Understanding Quantum Computers: Mechanisms Explained
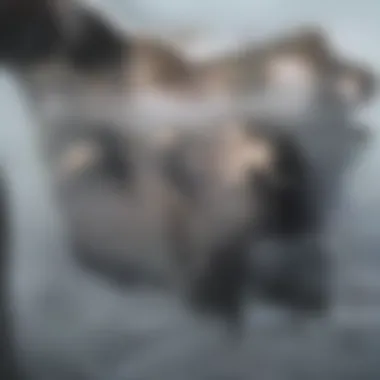
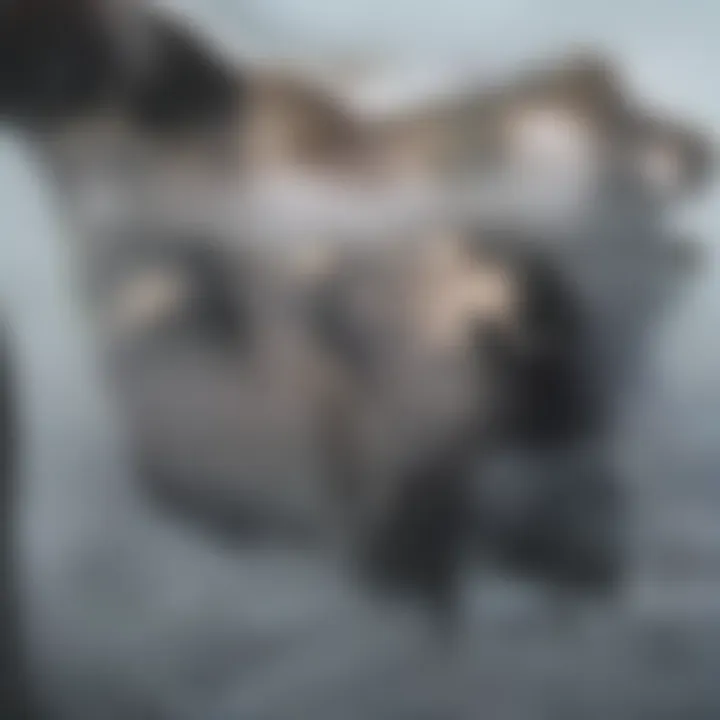
Intro
Quantum computing stands at the forefront of modern technology, heralding new possibilities across various fields. The exploration of quantum mechanics presents profound implications that diverge significantly from classical computing. While traditional computers use bits to represent information in binary form, quantum computers leverage qubits to manipulate data in ways that classical systems cannot replicate. The complexity behind these mechanics can be daunting, but understanding the basics provides a solid foundation for grasping this revolutionary field.
In this article, we aim to uncover the nuances of quantum computing, elaborating on its structural characteristics, algorithms, and real-world applications. We also consider the current hurdles and future prospects in quantum technology, giving the reader a well-rounded perspective on this exciting domain.
Key Research Findings
Overview of Recent Discoveries
Recent breakthroughs in quantum computing have expanded the potential of this technology greatly. Research shows that quantum supremacy, achieved by Google in 2019, marked a pivotal milestone. By solving specific problems faster than the most advanced classical supercomputers, it demonstrated the unique capabilities of quantum systems.
Furthermore, developments in quantum algorithms, such as Shor's algorithm for factoring large numbers, illustrate the potential for exponential speed-up in processing tasks. Other significant advancements include quantum error correction techniques that aim to address stability issues in qubits, ensuring that quantum states remain reliable for calculations.
Significance of Findings in the Field
The significance of these findings cannot be overstated. They present possibilities that may transform industries like cryptography, optimization, and drug discovery. As companies and research institutions like IBM, Microsoft, and D-Wave invest in quantum technology, the economic implications grow alongside technological advances.
"Quantum computing is not just about faster calculations; it's about solving problems previously deemed unsolvable."
- Anonymous Quantum Researcher
Breakdown of Complex Concepts
Simplification of Advanced Theories
To fully grasp quantum computing principles, simplification of its advanced theories is essential. Key concepts include superposition and entanglement. Superposition allows qubits to exist in multiple states at once, enabling parallel processing. Entanglement creates a link between qubits, making them interdependent. This interconnectedness is what leads to the potential exponential growth in processing power.
Visual Aids and Infographics
Visual aids can greatly enhance understanding. Diagrams depicting qubit arrangements and gate operations, for example, illustrate how quantum circuits operate. Infographics summarizing the differences in processing capabilities between classical and quantum computers can also assist in clarifying these complex ideas.
