scientists can ascertain whether specific repeat sequences correlate with specific bacterium resilience against viral threats.
- Technological Innovation: Knowledge of these sequences has paved the way for advancements in genome-editing tools, notably CRISPR-Cas9.
"The identification of repeated sequences in CRISPR is not only significant for taxonomy but also vital for understanding evolutionary biology and microbial ecology."
This structural identification retains implications in biotechnology, underscoring the importance of repeated sequences in understanding and manipulating genetic elements.
Understanding Spacer Sequences
Spacer sequences represent another critical component of CRISPR systems. These DNA segments are inserted between the repeated sequences and are unique to each bacterium. Spacer sequences serve as a record of past viral infections, capturing snippets of viral DNA that the bacterium has previously encountered. This record is vital because it allows for a form of adaptive immunity against future threats from the same viruses.
The process is as follows:
- Acquisition: When a bacterium encounters a virus, it can incorporate a piece of the viral DNA into its genome as a new spacer.
- Expression: The bacterium then expresses these spacers as RNA molecules.
- Interference: If the same virus attempts to infect again, the bacterium can recognize it and mount a defense by cutting the viral DNA using CRISPR-associated proteins (Cas proteins).
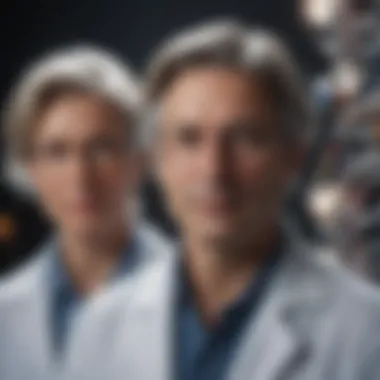
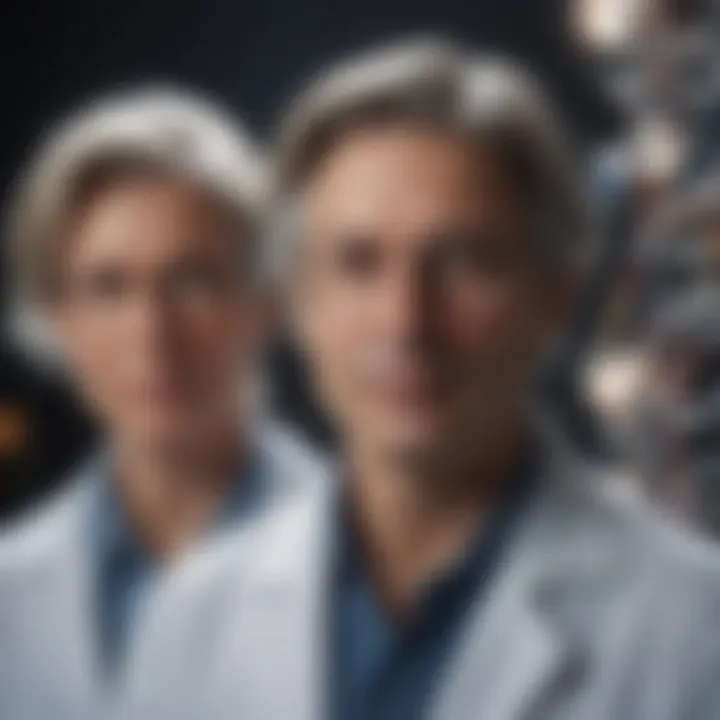
Understanding these spacer sequences is important in various research fields:
- Viral Interaction Studies: Analyzing how bacteria acquire these sequences enlightens researchers about the co-evolution of viruses and hosts.
- Biotechnological Applications: The understanding of spacer sequences has directly influenced the design of synthetic biology tools and genome editing technologies. Researchers can tailor CRISPR systems to target specific genes, enhancing precision in genetic manipulation.
In summary, the characterization of CRISPR focuses on identifying unique features within its structure, primarily repeated and spacer sequences. This knowledge not only deepens our understanding of microbial defense mechanisms but also facilitates significant biotechnology advancements, making CRISPR a powerful tool in genetic research.
