Understanding Neuron Microscopes for Neuroscience Advances
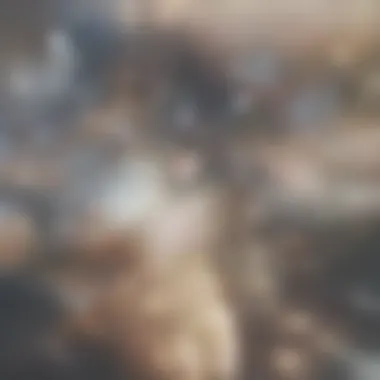
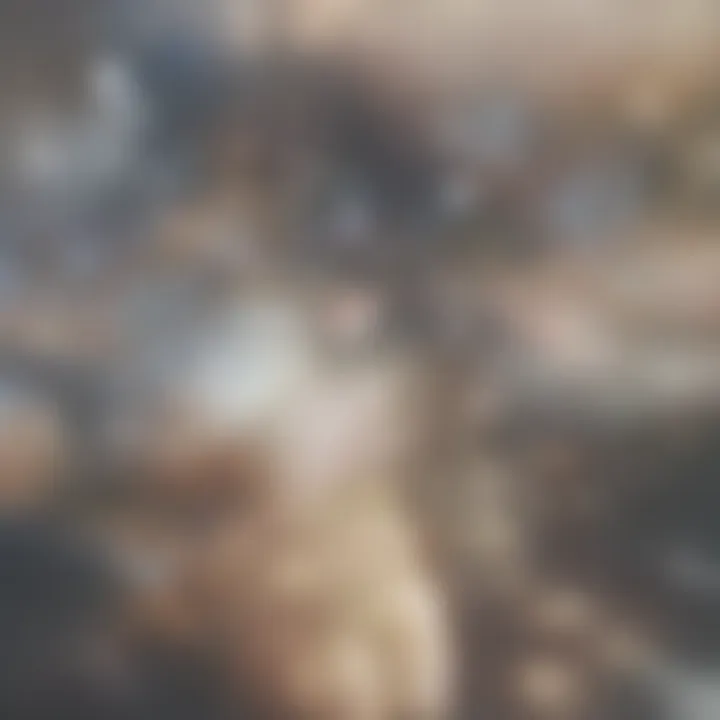
Intro
The investigation of neurons has become a central concern in modern neuroscience. Neuron microscopes play a vital role in visualizing the intricate structures and functions of these cells. By enhancing our view of neurons, we gain insights into how they operate and interact. This knowledge is essential for understanding both normal brain functions and various neurological disorders. Advances in microscopy are not only improving imaging techniques but also enhancing our capacity for research and discovery in this field.
Key Research Findings
Overview of Recent Discoveries
Recent advancements in neuron microscopy have led to significant discoveries that illuminate previously opaque aspects of neural function. Techniques such as super-resolution microscopy have allowed researchers to observe fine details of neuronal connections at a molecular level. This has resulted in uncovering how synapses are formed and how they facilitate communication between neurons. Studies have shown that the plasticity of these connections is crucial for learning and memory, impacting our understanding of cognitive processes.
Significance of Findings in the Field
The findings from neuron microscopy extend far beyond academic interest. They have direct relevance to therapeutic interventions for neurological diseases like Alzheimerโs and Parkinsonโs. For example, understanding the structural integrity of synapses may influence the development of drugs that target these connections. Moreover, identifying pathological changes in neurons aids in early detection of disease progression, improving patient outcomes.
"Microscopy allows us to visualize the unseeable, bringing clarity to the once hidden realms of neural networks."
Breakdown of Complex Concepts
Simplification of Advanced Theories
Microscopy techniques can be complex, particularly as they evolve into more advanced forms. Techniques like fluorescence microscopy and electron microscopy require a good grasp of physics and biology. However, breaking down these theories into simpler components makes them more accessible. For example, rather than focusing on the intricate details of photon interaction, one can concentrate on the outcomes these interactions produce in neuronal imaging.