In summary, the mechanics of quantum computing rests on intricate principles that challenge our traditional understanding of computation. The findings discussed underscore the transformative potential of quantum technology. As we delve further into this subject, we will explore the architectures and algorithms shaping current quantum systems, as well as examine their real-world applications.
Preface to Quantum Computing
Quantum computing represents a landmark shift in how we process information. This section underscores the importance of understanding quantum computing as it introduces fundamental concepts that underpin what distinguishes quantum computers from classical systems. Recognizing the nuances of quantum mechanics is pivotal for grasping the workings of quantum computers and their potential applications in various fields.
The significance of quantum computing cannot be overstated. With traditional computers operating on binary bits, quantum computers leverage the peculiar principles of quantum mechanics. This allows them to simultaneously process a vast amount of possibilities. As we progress into an era rich with data, harnessing these unique computational abilities offers expansive potential in sectors like cryptography, medicine, and optimization.
Moreover, the discourse surrounding quantum computing brings forth critical considerations, including its implications for security, the economy, and advancing technology. Educators, researchers, and professionals delve into this area to not only understand quantum mechanics but to also explore its practical applications and theoretical underpinnings.
Definition of Quantum Computing
Quantum computing involves the study and application of quantum-mechanical phenomena, primarily focusing on the use of quantum bits or qubits. Unlike classical bits that can represent a value of either 0 or 1, qubits can exist in a state of superposition, meaning they can represent both 0 and 1 simultaneously. This property allows quantum computers to perform a multitude of calculations at once, enhancing computational efficiency for certain tasks.
In essence, quantum computing utilizes quantum states to encode information and perform operations. This enables calculations that classical computers might find infeasible, mandating a shift in how we approach complex problem-solving.
Historical Context
The evolution of quantum computing spans several decades and is intertwined with advancements in physics and computer science. The notion began forming in the early 1980s, with physicist Richard Feynman positing that a quantum system could not be accurately simulated using classical computing methods. This idea laid the groundwork for considering how quantum mechanics could be harnessed to create powerful computational models.
By the 1990s, the theoretical foundations were solidified further with Peter Shor's groundbreaking algorithm that demonstrated the potential of quantum computers in factoring large numbers exponentially faster than any known classical algorithm. Additionally, Lov Grover's search algorithm provided insights into how quantum techniques could optimize database searches and other computational tasks.
The journey from theory to practice accelerated in the 21st century with advancements in technology and a growing interest among researchers and commercial entities. Many startups and established companies now invest in quantum technologies, marking a transition towards practical applications. As these developments unfold, they continue to shape our understanding and expectations about the capabilities and implications of quantum computing.
Fundamental Principles of Quantum Mechanics
Understanding quantum computing begins with grasping the fundamental principles of quantum mechanics. These principles, while complex, provide the essential framework that distinguishes quantum systems from classical ones. They underpin every operation in quantum computers, making them capable of solving problems that would be untenable for classical computers.
The importance of these principles cannot be overstated. By comprehending how quantum superposition, entanglement, and measurement work, one can appreciate the power and potential of quantum computing. For instance, these principles allow quantum bits, or qubits, to perform multiple calculations simultaneously, a capability traditional bits simply cannot match.
Additionally, the integration of quantum mechanics into computing leads to advancements in various fields such as cryptography, material science, and optimization problems. Each principle opens the door to new possibilities and innovations. Thus, understanding these fundamental concepts is crucial for anyone seeking to navigate the landscape of modern technology.
Quantum Superposition
Quantum superposition is a foundational concept in quantum mechanics. It refers to the ability of a quantum system to exist in multiple states at once until it is measured. Unlike classical bits, which represent either a 0 or a 1, qubits can represent both states simultaneously. This property is what enables quantum computers to perform parallel computations.
Superposition can be likened to spinning a coin; the coin has a potential to be both heads and tails until observed. In practical terms, the effects of superposition allow quantum algorithms to process vast amounts of data more efficiently than classical algorithms.
Applications of this principle can be found in quantum algorithms like Grover's search algorithm, which allows for faster database searches than classical methods. Understanding superposition is essential for grasping how quantum computers handle information uniquely.
