Understanding Google Quantum Computing Revolution
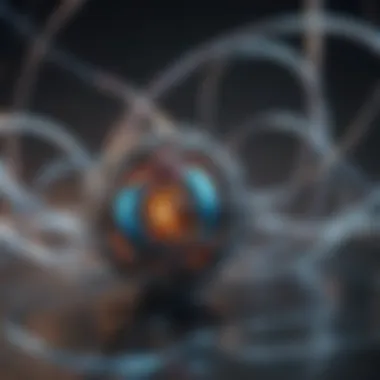
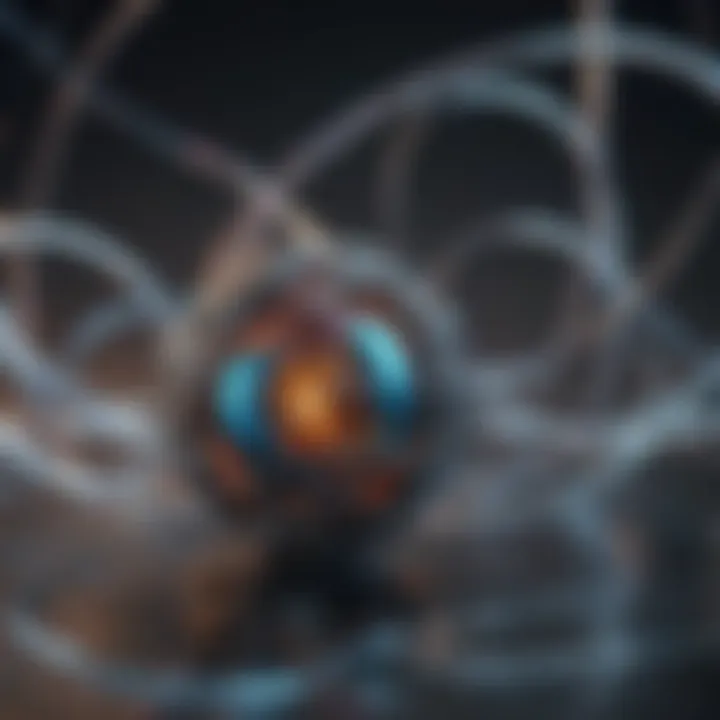
Intro
The field of quantum computing is evolving rapidly and Google has emerged as a significant player in this domain. This technology is not simplistic, as it operates on principles that are markedly different from those governing classical computing. By exploiting the principles of quantum mechanics, Google aims to push the boundaries of what can be achieved computationally. This article provides an in-depth analysis of Googleβs journey in quantum computing, examining the breakthroughs it has made and the challenges it continues to face.
This discussion is vital for a range of audiences including students, researchers, educators, and professionals. Given the potential implications of quantum technology across various industries, understanding its nuances is imperative. We will dissect core concepts, provide insights into Google's innovative contributions, and explore the broader implications for society.
Key Research Findings
Overview of Recent Discoveries
Google has pioneered several advancements in quantum computing that have captured global attention. One major milestone was the achievement of quantum supremacy in 2019, when Google announced that its quantum processor, Sycamore, completed a specific task faster than the worldβs fastest supercomputer could. This achievement underscores the possibilities of quantum processing power in handling tasks that are infeasible for classical computers.
In addition to quantum supremacy, Google has worked extensively on developing robust quantum algorithms. These algorithms are crucial for leveraging the computational advantages of quantum mechanics, demonstrating quantum speedup in areas such as optimization and machine learning.
Significance of Findings in the Field
The findings from Googleβs research signify a watershed moment for the field of quantum computing. They not only validate the theoretical foundations of quantum mechanics in computation but also open new avenues for research. Googleβs advancements may ultimately lead to breakthroughs in cryptography, materials science, and complex systems modeling, reshaping industries that rely heavily on data processing.
"Quantum computing is poised to revolutionize how we think about data analysis and problem-solving, moving beyond the limitations of traditional architectures."