Visual Aids and Infographics
Incorporating visual aids is beneficial for understanding these complex methodologies. Diagrams illustrating the layers of microscopy can demystify the processes involved. Infographics showing how different techniques compare in terms of resolution and application can clarify the practical aspects of neuron imaging.
In essence, neuron microscopes represent a confluence of cutting-edge technology and intricate biology. Their contributions to our understanding of neural mechanisms cannot be overstated. The implications of this knowledge span health, education, and many other fields, paving the way for future advances in neuroscience.
Preamble to Neuron Microscopy
Neuron microscopy serves an essential role in elucidating the complex architecture and functions of neural systems. Understanding this specialized field of microscopy is crucial for unraveling mysteries related to the brain and nervous system. With advancements in imaging technologies, researchers can now explore neuronal cells at a level of detail previously unimaginable.
Key elements of neuron microscopy include the application of various imaging techniques, including light and electron microscopy, which allow for distinct views of neuronal structures. The benefits of these methodologies can be profound, providing critical insights into how neurons operate and interact within their environments.
Moreover, the relevance of this topic extends beyond basic research. Neuron microscopy enables the study of neuronal behavior in disease contexts, offering avenues for therapeutic interventions in conditions like Alzheimer's disease, Parkinson's disease, and other neurodegenerative disorders.
As the field evolves, neuron microscopy incorporates increasingly sophisticated techniques that improve resolution and contrast, shedding light on synaptic functions and the dynamic processes of neuronal connections. Today, understanding neuron microscopy is not just for specialists; it serves to bridge the gap between neuroscience and broader biomedical disciplines, making it vital for students, researchers, and educators alike.
Defining Neuron Microscopy
Neuron microscopy is the branch of microscopy focused on the imaging of neurons and their networks. By using a variety of advanced techniques, researchers can visualize the intricate details of neuronal structures at microscopic scales. Key components of neuron microscopy include methods like light microscopy, electron microscopy, and fluorescence imaging.
The main objective is to capture high-resolution images of neuronal componentsโsuch as dendrites, axons, and synapsesโthat play a direct role in brain function. Fluorescent tags and stains often assist in highlighting specific neuronal structures, enabling scientists to differentiate among various types of neurons and their interconnections.
Historical Perspective
The history of neuron microscopy is rich, tracing back to the foundational developments in microscopy itself. The earliest microscopes, created in the 17th century, laid the groundwork for later advancements in understanding cellular structures. A significant milestone occurred in the 19th century with the formulation of the neuron doctrine, which proposed that neurons are individual cells rather than interconnected fibers.
As technology progressed, numerous breakthroughs occurred. Electron microscopy emerged in the 1930s, allowing researchers to examine intracellular structures with unprecedented clarity. This transition paved the way for examining synaptic connections and cellular interactions in real-time. The development of vital stains and fluorescent markers in the latter half of the 20th century provided further advancement, enabling researchers to visualize neurons with even greater specificity.
Today, the combination of historical knowledge and modern technological innovations makes neuron microscopy a critical tool in neuroscience research, guiding experiments that delve into the complexity of brain function and disease. A deeper understanding of these foundational aspects will set the stage for exploring more advanced topics in microscopy.
Basic Principles of Microscopy
Understanding the basic principles of microscopy is essential for appreciating how neuron microscopes function and their applications in neuroscience. This section breaks down the fundamental concepts that guide microscopy practices.
Light and Electron Microscopy
Light microscopy and electron microscopy are two primary techniques.