Functional Implications of CRISPR
CRISPR technology has far-reaching implications that extend beyond its initial discovery in 1987. Understanding these implications is crucial for grasping the transformative role CRISPR plays in science and medicine today. This section focuses on two essential aspects: its role in bacterial immunity and its connection to adaptive immune systems. Both elements illustrate the practical utility of CRISPR, shedding light on its significance in the context of biological research and therapeutic applications.
Role in Bacterial Immunity
Bacteria have long faced threats from various phages, which can lead to cell lysis and eventual death. The introduction of CRISPR systems offers a sophisticated answer to this challenge. CRISPR provides bacteria with a form of adaptive immunity. It does this by integrating short sequences of phage DNA into the CRISPR locus of the bacterial genome. This process enables the bacteria to create a memory bank of previous infections.
Each time a phage attempts to infect a bacterium, the bacterium can transcribe these sequences into RNA. This RNA then guides Cas proteins to specifically target and cut the DNA of the invading phage. This mechanism ensures that the bacteria are better equipped to defend themselves against future phage invasions.
The intricacies of this system are highlighted in the following points:
- Memory Formation: Bacteria store information about past infections, which aids in quicker and more efficient responses.
- Specificity: The precision with which CRISPR identifies and destroys viral DNA minimizes collateral damage to the bacterial genome.
- Evolutionary Advantage: Bacteria with effective CRISPR systems have a selective advantage in environments with high phage loads, thus promoting their survival and proliferation.
Adaptive Immune Systems
The impact of CRISPR systems extends beyond the individual cells of bacteria. Understanding these systems reveals the incredible complexity of microbial life. Adaptive immunity, as seen in higher organisms, can be paralleled with bacterial systems enabled by CRISPR.
Bacteriophages evolve rapidly, necessitating that bacterial defenses also adapt concurrently. This mutual evolution exemplifies an arms race in the microbial world. The adoption of CRISPR systems in other organisms is now an area of great interest for researchers. Some of the significant aspects include:
- Cross-Species Applications: Insights gained from bacterial systems are being mirrored in research on immune systems of plants and animals, enhancing understanding of immune memory in different biological contexts.
- Gene Editing Potential: Utilizing CRISPR as a tool for genome editing harnesses the natural mechanisms of bacterial immunity, enabling precise modifications in complex organisms, including humans.
CRISPR represents a fundamental breakthrough that extends our comprehension of immune functions at both bacterial and organismal levels.
These functional implications form the basis of CRISPR's significance in contemporary research, particularly in genetics and biotechnology. The understanding of bacterial immunity and adaptive immune systems not only provides insight into the natural world but also paves the way for innovative applications in medical science, particularly in genetic engineering and therapeutic development.
Evolution of CRISPR Research
The evolution of CRISPR research represents a significant transformation in genetic sciences, impacting various fields such as biotechnology, medicine, and agriculture. This section highlights the timeline and developments that followed the discovery of CRISPR in 1987, emphasizing key advancements, increased understanding of its mechanisms, and its application as a gene-editing tool.
Advancements Post-1987
Following the initial discovery of CRISPR sequences, researchers began to explore these elements further. As the years progressed, the focus shifted from mere identification to understanding the functional roles of CRISPR in bacteria.
Important advancements included:
- Identification of Cas Genes: In the early 2000s, scientists began identifying CRISPR-associated (Cas) genes, which play a crucial role in the operational machinery of CRISPR. This was a major step toward comprehending how CRISPR functions as part of a bacterial immune system.
- First Characterizations: By the late 2000s, researchers like Francisco Mojica were pivotal in characterizing CRISPR functions, largely establishing the framework for genetic engineering applications. His work was instrumental in revealing how CRISPR stores and retrieves viral DNA segments, ultimately leading to a developed adaptive immune mechanism in bacteria.
- Tool Development: The advent of techniques such as CRISPR-Cas9 in the early 2010s revolutionized gene editing. This tool emerged from understanding how Cas proteins target and edit specific DNA sequences in genomes, showcasing the practical applications of CRISPR technology.
These advancements laid a foundation for various practical applications of CRISPR, positioning it as a transformative technology in genetics.
CRISPR as a Gene-Editing Tool
CRISPR's application as a gene-editing tool marks one of the most exciting developments in modern biotechnology. The simplicity and efficiency of the CRISPR-Cas9 system allow for precise modifications to DNA, opening new avenues for research and therapy.