This statement reflects the broader sentiment in the scientific community regarding the transformative potential of quantum technology.
Breakdown of Complex Concepts
Simplification of Advanced Theories
At the core of quantum computing are concepts such as qubits, entanglement, and superposition. A qubit is the basic unit of quantum information. Unlike classical bits, which must be either 0 or 1, qubits can exist in multiple states simultaneously due to superposition. This property allows quantum computers to process a vast amount of possibilities concurrently.
Entanglement, another critical concept, allows qubits that are entangled to be correlated with each other, regardless of the distance separating them. This feature enables faster computation and communication, forming a cornerstone of quantum algorithms that could revolutionize their respective fields.
Visual Aids and Infographics
To further clarify these complex ideas, visual aids can be instrumental. For instance, diagrams illustrating the behavior of qubits under various conditions, or infographics that compare classical and quantum computing paradigms, can serve as essential educational resources. These tools can bridge the gap between theoretical constructs and practical applications, enhancing the learning experience for the audience.
Prelude to Quantum Computing
Quantum computing represents a paradigm shift in the way we process information. Unlike classical computers that rely on bits as the fundamental unit of data, quantum computers utilize quantum bits, or qubits, which can exist simultaneously in multiple states. This unique property allows quantum systems to perform complex calculations far more efficient than classical systems.
The significance of understanding quantum computing lies in its potential applications across various sectors, from cryptography to drug discovery and optimization problems. Recognizing the foundations of quantum computing facilitates insights into how it will affect current technologies and industry practices. Furthermore, it is essential for students, researchers, and professionals to comprehend the evolving landscape of this technology to stay relevant in their respective fields.
Definition of Quantum Computing
Quantum computing is the study of how to leverage the principles of quantum mechanics to perform computation. At its core, it relies on the phenomena of superposition and entanglement.
Superposition allows a qubit to exist in multiple states at once. This capability enables quantum systems to explore numerous solutions simultaneously. On the other hand, entanglement is a unique correlation between qubits, regardless of the distance separating them. This aspect allows for a more efficient interconnection between quantum bits, leading to enhanced computational capabilities.
Historical Context
The journey of quantum computing can be traced back to the early 1980s when physicist Richard Feynman first proposed that quantum mechanics could be used to model quantum systems. The development of quantum algorithms, such as Shor's algorithm in the 1990s, showcased the potential for significant speedups in computational tasks, particularly for factoring large numbers, a problem that underlies current encryption methods.
Subsequent advancements in both theoretical foundations and experimental implementations laid the groundwork for todayβs quantum computing initiatives. Googleβs involvement in this field started earnestly when they introduced their Quantum AI lab in 2013, aiming to explore how quantum computing could enhance artificial intelligence and machine learning tasks. This historical perspective provides critical insight into the technological evolution and ongoing efforts in quantum computing, hinting at the vast possibilities ahead.
Fundamentals of Quantum Mechanics
Understanding quantum mechanics is essential to grasp how quantum computing operates. This section explores fundamental concepts and their relevance to the advancements in computing that Google and other organizations are pursuing. The principles of quantum mechanics provide the foundation for quantum bits, superposition, and entanglementβconcepts that are pivotal in enhancing computational capabilities.
Quantum Bits and Superposition
Quantum bits, or qubits, are the building blocks of quantum computing. Unlike classical bits, which represent either a 0 or a 1, qubits can represent multiple states simultaneously due to a property called superposition. This allows quantum systems to process information in a fundamentally different way.
The significance of superposition lies in its capacity to vastly increase the computational power of quantum devices. When a qubit is in superposition, it can be thought of as being in both the 0 and the 1 state at the same time, until measured. This characteristic enables quantum computers to explore a much larger solution space compared to traditional computers.