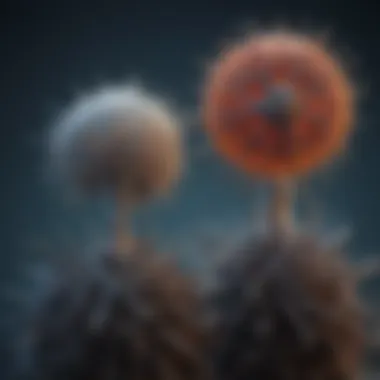
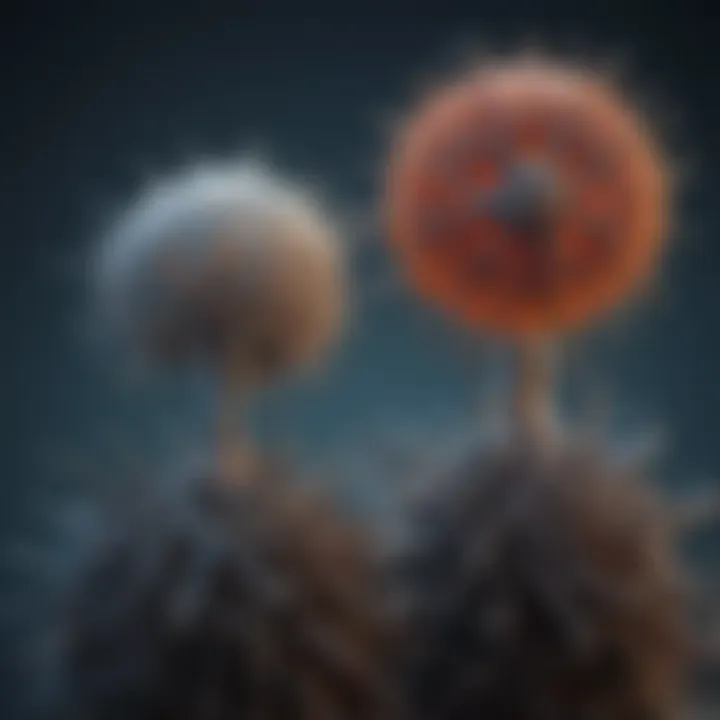
- Light Microscopy: This method uses visible light to illuminate samples. Light microscopes can magnify specimens up to 1,000 times. They allow for the observation of living cells and tissues, which is crucial in neurobiology. However, their resolution is limited by the wavelengths of light used.
- Electron Microscopy: Unlike light microscopy, electron microscopy employs a beam of electrons to create an image. This approach offers much higher resolution, up to 1 million times. It allows researchers to visualize cellular structures at a nanometer scale. However, the samples need to be prepared in a way that usually kills them, limiting the observation of live processes.
Understanding both types of microscopy helps researchers choose the right technique based on their specific needs in neuron imaging.
Fluorescence Microscopy Techniques
Fluorescence microscopy has gained popularity in studying neurons. This technique uses fluorescent dyes that emit light when excited by a light source. The key advantages include:
- Targeting Specific Structures: Researchers can use different fluorescent markers to label specific proteins or cellular structures in neurons. This selective staining allows for detailed visualization of neuronal components.
- Real-Time Observation: Fluorescence microscopy can be used to visualize dynamic processes in live neurons. This capability is valuable for understanding neuron function and behavior.
Techniques such as confocal microscopy can further enhance fluorescence imaging by improving resolution and reducing background noise, making it particularly useful in complex tissues.
Imaging Resolution and Contrast
Imaging resolution and contrast are critical factors in microscopy that influence the clarity of images obtained.
- Resolution refers to the ability to distinguish between two close points in an image. It directly affects the level of detail visible in neuronal structures. Higher resolution is necessary to see small organelles within neurons.
- Contrast is what allows differences in light intensity in the specimen to be observed. High contrast is vital for visualizing the intricate details of neuron structures against their backgrounds. Techniques like staining and using specific microscopy systems can enhance contrast dramatically.
Both resolution and contrast are essential for accurate interpretation of images in neuroscience research.
In summary, understanding the basic principles of microscopy unveils the tools and processes that underpin neuron imaging. It lays the groundwork for exploring more advanced techniques and applications in subsequent sections.
Technological Advances in Microscopy
Technological advancements in microscopy are crucial to the study of neurons. These innovations improve imaging techniques, allowing researchers to delve deeper into the intricate workings of the brain. By enhancing resolution and imaging capabilities, these advancements enable the visualization of neuronal structures with unprecedented clarity. Consequently, they contribute significantly to both basic neuroscience and medical research.