- Mechanism of Action: The CRISPR-Cas9 system functions by using a guide RNA to lead the Cas9 protein to a specific DNA sequence. Once bound, Cas9 introduces double-strand breaks, which the cell attempts to repair. This repair process can be harnessed to modify genes effectively.
- Applications in Human Health: Researchers have begun exploring CRISPR's potential in treating genetic disorders, such as sickle cell anemia and cystic fibrosis. Early trials indicate promising outcomes, illustrating CRISPR's capacity for transformative therapeutic interventions.
- Agricultural Improvements: Beyond human health, CRISPR technology is now applied in agriculture to enhance crop resilience and yield. For example, gene-editing has been used to develop crops with better drought resistance and improved nutritional profiles.
"CRISPR technology is more than a tool; it is a catalyst that has significantly changed the landscape of genetic engineering, creating possibilities previously deemed unattainable."
Overall, the evolution of CRISPR research has shifted from foundational discoveries to applications with substantial implications for society. This journey illustrates not only the scientific progress but also the potential for CRISPR to redefine approaches across many disciplines.
Key Figures in CRISPR Development
The journey of CRISPR technology is not solely defined by the scientific discoveries made, but significantly influenced by the key figures that propelled it forward. Notable individuals such as Jennifer Doudna and Emmanuelle Charpentier led the way, igniting a wave of research interest around CRISPR. Their combined efforts, along with contributions from other researchers, created a strong foundation for understanding and utilizing CRISPR. This section explores their critical roles and broader implications in the development of gene-editing technologies.
Jennifer Doudna and Emmanuelle Charpentier
Jennifer Doudna and Emmanuelle Charpentier are pivotal in the CRISPR narrative. Their landmark paper published in 2012 detailed the mechanisms through which CRISPR systems operate, particularly in Escherichia coli. They demonstrated how the CRISPR-Cas9 system could be used for precise gene editing. This discovery opened doors to new possibilities in genetic engineering.
Doudna, a professor at the University of California, Berkeley, was instrumental in merging ideas from various fields such as molecular biology, genetics, and biochemistry, bringing her unique perspective to the CRISPR framework. Similarly, Charpentier, with her deep expertise, brought in critical insights into the role of the Cas9 protein.
Their collaboration is noteworthy not only for the scientific advancements but also for how it redefined partnerships within the scientific community. Together, they shared various accolades, including the prestigious Breakthrough Prize in Life Sciences. This recognition reflects the impact of their work on the global scientific landscape, encouraging further research in genetic modification.
Contributions of Other Researchers
While Doudna and Charpentier are often the faces associated with CRISPR, many other researchers have significantly contributed to its development. For instance, Feng Zhang has worked extensively on the application of CRISPR technology in human cells. His efforts have expanded the scope of CRISPR's capabilities, making gene editing more efficient and applicable in biomedical research.
- Contributions from scientists like
- Feng Zhang: Focused on applying CRISPR in eukaryotic cells.
- George Church: Advocated for the integration of CRISPR in various genetic applications.
- Martin Jinek: Worked on clarity of the CRISPR-Cas9 mechanism, refining its operational method.
These researchers have not only pushed the boundaries of what was thought possible with CRISPR, but also fostered an environment of collaboration across disciplines, enhancing innovation.
Their endeavors ensure a comprehensive understanding of the science behind CRISPR. This collective effort reminds us that scientific progress is rarely a solo endeavor. Through integration of multiple perspectives and expertise, the CRISPR field continually evolves, reflecting the collective knowledge of its contributors.
Impact on Biotechnology and Medicine
The impact of CRISPR technology on biotechnology and medicine is profound and multifaceted. This revolutionary tool has transformed the landscape of genetic engineering, enabling scientists to edit genes with unprecedented precision and efficiency. Announced formally in literature by Yoshizumi Ishino in 1987, CRISPR's potential continued to evolve, becoming a pivotal element in modern biology.
One significant application of CRISPR is in genetic engineering. This process allows researchers to modify or replace genes within organisms. The precise nature of CRISPR can result in specific changes, which is far superior to older techniques that were often imprecise and cumbersome. Notably, organisms such as Escherichia coli and Drosophila melanogaster have been subject to gene edits that have far-reaching benefits in fields from agriculture to pharmaceuticals. Some notable applications include:
- Creating genetically modified crops that are resistant to pests and diseases.