Quantum Entanglement
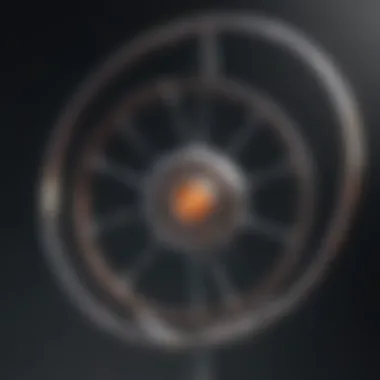
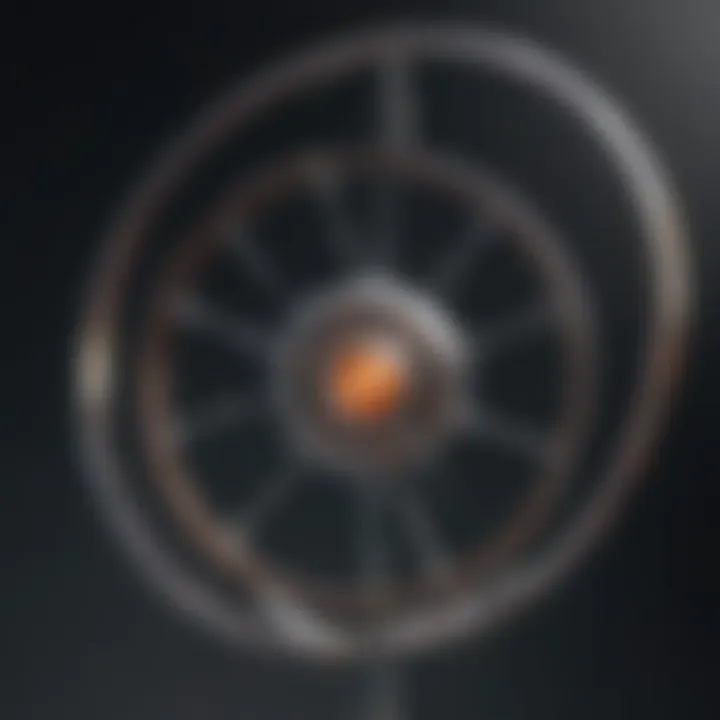
Quantum entanglement is another key principle of quantum mechanics. It describes a phenomenon where two or more qubits become interconnected, so the state of one qubit will instantly affect the state of the other, regardless of the distance separating them.
This instantaneous correlation has profound implications for quantum computing. Entangled qubits can be used to create highly complex quantum circuits. Also, this principle is fundamental in quantum cryptography, providing unprecedented security features due to the nature of entangled states.
Entanglement highlights how quantum computers can operate on data in ways classical systems cannot. By utilizing these entangled states, quantum computers can significantly improve their processing capabilities, making this understanding ever more critical.
Principle of Quantum Measurement
The principle of quantum measurement poses one of the most interesting challenges in quantum mechanics. When a qubit is measured, its superposition collapses to a definite state, either 0 or 1. This act of measurement, therefore, alters the system and leads to unique outcomes that are probabilistic in nature.
Understanding measurement is vital because it has implications for how quantum algorithms are designed. Quantum gates manipulate qubits in superposition, but the final output is only revealed upon measurement. This intrinsic uncertainty emphasizes the importance of probabilistic thinking in quantum computing processes.
Furthermore, quantum measurement leads to discussions about noise and decoherence, which are critical issues in building scalable quantum computers. Therefore, mastering the principles of quantum measurement is necessary for developing efficient and reliable quantum systems.
Quantum Bits (Qubits) Explained
Quantum bits, or qubits, form the core building blocks of quantum computing. In contrast to classical bits that can either be 0 or 1, qubits leverage the principles of quantum mechanics, enabling them to exist in multiple states simultaneously. This inherent ability significantly enhances computational power and efficiency, allowing quantum computers to solve complex problems at an unprecedented rate. Understanding qubits is essential as they introduce a paradigm shift in computation.
Qubit Definition and Functionality
A qubit operates as the fundamental unit of quantum information. It can represent both 0 and 1 at the same time, thanks to the principle of superposition. This characteristic allows qubits to perform numerous calculations simultaneously. When we manipulate qubits through quantum gates, we alter their states. Importantly, quantum measurements will collapse these multiple states into one observable outcome, which is crucial for computing tasks.
One interesting feature is that qubits can be entangled, meaning the state of one qubit can depend on the state of another, regardless of distance. This interconnectedness amplifies the computational capabilities of quantum systems, making qubits indispensable in quantum computing.
Types of Qubits
Different technologies are employed to implement qubits. These methods vary significantly in their principles, benefits, and challenges. Understanding these diverse types helps in appreciating their roles in quantum computing.