Confocal Microscopy Innovations
Confocal microscopy has transformed how scientists observe biological specimens. It utilizes a laser system to scan samples point by point, collecting images in a controlled manner. This approach allows for the acquisition of high-resolution images while minimizing background noise. The result is enhanced contrast and detail in the visualization of neuronal components.
Key innovations in confocal microscopy include:
- Multi-channel imaging: This permits the simultaneous observation of different cellular structures, facilitating the study of complex interrelations within neurons.
- Time-lapse imaging: Researchers can monitor dynamic processes over time, capturing real-time changes in neuronal morphology and function.
- Advanced software algorithms: These tools assist in image processing and reconstruction, enabling clearer and more informative outputs.
This technology is invaluable in understanding the brain's architecture and function, leading to new insights into neurobiology.
Super-Resolution Microscopy Breakthroughs
Super-resolution microscopy represents a leap beyond traditional optical resolution limits. Techniques such as STORM (Stochastic Optical Reconstruction Microscopy) and PALM (Photo-Activated Localization Microscopy) allow for imaging at the nanometer scale. Through innovative methods, these techniques circumvent diffraction limits of light, enabling visualization of microscopic structures at unmatched resolution.
Some important breakthroughs include:
- Nanoscopic imaging: This facilitates the observation of sub-cellular structures, essential for understanding synaptic organization and neuronal connections.
- Live-cell imaging: Researchers can study biological processes in real-time, providing insights into synaptic plasticity and cellular signaling mechanisms.
- Multiplexing capability: These methods allow for the simultaneous tracking of multiple molecules, enhancing studies on protein interactions within neurons.
This enhanced resolution is fundamental for both basic research and therapeutic development, given its implications for revealing the details of neuronal behavior and pathology.
Two-Photon Microscopy Applications
Two-photon microscopy has become an essential tool in neuroscience research. It uses two photons of lower energy to excite fluorescent molecules, allowing deep tissue imaging with minimal photodamage. This is particularly relevant for studying live neuronal tissue and complex brain structures in their native environments.
Key applications of two-photon microscopy include:
- Deep tissue imaging: It can penetrate living tissues up to a millimeter, providing views of neuronal circuits in a context relevant to their in vivo environments.
- Functional imaging: Researchers can investigate neuronal activity through calcium indicators, which reveal insights into synaptic events and action potentials.
- Disease modeling: This technology aids in visualizing changes in neuronal circuitry associated with disorders such as Alzheimerโs and Parkinsonโs disease, contributing to understanding their pathophysiology.
The advancement of two-photon microscopy is significant for unveiling previously hidden aspects of neuronal function. It reflects the continual evolution of imaging techniques that push the boundaries of neuroscience, aiming for improved treatments and understanding of neurological conditions.
"Technological innovations in microscopy are not merely tools; they are critical enablers for groundbreaking discoveries in neuroscience."
In summary, the technological advances in microscopy not only enrich our understanding of neuron structures but also allow for the exploration of complex neuronal functions. As research progresses, these tools will likely uncover new therapeutic avenues in neuroscience.