- Developing animal models for studying human diseases.
- Accelerating the discovery of novel therapeutic compounds.
Consequently, these advancements offer potent solutions to global challenges, such as food security and health care. This functionality makes CRISPR a cornerstone of modern biotechnology.
Applications in Genetic Engineering
CRISPR has wide-ranging applications in genetic engineering. The versatility of the CRISPR-Cas9 system assists in a variety of scientific endeavors.

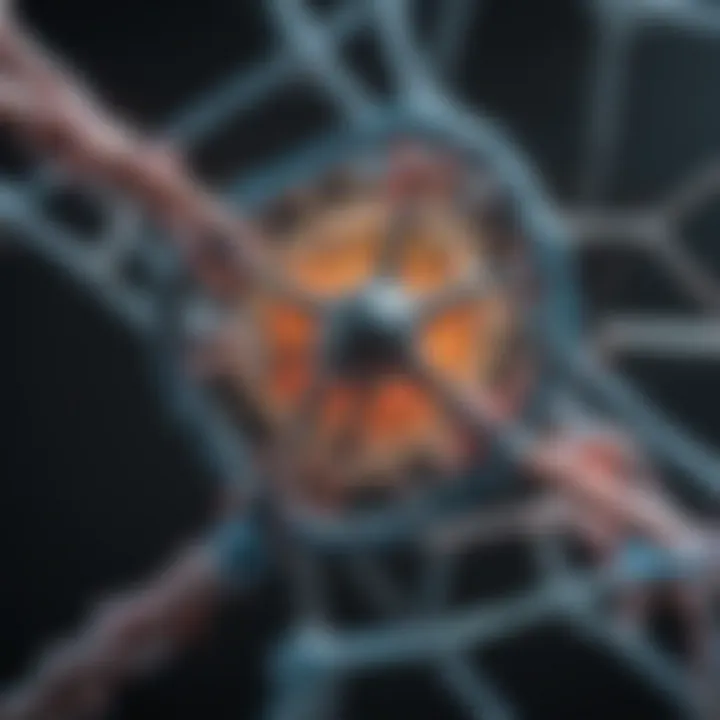
Some key applications include:
- Gene knockout: Disabling specific genes to observe the impact on phenotypes.
- Gene addition or replacement: Introducing new or corrected genes to restore function.
- Genome-wide screens: Identifying gene function by systematically knocking out genes across genomes.
Furthermore, its simplicity enables rapid advancements in research and development timelines. As it continues to be refined, its applications are expected to expand even further.
Potential in Therapy Development
Another significant area where CRISPR holds promise is in therapy development. The ability to edit genes opens new avenues for treating genetic disorders, cancers, and infectious diseases. The prospect of correcting genetic mutations at their source is especially compelling. Some of the notable therapeutic potentials include:
- Sickle cell disease: Pursuing gene therapy to correct the mutation in hemoglobin.
- Cystic fibrosis: Editing the CFTR gene to restore its function in affected patients.
- Cancer treatment: Targeting oncogenes to inhibit tumor growth.
Furthermore, CRISPR's ability to engineer immune cells presents innovative strategies to enhance the body's natural defenses. As promising as these applications seem, clinical translation requires addressing many technical and ethical challenges.
"CRISPR technology is like a powerful Swiss army knife in the laboratory, allowing us to tackle diverse challenges in genetic research and medicine."
In essence, the impact of CRISPR on biotechnology and medicine cannot be overstated. As research progresses, the integration of CRISPR into therapeutic practices expands our understanding and control of genetic illnesses, thus holding the potential to revolutionize healthcare on a global scale.
Ethical Considerations in CRISPR Research
The advent of CRISPR technology has ushered in not only a new era for genetic engineering but also a myriad of ethical considerations that must be carefully navigated. Understanding the implications of gene editing, particularly at the level of human germline modification, raises questions about the morality of altering genetic material that can affect future generations. The ethical discourse surrounding CRISPR revolves around the potential benefits it brings against the possible risks of misuse or unforeseen consequences. As CRISPR continues to evolve in its applications, the need for robust ethical frameworks becomes increasingly critical.