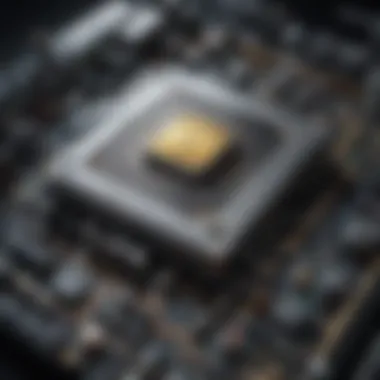
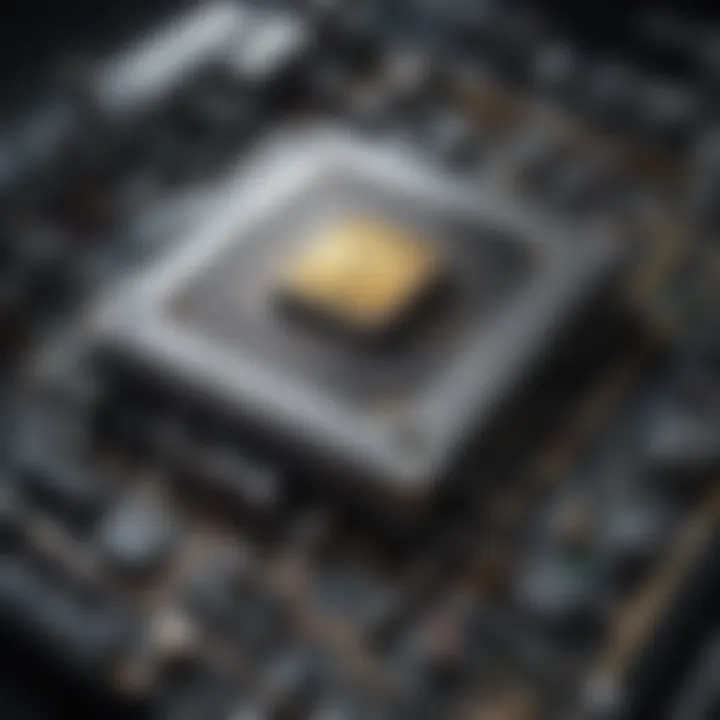
Qubits and superposition can provide an exponential increase in computational efficiency.
For instance, while a classical computer with 10 bits can be in one of 1024 possible states, a quantum computer with 10 qubits can be in all 1024 states simultaneously. This potential makes quantum computers uniquely capable of solving complex problems, which were previously infeasible for classical systems. The manipulation of qubits through quantum gates allows for intricate computations, fundamentally changing how data is processed.
Entanglement and Quantum States
Entanglement is another core principle of quantum mechanics that underpins the power of quantum computing. When qubits become entangled, the state of one qubit becomes dependent on the state of another, no matter the distance between them. This phenomenon does not have a classical counterpart and adds a layer of complexity and capability to quantum systems.
The implications of entanglement are profound. It opens avenues for parallel processing and instantaneous communication between qubits. For example, if two entangled qubits are manipulated, a change to one qubit instantaneously affects the other, which can enhance error correction and data integrity.
Entangled states make it possible for quantum computers to perform operations that would be impossible for classical individuals. They create correlations between qubits that can be leveraged to improve computational efficiency. However, entanglement is fragile and maintaining it poses significant technical challenges, particularly in larger systems.
Overall, the categories of superposition and entanglement provide insights into how quantum computers operate at a fundamental level. These principles will be vital as we explore specific algorithms and their practical applications in the world of computing.
Google's Role in Quantum Computing
Google's involvement in quantum computing reflects not only the company's commitment to advancing technology but also its understanding of the essential shifts within the computing paradigm. As a leader in the tech industry, Google has allocated significant resources towards the exploration of quantum computing, recognizing its potential to solve problems beyond the capabilities of classical computers. By focusing on this branch of computer science, Google aims to unlock new possibilities across various fields. This section will emphasize the core aspects of Google's role in quantum computing and discuss the implications of its efforts on both the tech industry and society as a whole.
Overview of Google Quantum AI
Google Quantum AI is a division dedicated to the development of quantum computing technologies. Key to its strategy is the pursuit of building a quantum computer that can outperform traditional computers in specific tasks known as quantum supremacy. Google initiated its quantum research efforts in 2013, which represents a significant investment in human capital and infrastructure.