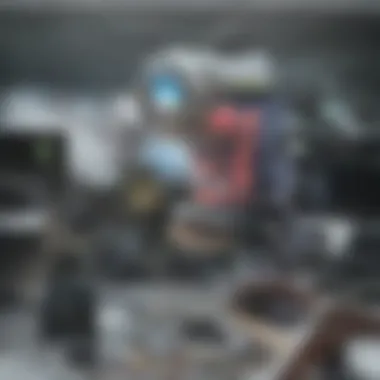
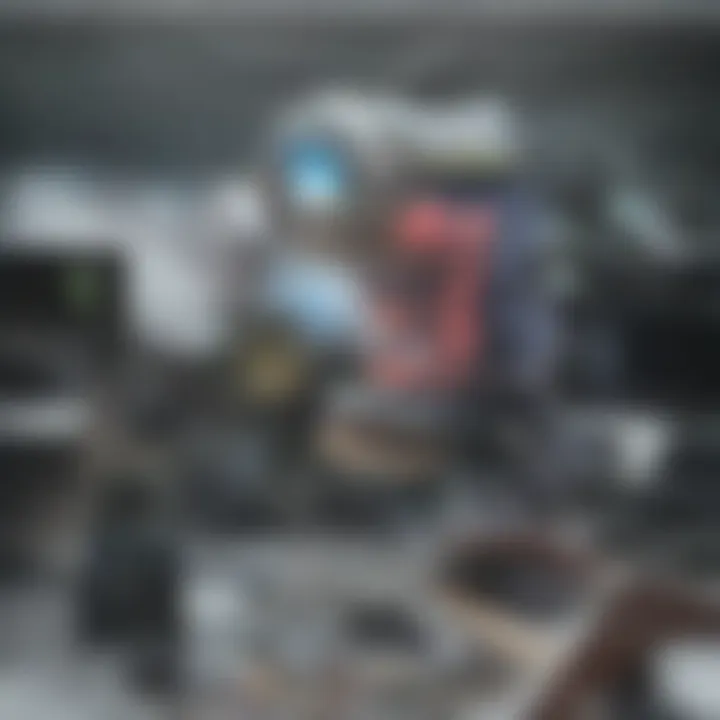
Neuron Structure and Imaging
Understanding neuron structure and imaging is essential for comprehending the complexities of the brain and its functions. Neurons are the fundamental building blocks of the nervous system, and their intricate structures reveal how they communicate, process information, and respond to stimuli. Imaging techniques aimed at visualizing neurons provide critical insights into both normal and pathological states, making this topic invaluable for researchers and clinicians alike.
Significance of Neuron Imaging
Neuron imaging holds great significance in various fields of neuroscience. It enables researchers to observe real-time changes in neuronal structures and functions. By applying advanced imaging techniques, scientists can study how neurons develop, how they communicate with each other, and how diseases may alter their normal functions. This is particularly crucial in understanding neurodegenerative disorders, where early detection and intervention can significantly influence patient outcomes. The insights gained from imaging studies help guide therapeutic strategies and drug development.
Moreover, neuron imaging aids in unraveling neural circuitry, which is vital in understanding cognition and behavior. Through imaging, scientists can uncover how neuronal connections support various brain functions, from sensory processing to decision-making. Thus, neuron imaging is not just a tool; it is a key that unlocks critical understanding of brain operations and its disorders.
Visualization of Neuronal Components
Visualizing the components of neurons is an intricate process, yet it gains importance as it provides a window into the cells' inner workings. Neurons consist of multiple parts: dendrites, axons, soma, and synaptic terminals. Imaging techniques such as fluorescence and electron microscopy allow researchers to visualize these components with high precision.
For instance, fluorescence microscopy lets scientists tag specific proteins or structures within neurons, highlighting their functions and interactions. This visualization assists in identifying how neurons operate and adapt over time. Additionally, with techniques like transmission electron microscopy, researchers can achieve nanoscale resolution, revealing details of synaptic structures that are crucial for understanding synaptic strength and plasticity. The detailed visualization of neuronal components is thus essential for discerning the mechanisms behind both typical functioning and disrupted states of neurons.
3D Reconstructive Imaging Techniques
3D reconstructive imaging techniques have revolutionized how scientists view neuronal structures. Unlike traditional 2D imaging, 3D reconstruction allows for a more accurate representation of neuronal form and spatial relationships. This technique is particularly beneficial in understanding complex neural networks.
Using methodologies like confocal microscopy or diffraction-limited 3D imaging, researchers can create three-dimensional models of neurons. These models enable the assessment of neuronal morphology and connectivity in far greater detail. Systems such as light-sheet fluorescence microscopy allow scientists to visualize entire neurons or even groups of neurons while maintaining their spatial context.
The power of 3D imaging lies in its ability to provide insights into how neurons interact on a larger scale. Understanding the spatial arrangement and connectivity among neurons is crucial for modeling brain functions and studying neurological diseases. This goes beyond mere structure but encompasses the essence of neural communication and integration.
"The future of neuroscience depends on our ability to visualize and understand neuronal networks and their complex interactions."
For further information on neuron structure and the latest imaging techniques, you may find this link helpful.