Debates on Gene Editing Ethics
Debates in the scientific community highlight a spectrum of opinions regarding the ethics of gene editing. Proponents argue that CRISPR offers unprecedented opportunities to cure genetic diseases and improve agricultural efficiency. Gene therapy targeting conditions such as cystic fibrosis or sickle cell disease showcases the technology's transformative potential. However, critics emphasize the moral hazards involved. The idea of creating "designer babies" evokes concerns about eugenics and social inequality, raising the question: should humanity play a role in determining the genetic makeup of individuals?
A key aspect of these debates focuses on:
- Safety: The potential for unintentional mutations that could arise from CRISPR applications.
- Accessibility: How equitable will access to these technologies be across different socioeconomic groups?
- Consent: The impossibility of obtaining informed consent from future generations whose genomes are being edited.
"The possibilities from CRISPR are immense, but ethical responsibility cannot be overshadowed by the excitement of innovation."
Regulatory Frameworks
The establishment of regulatory frameworks is essential to guide the responsible application of CRISPR technology. In many countries, regulations have lagged behind scientific advancements, creating a gap that could lead to unethical practices. Current frameworks vary widely across the globe, influenced by cultural, social, and political factors.
In the United States, regulatory oversight mainly falls under the jurisdiction of agencies such as the Food and Drug Administration (FDA) and the National Institutes of Health (NIH). Their guidelines govern clinical applications of CRISPR, emphasizing safety and efficacy. Conversely, the approach in countries like China has shown a more relaxed regulatory environment, raising alarm about potential abuses of gene-editing technologies.
This disparity underscores the need for:
- International Collaboration: Aligning global standards for the use of CRISPR can foster safer research practices.
- Public Involvement: Engaging the public in discussions about the implications of CRISPR can enhance transparency and trust.
- Continuous Evaluation: As new advancements arise, regulatory frameworks must adapt to address emerging bioethical challenges.
Overall, ethical considerations in CRISPR are paramount. Balancing innovation with responsibility will dictate the future trajectories of this groundbreaking technology.
Future Directions of CRISPR Research
The exploration of future directions in CRISPR research holds immense significance in advancing genetic engineering. As technology develops, the potential applications of CRISPR continue to expand, promising solutions to longstanding global challenges such as disease, food security, and environmental concerns. Researchers must keep abreast of emerging trends and innovations in this domain.
Emerging Technologies in Genome Editing
The landscape of genome editing is rapidly evolving. Several cutting-edge technologies are converging with CRISPR, enhancing its capabilities and precision. Techniques such as CRISPR-Cas9 are becoming integrated with high-throughput sequencing, allowing for more accurate assessments of gene edits.
- New Cas Variants: Scientists are identifying novel CRISPR-associated (Cas) proteins that offer improved targeting and fewer off-target effects.
- Prime Editing: This technique, known for its ability to perform โsearch and replaceโ edits, minimizes insertions or deletions, offering higher fidelity than conventional CRISPR methods.
- Base Editing: This allows direct conversion of one DNA base to another without causing double-strand breaks, thus reducing unintended mutations.
By harnessing these advances, researchers are positioning CRISPR as a more versatile and safer tool for gene editing. These emerging technologies may facilitate breakthroughs in treating genetic disorders and enhancing agricultural resilience.
Interdisciplinary Collaborations
The future of CRISPR research increasingly relies on interdisciplinary collaborations. Engaging experts from diverse fields fosters innovation and enhances research effectiveness.
- Biology and Data Science: The integration of bioinformatics and machine learning is critical for analyzing the massive data generated from genomic studies, helping identify patterns that were previously undetectable.
- Ethics and Regulation: Interdisciplinary dialogue is essential in addressing the ethical implications of genome editing as well as shaping effective regulatory frameworks that ensure responsible use of CRISPR.
- Industry Partnerships: Collaborations with biotech companies can accelerate the translation of research findings into practical applications, such as new gene therapies and agricultural products.
These collaborative efforts will illuminate the path forward, enabling researchers to not only refine CRISPR technologies but also navigate the multifaceted challenges that its application brings.
"Emerging technologies and interdisciplinary partnerships are crucial for maximizing the potential of CRISPR while ensuring ethical considerations remain at the forefront of innovations."
Engaging in thoughtful discussions about the trajectory and implications of CRISPR will enhance its role in addressing both current and future scientific challenges.