Superconducting Qubits
Superconducting qubits are one of the most widely explored methods. They utilize superconducting materials to generate and control quantum states. A key characteristic of superconducting qubits is their relatively larger coherence times compared to other types. This means they can maintain their quantum state longer, which is crucial for complex operations. These qubits are seen as popular choices because they can be integrated with existing technologies, making them easier to scale.
However, a unique feature is their sensitivity to noise, which can lead to errors in computations. Thus, error correction protocols are essential for superconducting qubits to ensure accurate results. Despite these challenges, their potential for scalability makes them vital in current quantum research.
Ion-trap Qubits
Ion-trap qubits rely on ions trapped in electromagnetic fields. The primary characteristic of ion-trap qubits is their precision control. Each trapped ion can be manipulated with laser beams, allowing for high-fidelity operations. This method offers impressive coherence times, enhancing computational reliability.
One unique aspect is the ability to entangle multiple ions easily, which is advantageous in performing complex calculations. However, ion-trap systems require sophisticated equipment and precise conditions, making them less accessible. Nevertheless, their reliability and precision contribute significantly to the advancement of quantum computing.
Topological Qubits
Topological qubits represent a novel approach to quantum computing, utilizing topology to protect quantum information. The main characteristic of these qubits is their robustness. They are less susceptible to local errors due to their unique properties. This feature positions them as a promising option for fault-tolerant quantum computation.
The unique feature of topological qubits lies in their braiding, where the paths of particles affect their states. This behavior can potentially lead to more stable qubits than other types. However, research is still ongoing in this area, and practical implementations are limited. Their potential for enhanced error resistance definitely marks them as an intriguing direction in the evolution of quantum systems.
Understanding these types of qubits provides critical insights into their respective advantages and limitations. Each type plays a role in addressing specific challenges within the broader framework of quantum computing, emphasizing the intricate mechanics at play.
Quantum Gates and Circuits
Quantum gates and circuits are fundamental components underpinning the operation of quantum computers. They play a pivotal role in how quantum information is processed and manipulated. Unlike classical gates that handle bits, quantum gates operate on qubits, allowing for the superposition and entanglement phenomena characteristic of quantum computing. Understanding these concepts is essential for grasping how complex quantum computations are performed.
Defining Quantum Gates
Quantum gates are the building blocks of quantum circuits. They are represented mathematically by unitary matrices that transform the state of qubits. Each gate executes a specific function by altering the quantum state within a circuit. This change is not just about flipping bits but involves complex transformations that enable multiple states to exist simultaneously. The precision and functionality of quantum gates directly influence the capacity of quantum computers to outperform their classical counterparts in various tasks.
Types of Quantum Gates
Single-Qubit Gates
Single-qubit gates focus on manipulating one qubit at a time. They are crucial for preparing and evolving quantum states in a controlled manner. The most common single-qubit gates include the Pauli-X, Pauli-Y, and Hadamard gates. Each has distinct functions and characteristics:
- Key Characteristic: They can rotate the state of a qubit across the Bloch sphere, a geometrical representation of quantum states.
- Benefit: Single-qubit gates are essential for initializing and measuring qubit states, making them vital for quantum algorithms.
- Unique Feature: The Hadamard gate, for instance, creates superposition, allowing a single qubit to represent both 0 and 1.
- Disadvantages: Errors can accumulate quickly, requiring error correction to maintain state fidelity over time.
Multi-Qubit Gates
Multi-qubit gates operate on two or more qubits simultaneously. They facilitate interactions between qubits, creating entangled states critical for quantum computation. Common examples are the CNOT (Controlled-NOT) gate and the Toffoli gate:
- Key Characteristic: They enable entanglement, allowing qubits to be interconnected, which is essential for leveraging quantum parallelism.
- Benefit: Multi-qubit gates enhance the computational capabilities of quantum circuits, enabling more complex computations than single-qubit operations alone.
- Unique Feature: The CNOT gate flips the state of a target qubit based on another qubit's state, creating correlations that are foundational in quantum algorithms.
- Disadvantages: They can be more challenging to implement due to the need for precise control over multiple qubits during operations.
Constructing Quantum Circuits
Constructing quantum circuits involves arranging quantum gates to perform specific computations. The sequence of operations must be carefully designed to achieve the desired outcome. This requires not only an understanding of how individual gates operate but also how they interact within a circuit.