Applications of Neuron Microscopy in Research
Neuron microscopy plays a vital role in pushing the boundaries of our understanding of the nervous system. It provides the tools that enable researchers to visualize, measure, and analyze the intricate structures and dynamics of neurons. This section will cover the critical applications of neuron microscopy, highlighting various areas of focus such as neurodevelopmental studies, neurodegenerative disease research, and synaptic plasticity investigations.
Neurodevelopmental Studies
In the field of neurodevelopment, neuron microscopy facilitates the investigation of the formation and maturation of neuronal networks. The early stages of brain development are complex. Missteps during these stages can lead to serious conditions such as autism or intellectual disabilities.
Using advanced imaging techniques like confocal and two-photon microscopy, researchers can visualize the processes behind neuronal differentiation and connectivity. For instance, scientists can track the growth and pathfinding of axons. Additionally, fluorescence imaging allows for the observation of specific cell types and signaling pathways during development.
Understanding these processes is crucial for identifying the underlying mechanisms of neurodevelopmental disorders. This knowledge can also guide the development of potential therapies. As such, neuron microscopy has become indispensable for researchers studying the delicate progressions of neural development.
Neurodegenerative Disease Research
Neuron microscopy offers profound insights into neurodegenerative diseases like Alzheimer's and Parkinson's. These diseases often manifest through specific alterations in neuronal structure and function. Detecting these changes at an early stage is essential for effective intervention.
By employing techniques customarily found in neuron microscopy, researchers can observe pathologies such as amyloid-beta plaque formation and tau tangles in Alzheimer's disease. Imaging technologies can also reveal synaptic loss and neuronal death over time. Such capabilities equip scientists with the necessary information to formulate targeted drug therapies and novel treatment strategies.
Moreover, microscopy adds value in preclinical trials by helping to assess the efficacy of potential drugs and treatments on live neuronal tissues. The meticulous insights offered through imaging are crucial for translating research findings into clinical settings.
Synaptic Plasticity Investigations
Synaptic plasticity refers to the capacity of synapses to strengthen or weaken over time, in response to increases or decreases in their activity. This fundamental process underlies learning and memory. Neuron microscopy serves an essential function in studying synaptic plasticity by enabling the visualization of synapse dynamics in real time.
Techniques like electrophysiology combined with high-resolution imaging allow for examining synaptic changes at the molecular level. Researchers can label specific proteins and track changes in their localization and abundance as synaptic activity occurs. This data helps build a clearer picture of how neurons adapt and communicate over time.
Understanding synaptic plasticity has deep implications for numerous neurological conditions. Disorders such as schizophrenia and depression are linked with improper synaptic functioning. Therefore, neuron microscopy is a powerful tool for exploring potential treatments that could restore normal synaptic connections.
In summary, the applications of neuron microscopy are vast and varied. They extend across many disciplines within neuroscience, offering critical perspectives on developmental, degenerative, and functional processes in the brain. As technology continues to evolve, neuron microscopy will undoubtedly uncover more mysteries of the brain and its intricate workings.