CRISPR's Global Influence
The introduction of CRISPR technology has had a profound effect on scientific research and biotechnology across the globe. Its mechanisms are being utilized in diverse fields like medicine, agriculture, and environmental science, reshaping how scientists approach genetic modifications. CRISPR's global influence extends beyond laboratories, influencing public discourse about genetic research and its implications for society. As labs around the world explore its potential, the technology's benefits and challenges require careful consideration.
International Research Initiatives
Numerous international collaborative efforts have emerged due to CRISPR's capabilities. Research institutions worldwide are pooling resources to advance CRISPR applications. This cooperative approach accelerates discovery and innovation. For example, initiatives like the Comprehensive Genetic Research Program connect universities and biotech firms facilitating shared knowledge and technology.
The benefits of these collaborations are numerous:
- Resource Sharing: Countries with less funding benefit from access to advanced technology.
- Knowledge Exchange: Researchers can share techniques that improve understanding and application of CRISPR.
- Standardization of Practices: Collaborative frameworks lead to the establishment of global standards for CRISPR application and assessment, enhancing reproducibility in research.
Despite these advantages, challenges exist. Different regulatory environments can complicate collaborative efforts and result in ethical concerns.
CRISPR in Developing Countries
CRISPR technology is particularly promising for developing countries. The potential applications in agriculture and healthcare are transformative. For example, genetically modified crops can enhance food security by creating plants resistant to pests and diseases, which is crucial for regions prone to food shortages.
In healthcare, CRISPR holds the potential to address genetic diseases more effectively. Many developing nations suffer from high rates of infectious and hereditary diseases. Introducing CRISPR-based therapies could significantly improve health outcomes, making treatments more accessible and affordable.
However, the implementation of CRISPR in these areas is not without challenges. Some key issues include:
- Infrastructure: Limited facilities and lack of technology can hinder research and practical application.
- Education and Training: There is a need for education programs to train local scientists on CRISPR techniques.
- Ethical Considerations: The adoption of any new technology must consider local cultural perspectives and ethical norms.
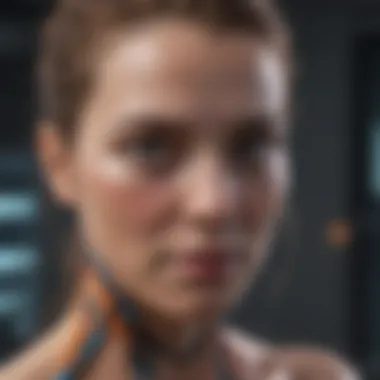
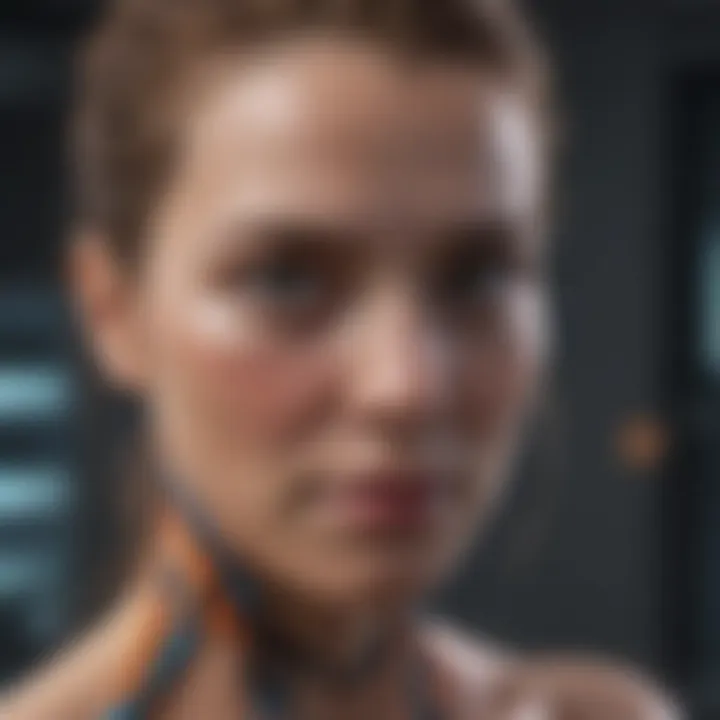
The impact of CRISPR is significant, but its success in diverse contexts depends on addressing the unique challenges faced by different regions.