The architecture of Google's quantum systems is based on superconducting qubits, which are crucial for conducting quantum operations. These qubits can exist in a state of superposition, allowing for the parallel processing of vast amounts of information. By leveraging advanced algorithms, Google aims to enhance the performance and efficiency of quantum computing.
Additionally, the collaboration with academic institutions and other organizations promotes knowledge sharing and innovation. This creates a robust ecosystem for research and development, which is essential for advancing the field.
Key Milestones Achieved
Over the years, Google has made remarkable strides in quantum computing. Here are some key milestones:
- Quantum Supremacy: In October 2019, Google announced that it achieved quantum supremacy using its 53-qubit quantum processor, Sycamore. This milestone involved solving a problem in 200 seconds, which would take the fastest supercomputers approximately 10,000 years to complete.
- Control of Qubits: Google demonstrated effective control over multiple qubits, indicating the potential for more complicated quantum algorithms. Successfully manipulating qubits is essential for reliable computations.
- Development of Quantum Algorithms: Google has developed quantum algorithms aimed at addressing real-world issues, such as optimization problems and materials science. This work demonstrates the practical applications of quantum computing.
In summary, Google's robust presence in the quantum computing landscape can be seen through its foundational developments and significant advancements. The ongoing efforts not only enhance technology but also pave the way for the future possibilities that quantum computing holds.
"The future of quantum computing is not only about building faster machines but understanding how they can redefine the boundaries of computation."
Quantum Hardware: The Technological Framework
Quantum hardware is essential in the field of quantum computing. It serves as the backbone that enables the revolutionizing algorithms and applications that quantum computing promises to deliver. This section sheds light on the intricate components that form the framework for quantum processors, their design principles, and how they function effectively in harnessing quantum mechanics.
Quantum Processors: Design and Functionality
The heart of quantum computing lies in quantum processors. These processors are composed of quantum bits, or qubits, which can exist in multiple states simultaneously. Unlike classical bits, which can be either 0 or 1, qubits can be in a state of superposition, allowing them to perform a vast number of calculations at once.
Google has invested significant resources into developing its quantum processors. One of the most notable designs is based on superconducting qubits. These qubits are cooled to near absolute zero to reduce noise and interference, enabling stable and reliable computational processes. The design also incorporates microwave technology to manipulate the states of the qubits, allowing precise control over quantum operations.
Quantum processors are evaluated based on several key aspects:
- Qubit Coherence Time: This is the time a qubit can maintain its quantum state before it decoheres, or loses its quantum information.
- Gate Fidelity: This refers to the accuracy of quantum operations performed on the qubits. High fidelity is essential to perform complex algorithms reliably.
- Scalability: The ability to increase the number of qubits without losing performance is crucial for building more powerful quantum systems.
"Scalable quantum processors are necessary to realize the true potential of quantum computing and solve problems that are currently unsolvable by classical means."
Error Correction in Quantum Systems
Error correction is a vital challenge in quantum computing. Due to the fragile nature of qubits, quantum states are prone to errors caused by decoherence and other noise sources. Quantum error correction schemes have been developed to detect and correct these errors without directly measuring the qubit states, which would collapse the superposition.
The threshold theorem suggests that as long as the error rate of each gate is below a certain level, arbitrary long calculations can be performed with high reliability. Some approaches include:
- Stabilizer Codes: These are based on linear algebra and allow for error detection without revealing information about the stored data.
- Surface Codes: These have gained interest due to their relatively high fault tolerance and scalability. They arrange qubits on a two-dimensional grid to facilitate error correction.
- Cat Codes: Utilizing cat states, which are entangled superpositions, to protect information against phase errors.
In summary, quantum hardware serves as the foundation of Google's quantum computing ambitions. Understanding the design and functionality of quantum processors, along with the strategies for error correction, provides insight into how quantum computing can be realized and scaled to address complex real-world problems.