To design a quantum circuit:
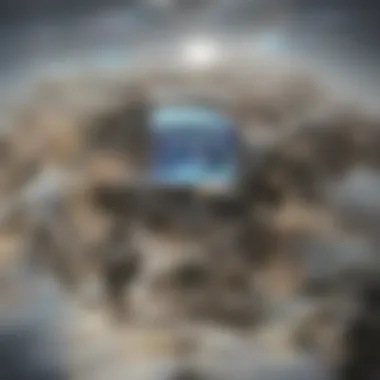
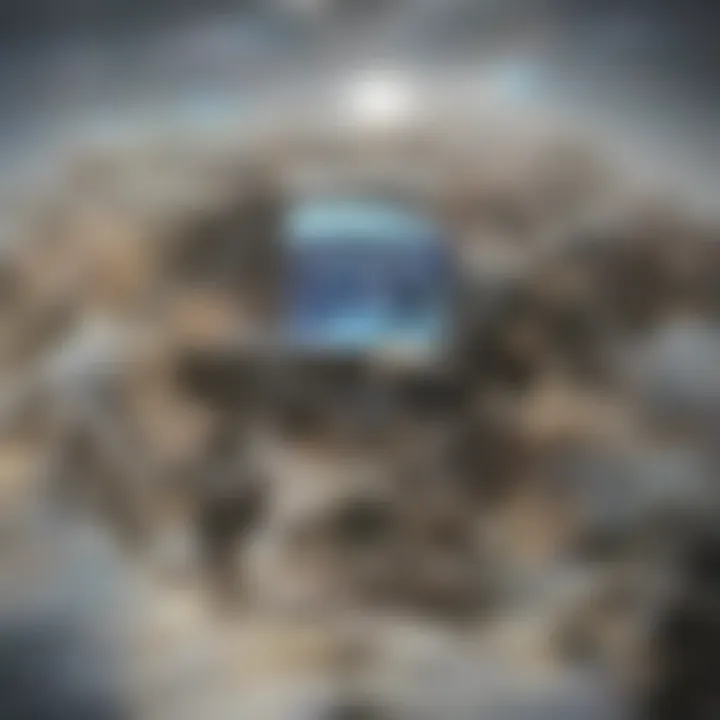
- Identify the Problem: Determine the specific computation or algorithm to be executed.
- Choose Gates: Select appropriate quantum gates that will perform the needed operations.
- Arrange Gates: Organize gates into a sequence that preserves the necessary dependencies between qubits.
- Test and Optimize: Simulate the circuit to identify any errors or inefficiencies and optimize accordingly.
Building quantum circuits effectively is critical to quantum computing, as the arrangement and choice of gates directly influence the performance and accuracy of the computations.
Quantum Algorithms
Quantum algorithms represent a pivotal element in the realm of quantum computing. They enable quantum computers to process information fundamentally differently than classical computers. Understanding these algorithms is crucial for appreciating how quantum systems can solve certain problems with extraordinary efficiency. The exploration of quantum algorithms is not merely an academic exercise; it is tied to practical advancements across various fields such as cryptography, database searching, and drug discovery.
Overview of Quantum Algorithms
Quantum algorithms are designed to leverage the unique properties of quantum mechanics. They often utilize quantum superposition and entanglement to achieve outcomes unattainable by classical computational methods. One of the standout benefits of quantum algorithms is their ability to reduce the time complexity of problem-solving. For instance, they can accomplish in seconds what might take classical counterparts years. This efficiency presents massive implications for industries relying on complex computations.
Key elements of quantum algorithms include:
- Quantum Speedup: Quantum algorithms can potentially provide significant speed advantages over the best classical algorithms.
- Parallelism: Due to superposition, quantum systems can explore multiple solution paths simultaneously.
- Problem-Specific Solutions: Certain algorithms are tailored for specific types of problems, indicating the need for developing a diverse set of methods for varied applications.
Given these capabilities, researchers and practitioners are enthusiastic about the possibilities quantum algorithms present in revolutionizing computation.
Shor's Algorithm
Shor's algorithm is a seminal example in the field of quantum computing, known for its application in factoring large integers. Specifically, it can solve the problem of integer factorization efficiently, where classical algorithms struggle significantly with larger numbers. The importance of Shor's algorithm transcends computational theory; it challenges the very foundations of current encryption methods that secure digital communications.
Essential impacts of Shor's algorithm include:
- Cryptography Vulnerability: Traditional encryption schemes, like RSA, could be broken using Shor's algorithm, necessitating the development of quantum-resistant encryption methods.
- Quantum Advantage: Demonstrates quantum computers achieving practical advantages under specific conditions.