CRISPRโs global influence is evident. The implications are substantial, paving the way for innovation, collaboration, and ethical considerations. Education and proper channels for implementing this technology will be crucial, especially in the developing world.
The Scientific Community's Reception
The discovery of CRISPR sequences in 1987 by Yoshizumi Ishino and his colleagues received increasing recognition within the scientific community over the following decades. This attention reflects not only the initial novelty of the findings but also the transformative potential that CRISPR technology has shown in various fields such as genetics, molecular biology, and biotechnology. Various elements contributed to this reception, highlighting the importance of peer recognition and collaborative endeavors in scientific research.
Peer Recognition and Awards
Awards play a vital role in shaping the narrative of scientific achievements. The early recognition of Ishinoโs work laid the groundwork for subsequent accolades awarded to researchers who contributed to the development of CRISPR. These awards often signify peers' acknowledgment of a significant discovery, further encouraging ongoing research and innovation.
Researchers involved in CRISPR development have received numerous honors, including:
- Nobel Prize in Chemistry (2020): Jennifer Doudna and Emmanuelle Charpentier were awarded for their pioneering work in developing the CRISPR-Cas9 gene-editing technology. Their groundbreaking research paved new pathways in genetic engineering.
- MacArthur Fellowship: Doudna received this prestigious fellowship for her work in molecular biology, showcasing the impact of her contributions on society and science.
- National Medal of Science: This is a testament to the recognition of exceptional contributions to the field of science, awarded to researchers who significantly advanced our understanding of the biological world.
Such recognitions not only validate individual efforts but also amplify the visibility of collective research endeavors in CRISPR technology.
Collaborations and Networking
The advancement of CRISPR technology is also traceable to effective collaborations within the scientific community. Networking among researchers allowed for knowledge exchange and innovation sharing, forming alliances that strengthened individual research capacities.
Key aspects of collaboration in CRISPR research include:
- Joint Research Projects: Many universities and research institutions engaged in joint projects, allowing researchers to pool resources, knowledge, and expertise.
- Conferences and Workshops: Events dedicated to genetics and molecular biology enabled scientists to present their findings, fostering dialogue and critical feedback which refined ongoing projects.
- Publications: Co-authored papers by diverse teams reflect the collaborative spirit of this research realm and disseminate knowledge, fueling further investigations.
The scientific community's reception of CRISPR highlights a dynamic interplay of recognition and collaboration. These elements are essential in establishing the foundational significance of CRISPR technology and in mapping its future trajectory in various applications.
Challenges in CRISPR Implementation
The implementation of CRISPR technology in research and clinical practices brings about several challenges that need careful consideration. Understanding these challenges is crucial to the responsible development and application of this powerful tool. As researchers and practitioners navigate the evolving landscape of genetic engineering, they must address both technical limitations and public perceptions that could significantly impact CRISPR's future.
Technical Limitations
One of the primary challenges faced in the application of CRISPR technology is the technical limitations inherent to gene editing. While CRISPR-Cas9 has revolutionized the field of genetics, it is not without flaws. Researchers have identified several key technical hurdles:
- Off-target effects: One significant concern is the potential for CRISPR to make unintended changes in the genome. This unpredictability can have serious implications, especially in therapeutic applications where accuracy is vital.
- Delivery mechanisms: Effectively delivering CRISPR components into target cells remains a challenge. Various delivery methods exist, such as viral vectors, but each has its own set of limitations regarding efficiency and immune responses.
- Complexity of genomic targets: The human genome's intricate structure adds another layer of complexity. Precise editing in specific regions can be difficult, particularly when dealing with repetitive sequences or large genomic areas.
Despite these issues, ongoing research aims to improve the specificity and efficiency of CRISPR technology. Scientists are actively working on developing advanced techniques and optimized delivery systems. These efforts are critical for expanding the use of CRISPR in medicine and agriculture.
Navigating Public Perception
Public perception plays a vital role in the acceptance and application of CRISPR research. As gene editing technology advances, it often sparks significant debate and ethical discussions. Understanding and addressing these concerns is essential for fostering a positive view of CRISPR.