Challenges in Neuron Microscopy
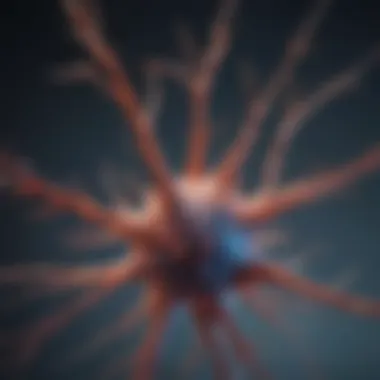
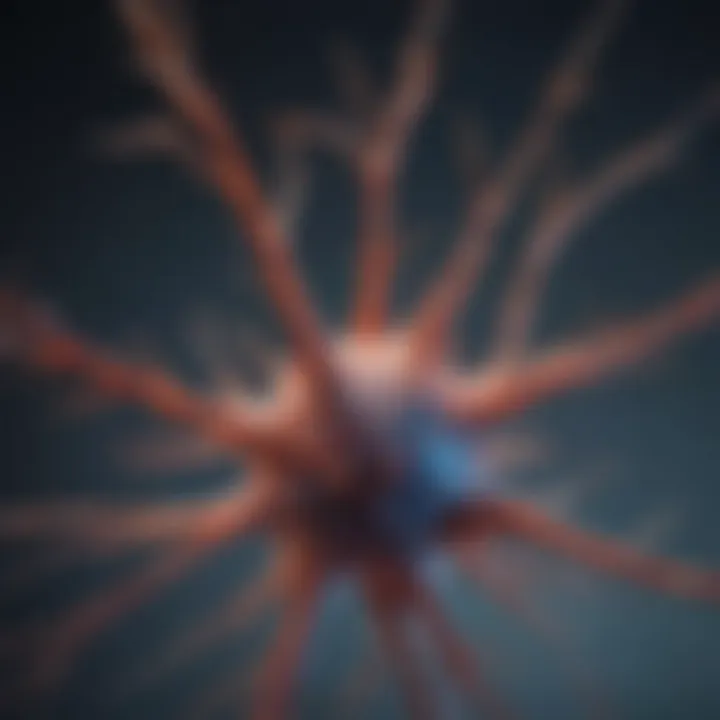
The field of neuron microscopy faces several challenges that can hinder effective imaging and analysis of neural structures. These challenges are critical because they affect both the quality of the data acquired and the interpretations that can be drawn from it. Understanding these challenges benefits researchers, educators, and professionals alike, as it can guide the development of improved methodologies and technologies in the future.
Technical Limitations
Technical limitations in neuron microscopy arise from various factors related to the equipment and methodologies employed. Common limitations include:
- Resolution and Contrast: Microscopes have limits on the resolution they can achieve, which may obscure crucial details within neuron structures. For example, optical diffraction limits constrain light microscopy, making it difficult to visualize very small neuronal components.
- Sample Preparation: The preparation of samples can introduce artifacts or alter neuronal structures, impacting the accuracy of the imaging results. Fixation and staining protocols must be carefully optimized to minimize these effects.
- Imaging Speed: In dynamic processes such as synaptic transmission, capturing rapid events can be difficult. Fast scanning rates are often needed, but they can lead to reduced signal-to-noise ratios, complicating data interpretation.
- Depth Limitation: When imaging thick tissue samples, like whole brain sections, it can become challenging to obtain high-quality images due to light scattering. This affects the ability to reconstruct accurate 3D models of neuronal architecture.
Addressing these limitations often requires advances in imaging technologies, including the development of novel microscopy techniques that can bypass traditional resolution barriers.
Biological Variability in Imaging
Biological variability presents another set of challenges in neuron microscopy. Neurons exhibit diversity in morphology and function, which can result in inconsistencies when trying to generalize findings. Key points to consider include:
- Species Differences: Different organisms may exhibit distinct neuronal structures. Thus, insights gained from one species may not be fully applicable to another, complicating comparisons and translations of findings.
- Individual Variability: Even within a single species, genetic differences and environmental factors can lead to variability in neuronal characteristics. Such factors may affect experimental outcomes and complicate replicability of results.
- Cell Type Specificity: Neurons are highly specialized, with various types exhibiting different morphological and functional properties. This specificity poses challenges when attempting to standardize imaging protocols to study neurons as a whole.
To mitigate these issues, researchers are increasingly employing integrative approaches, combining data from various sources and using computational models to account for biological variability in their analyses.
Understanding both technical limitations and biological variability is essential for advancing neuron microscopy and improving the reliability of results.
Future Directions in Neuron Microscopy
The field of neuron microscopy is evolving continuously. As it progresses, we see the introduction of more advanced techniques and technologies. This section discusses the importance of these future directions. Advancements could open new pathways in neuroscience, increasing our understanding of neuronal functions and interactions.
Ongoing research focuses on addressing limitations of current imaging technologies. Emerging techniques promise improved resolution and detail. The goal is to visualize neuronal connections more clearly. Research also concentrates on real-time imaging. This means that scientists could observe processes as they happen, allowing for a more dynamic understanding of neuronal behavior.