The algorithm operates by efficiently finding the prime factors of a composite number utilizing quantum Fourier transform techniques. This reinforces the value of quantum algorithms in addressing real-world problems effectively.
Grover's Algorithm
In contrast to Shor's algorithm, Grover's algorithm focuses on searching unsorted databases. It offers a quadratic speedup over classical search algorithms. While classical algorithms require linear time to search through n items, Grover's algorithm can perform this in approximately โn time. This acceleration can be particularly beneficial in various applications, from cryptography to search engines.
Notable attributes of Grover's algorithm include:
- Versatility: Applicable in solving NP-complete problems and enhancing search operations.
- Practical Applications: Can be utilized in optimization problems and scenarios where efficient information retrieval is critical.
Grover's algorithm illustrates the transformative potential of quantum computing for practical applications, extending beyond theoretical realms into tangible utility.
Overall, the study of quantum algorithms is a crucial milestone in establishing a comprehensive understanding of quantum computing. They not only highlight the power of quantum systems but also underscore the urgent need for ongoing research and implementation across disciplines.
Applications of Quantum Computing
The applications of quantum computing are emerging as one of the most exciting aspects of this technology. Quantum computers hold the potential to revolutionize various fields by solving complex problems that are currently intractable for classical systems. They utilize the unique principles of quantum mechanics, such as superposition and entanglement, enabling breakthroughs across diverse sectors.
Some significant areas of application include:
- Cryptography
- Drug Discovery
- Optimization Problems
Each of these areas can benefit tremendously from quantum computing, offering enhanced efficiency and capabilities.
Cryptography
Cryptography is a fundamental aspect of digital security. Quantum computing introduces both threats and opportunities in this domain. Quantum computers are theoretically capable of breaking many of the encryption algorithms that underlie modern security protocols, like RSA and ECC. This is primarily due to algorithms like Shor's Algorithm, which can factor large numbers much more efficiently than classical algorithms.
Conversely, quantum computing also paves the way for quantum cryptography, particularly through Quantum Key Distribution (QKD). QKD allows two parties to generate and share a secret key in a way that exploits the principles of quantum mechanics, namely the vulnerabilities of quantum states to measurement. If an eavesdropper tries to intercept the key, the quantum state changes, indicating that the communication has been compromised.
Drug Discovery
In the pharmaceutical industry, the process of drug discovery traditionally involves extensive trial and error, often taking years to identify viable candidates. Quantum computing offers the capability to simulate molecular interactions with high precision and speed that classical computers cannot achieve. This ability significantly reduces the time required for drug development.
For instance, quantum computers can model complex molecules and chemical reactions to predict how new drugs can interact with targets, enhancing the accuracy of potential outcomes. Companies like D-Wave and IBM are already working on quantum algorithms aimed at accelerating drug discovery, evidencing the growing interest in this application.
Optimization Problems
Many real-world problems require optimization solutions, where the goal is to find the best solution among a huge set of possibilities. Industries such as logistics, finance, and manufacturing face challenges that can benefit from quantum optimization.
For example, in logistics, companies can benefit from improved route planning and resource allocation, enabling them to minimize costs and delivery times. Quantum algorithms can evaluate multiple routes simultaneously, which classical algorithms struggle to do efficiently.
Overall, the applications of quantum computing are vast and varied. As the technology matures, its impact on areas such as cryptography, drug discovery, and optimization problems could reshape industries, making processes more efficient and effective.
Challenges in Quantum Computing
Understanding the challenges in quantum computing is vital for grasping the complexities of this advanced technology. These challenges are not merely technical hurdles but also dictate the pace at which practical applications can be developed. Researchers and engineers in the field must navigate various obstacles, such as decoherence, scalability issues, and resource requirements. Addressing these challenges will enhance the reliability and efficiency of quantum systems, paving the way for broader adoption.