The following aspects can influence public perception:
- Ethical implications: The ability to edit genes, especially in humans, raises ethical questions about designer babies, eugenics, and the long-term effects of such modifications. Society's views on these issues can vary widely, making it crucial to engage in open dialogue with the public.
- Misinformation: A lack of understanding regarding CRISPR technology can lead to misconceptions. Educational outreach and clear communication about the science behind CRISPR can help alleviate fears and promote informed discussions.
- Regulatory frameworks: Establishing and adhering to regulatory guidelines is necessary for building public trust. When individuals see that there are structured frameworks governing research and applications of CRISPR, they may feel more secure about its use.
"Public engagement is essential. Scientists need to communicate openly to demystify CRISPR technology and cultivate trust among communities."
By bridging the gap between scientific knowledge and public awareness, stakeholders in CRISPR research can work towards a more informed and supportive environment for its applications. Only through addressing these challenges can CRISPR technology reach its full potential.
Educational Outreach and Awareness
Educational outreach is crucial in disseminating knowledge about CRISPR technology. It raises awareness regarding its potential and implications in various fields such as genetic engineering and medicine. By improving understanding among students, researchers, and the public, outreach initiatives can foster informed discussions about the use of CRISPR.
University Programs and Workshops
University programs are pivotal in educating future scientists about CRISPR. Many institutions now offer specialized courses that address the technical, ethical, and practical aspects of this technology. Workshops provide hands-on experiences that enhance learning. Students can engage with CRISPR techniques, such as gene editing and analysis of genetic sequences. Programs often invite experts to speak, allowing students to learn directly from leaders in the field.
Benefits of these programs include:
- Enhancing practical skills in molecular biology.
- Encouraging critical thinking about the ethical implications.
- Building networks among peers and professionals.
Public Lectures and Seminars
Public lectures and seminars serve to bridge the gap between scientific communities and the general populace. They inform the public about CRISPR's possibilities while addressing misconceptions. Experts present findings and discuss ongoing research in an accessible manner. These events often encourage dialogue, allowing the audience to ask questions and express concerns.
Key considerations for these events include:
- Selecting engaging speakers who can simplify complex topics.
- Promoting discussions on ethical issues surrounding gene editing.
- Offering resources for further learning after the event.
"Informed public discourse will create a responsible environment for CRISPR research and application."
As a result, both university programs and public seminars play significant roles in shaping perceptions about CRISPR. They ensure that knowledge is not only academic but also available to anyone interested in the impacts of genetic research on society.
End
The exploration of CRISPR's discovery and subsequent advancements reveals its monumental significance in the realms of genetics and biotechnology. This section reflects on the transformative journey of CRISPR technology, highlighting its importance in various applications.
Reflections on CRISPR's Journey
The identification of CRISPR in 1987 paved the way for a revolution in genetic engineering. Initially discovered as simple sequences in bacterial genomes, the understanding of their function has evolved significantly.
The journey began with Yoshizumi Ishino's research, which laid the foundation for understanding the adaptive immune system in bacteria. This breakthrough suggested potential applications far beyond what was imagined in the 1980s.
"The ability of CRISPR systems to record viral infections in bacteria has changed our perspective on microbial immunity and evolution of microorganisms."
As research progressed, scientists like Jennifer Doudna and Emmanuelle Charpentier unveiled the CRISPR-Cas9 gene-editing tool, demonstrating CRISPR's versatility. The implications of their work continue to impact various fields, including medicine, agriculture, and environmental science. Researchers now harness CRISPR's precision to edit genes, aiming to cure genetic disorders and enhance crop resilience.
Looking Ahead to Future Innovations
The future of CRISPR research is filled with potential. Innovations are expected to expand its applications in medicine, agriculture, and synthetic biology.
Researchers are integrating CRISPR with other technologies, such as artificial intelligence, to optimize gene editing processes. This interdisciplinary approach promises more efficient and targeted treatments for complex diseases.
Moreover, the ethical considerations surrounding CRISPR will likely evolve. As society grapples with the implications of gene editing in humans and the environment, regulatory frameworks will need to develop concurrently to ensure responsible use.
In summary, CRISPR's journey has just begun. Its discovery as a basic immune mechanism in bacteria has transformed into a powerful tool that holds immense promise for future innovations in science and medicine.