Emerging Techniques and Technologies
Emerging techniques in neuron microscopy are addressing existing challenges while also providing new insights. For example, developments in light-sheet microscopy allow researchers to view living tissue without damaging it. This method shines light on specific planes of samples, reducing phototoxicity.
Optogenetics combined with advanced imaging techniques, like functional magnetic resonance imaging, is also on the rise. This integration allows neuroscientists to manipulate neuronal activity and observe the effects in real-time. Additionally, advanced machine learning algorithms are improving image analysis. These algorithms can detect patterns and anomalies that the human eye may miss.
Key Emerging Technologies:
- Light-sheet Microscopy: Reduces damage to living cells while achieving high resolution.
- Optogenetics: This is vital for studying how neurons interact. It allows control of neurons and can aid in understanding complex neural networks.
- Super-Resolution Techniques: Methods like STORM and PALM enhance resolution beyond the diffraction limit.
These developments indicate a shift toward more integrated and flexible tools, opening new avenues for exploration and research.
Integrative Approaches in Neuroscience
As neuroscience becomes increasingly interdisciplinary, integrative approaches are crucial. Combining microscopy with other techniques enhances understanding of neural systems. For instance, integrating genetic engineering with imaging provides a fuller picture of neuronal function.
Collaboration between fields like bioinformatics and engineering is also important. By using computational techniques alongside microscopy, clearer insights into vast amounts of neural data can be achieved. This integrative method enhances data analysis, allowing for better interpretations of findings.
Additionally, the shared data approaches across institutions can foster innovation. Open access to imaging data can lead to collaboration. This, in turn, can facilitate the development of new hypotheses and experimental designs.
In summary, future directions in neuron microscopy promise significant advancements. Emerging techniques and integrative approaches will help to expand our understanding of the complex world of neurons. As the field moves forward, the impact of these innovations could lead to breakthroughs in the study of neural systems and diseases.
Closure
In summation, this article emphasizes the profound significance of neuron microscopy within the field of neuroscience. This complex branch of study plays a crucial role in advancing our understanding of neural structures, communication, and pathologies. Through various imaging techniques and innovative technologies, researchers are able to unveil intricate details about neurons that were previously obscured.
Summary of Key Insights
The importance of neuron microscopy cannot be overstated. Key insights from this article include the following:
- Diverse Techniques: The exploration of light microscopy, electron microscopy, and fluorescence techniques have allowed for a multi-faceted approach to studying neurons. Each method carries unique advantages, enabling researchers to visualize specific cellular components or processes.
- Technological Evolution: The rapid advancement in microscopy technology has led to breakthroughs in imaging resolution and depth of insights. Super-resolution and two-photon microscopy stand out as significant developments that enhance our ability to study live neurons.
- Applications in Research: Neuron microscopy is not only relevant in basic research but also in practical applications. This includes neurodevelopmental studies, investigations of neurodegenerative diseases, and the examination of synaptic plasticity.
- Challenges and Considerations: While powerful, neuron microscopy faces certain challenges such as technical limitations and biological variability, which researchers need to navigate carefully.
The Importance of Advancing Neuron Microscopy
The ongoing enhancement of neuron microscopy techniques is vital for several reasons. The continuous improvement fosters a deeper understanding of the neuronal mechanisms involved in health and disease. Advancing these methodologies will facilitate:
- Improved Imaging: Ongoing refinement in technologies leads to better imaging quality, enabling us to see structures and processes that were once indistinguishable.
- Integration with Other Disciplines: The advancement of neuron microscopy can also lead to integrative strategies within neuroscience, improving how various aspects of neural functioning are understood collectively.
- Therapeutic Insights: As researchers gain clearer images of neuron behavior and structure, the potential for therapeutic development increases. This may shape future treatment paths for neurological disorders.
- Educational Impact: New techniques translate into enhanced educational tools for students and professionals in the field, facilitating a richer learning experience.
"The advancements in neuron microscopy provide tools that are essential for groundbreaking discoveries in understanding the brain's complexity."