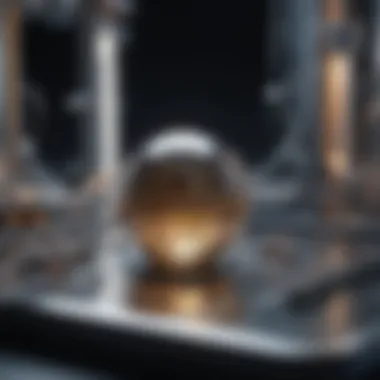
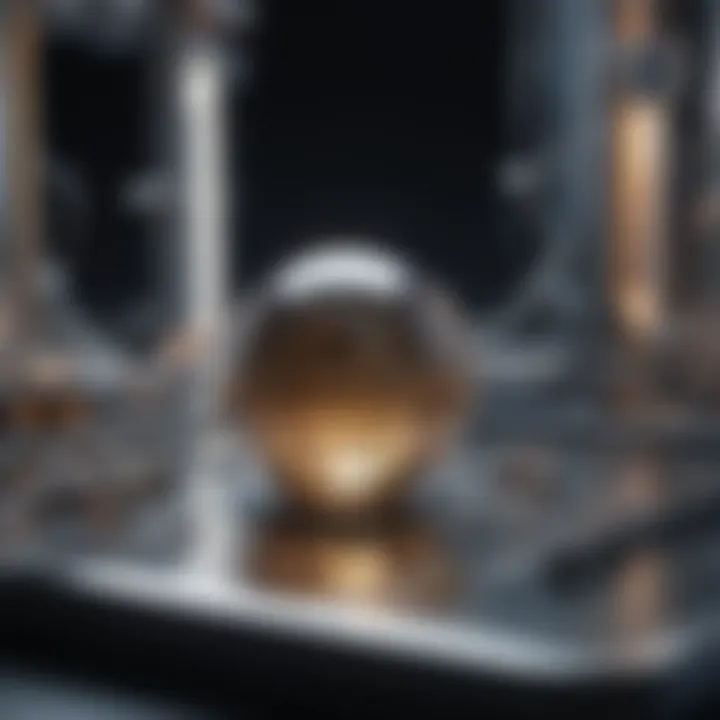
Decoherence and Error Rates
Decoherence occurs when a quantum state loses its coherence due to interaction with the external environment. This process is significant because it can lead to errors in quantum computations. The fragile nature of qubits means they can easily be disturbed, causing loss of information and incorrect results. Error rates in quantum computing are a major concern. High error rates can render quantum calculations unreliable, which is especially problematic for sensitive applications like cryptography and complex simulations.
To combat decoherence and manage error rates, various error correction techniques are being developed. These include using special codes and fault-tolerant architectures to protect information. Nonetheless, effective error correction increases the complexity and resource demands of quantum systems.
Scalability Issues
As quantum technology advances, scalability has emerged as a crucial issue. Building a quantum computer that can handle a significant number of qubits is still a challenge. Most existing quantum computers have a limited number of qubits, making them unsuitable for solving complex, real-world problems. Scalability is essential for moving from small-scale experiments to full-fledged quantum computing applications.
Developing scalable quantum systems involves not just improving the number of qubits but also ensuring that they can operate accurately and efficiently. Researchers are exploring various approaches, such as modular architectures and hybrid systems that combine classical and quantum resources. However, each approach presents its own set of difficulties, from maintaining coherence among a large number of qubits to managing physical infrastructure.
Resource Requirements
Quantum computing has specific resource requirements that can limit its accessibility and practicality. The need for advanced materials, cryogenic environments, and specialized hardware presents significant hurdles. For example, superconducting qubits require extreme cooling temperatures to function effectively. This translates to high operational costs and complex systems that may not be feasible for widespread use.
Furthermore, the talent pool for quantum computing is currently limited. There is a growing need for scientists and engineers who specialize in quantum mechanics, computer science, and related fields. Educating and training a new generation of professionals is essential to meet the demands of this emerging field and ensure continuous advancements.
"The realization of practical quantum computers will depend not only on technological advancements but also on addressing fundamental challenges that we face today."
These challenges in decoherence, scalability, and resource requirements are critical factors in determining the future of quantum computing. Overcoming them will provide the foundation for significant breakthroughs in various applications, from cryptography to complex algorithms.
Future Prospects of Quantum Computing
The future of quantum computing holds immense promise and importance. As we continue to delve into the capabilities of quantum mechanics, it becomes apparent that these machines could revolutionize various sectors. There are specific elements that make the exploration of future prospects essential. They include enhanced computational power, advancements in algorithms, and broader application scopes.
Research Directions
Ongoing research in quantum computing is diverse and multifaceted. Active areas of investigation include the development of new quantum algorithms, materials for better qubit performance, and methods for error correction. The pursuit of scalable quantum computing systems is a prominent research focus. Currently, various universities, research institutions, and private companies strive to figure out how to scale the technology while managing decoherence challenges. Understanding these aspects is vital. Future breakthroughs may yield significant improvements in processing capabilities and efficiency.
Some key research directions include:
- Quantum Error Correction: Creating robust systems that maintain integrity in computations despite error rates.
- Hybrid Quantum-Classical Systems: Exploring the integration of quantum computing with existing classical systems for enhanced performance.
- New Qubit Designs: Investigating novel approaches for creating stable and scalable qubits.
Research in these areas is critical for unlocking the potential of quantum computing.
Commercialization and Industry Impact
The shift toward commercialization of quantum technologies is evident. Several major tech companies, including IBM, Google, and Microsoft, have made substantial investments in this field. The impact of quantum computing on industry sector is set to be transformative.
As quantum systems become commercially viable, sectors such as finance, healthcare, and logistics can expect significant efficiency improvements. For instance, quantum computing can optimize complex logistics systems, significantly reducing operational costs. Additionally, breakthroughs in pharmaceuticals can lead to faster drug discoveries through molecular simulations that classical computers struggle to perform.
The timeline for commercialization will depend on overcoming several hurdles, particularly related to technology scalability and stability. Nevertheless, forecast indicate that industries focusing on quantum capabilities will gain a competitive edge. This potential will drive more companies to invest in quantum research and talent acquisition, further stimulating growth in the quantum computing ecosystem.
"Quantum computing has the potential to change how we solve some of the most complex problems in our world."
Ending
The conclusion of this article serves to emphasize the significance of quantum computing within the broader context of technological advancement. As we have explored, quantum computers operate on the fundamental principles of quantum mechanics, utilizing unique properties like superposition and entanglement. Understanding these principles not only enhances our grasp of future technologies but also highlights their potential impact across various fields.
Summary of Key Points
- Definition and Importance: Quantum computing harnesses the principles of quantum mechanics to perform computations far beyond the scope of classical computers. This difference enables solutions to problems previously thought insurmountable.
- Applications: Quantum computers have profound implications in areas such as cryptography, drug discovery, and optimization problems. These applications illustrate the transformative power of quantum technologies in addressing real-world challenges.
- Challenges: The journey toward practical quantum computing is fraught with challenges including decoherence, scalability, and resource requirements. Understanding these obstacles is essential for advancing this field.
- Future Prospects: As research continues, the commercialization and industry impact of quantum computing is likely to expand. Embracing these developments is critical for anyone involved in technology.
Final Thoughts on Quantum Computing
"The future of quantum computing is not just a theory; it is an emerging reality that reshapes our understanding of what computers can achieve."
By being aware of the intricacies and implications of quantum computing, stakeholders can navigate the future landscape effectively. It will be essential to engage with this technology as it continues to mature, promising not only innovation but also greater capabilities in processing complex information.
Further Reading
Further reading is crucial in the realm of quantum computing. This topic explores the vast amounts of information available that can deepen understanding and expand knowledge. As quantum technologies rapidly evolve, it becomes essential for students, researchers, educators, and professionals to stay informed. Accessing reliable sources enhances learning and assists in grasping advanced concepts.
Books on Quantum Computing
Books provide structured information and comprehensive insights into quantum computing. They can range from introductory texts to advanced discussions. Some notable books include:
- Quantum Computation and Quantum Information by Michael A. Nielsen and Isaac L. Chuang. This text is often considered the foundational book in iqantum computing. It covers a wide range of topics with clarity, making complex ideas accessible.
- Quantum Computing for Computer Scientists by Noson S. Yanofsky and Mirco A. Mannucci. This book bridges the gap between quantum mechanics and computer science. It is suitable for computer scientists looking to understand quantum theory's implications for the field.
- Quantum Computing: A Gentle Introduction by Eleanor Rieffel and Wolfgang Polak. This is a beginner-friendly guide. It introduces key concepts without requiring extensive background in physics or mathematics.
In addition to these titles, readers should explore specialized books focusing on specific algorithms or applications, as these can provide more in-depth perspectives.
Recent Research Articles
Keeping up with recent research articles is vital for anyone interested in the cutting edge of quantum computing. The scientific community frequently publishes work detailing advancements in the field. A few prominent journals include:
- Nature: Known for high-impact research, many groundbreaking quantum computing articles are published here.
- IEEE Transactions on Quantum Engineering: This journal focuses on the engineering aspects of quantum systems, making it suitable for practitioners.
- Physical Review Letters: A key publication for physicists, often including significant findings related to quantum mechanics and computing.
Additionally, platforms like arXiv offer preprints of research articles, allowing access to the latest findings before formal publication. This is essential for ongoing education and understanding future trends.
Staying informed through further reading not only enhances knowledge but also contributes to the broader conversation surrounding quantum computing, encouraging innovation and development in this promising field.