Quantum Algorithms and Their Significance
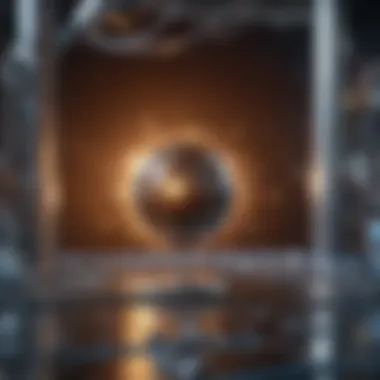
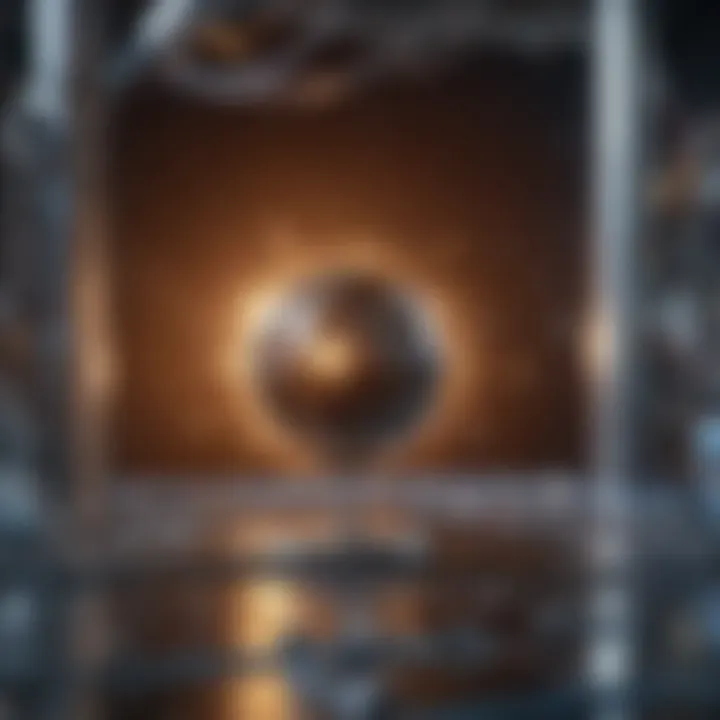
Quantum algorithms represent a pivotal component of quantum computing, distinguishing it sharply from classical computing methods. Their significance lies in the ability to solve certain problems exponentially faster. This is primarily due to the inherent parallelism afforded by quantum mechanics. Quantum algorithms harness phenomena like superposition and entanglement to offer solutions that would take classical algorithms a prohibitive amount of time. Understanding how these algorithms work is crucial for anyone involved in the fields of computing, cryptography, and data analysis.
Shor's Algorithm and Factorization
Shor's Algorithm, developed by mathematician Peter Shor in 1994, illustrates the profound potential of quantum computation. This algorithm specifically targets the problem of integer factorization, which is foundational to much of modern cryptography, particularly RSA encryption. Classical algorithms struggle with factorization as the size of the integers increases. However, Shor's Algorithm operates in polynomial time, allowing it to factor large numbers efficiently.
The implications of Shor's work are substantial. If a sufficiently powerful quantum computer can be built, it could compromise current encryption systems relatively easily. Users, businesses, and governments will need to evaluate this risk. Research continues into post-quantum cryptography to safeguard against such vulnerabilities.
In summary, Shor's Algorithm poses both opportunities and challenges, demanding readiness in various sectors as quantum technology evolves.
Grover's Algorithm and Search Optimization
Grover's Algorithm represents another significant quantum algorithm, focusing on the problem of search optimization. Classically, searching through an unsorted database requires linear time, as each entry must be checked sequentially. Grover's Algorithm, however, achieves this search in roughly the square root of the total number of items.
This provides a dramatic improvement in efficiency, particularly for large databases. The implications range from faster data retrieval in vast data sets to enhanced capabilities in fields such as artificial intelligence and machine learning. Just as with Shor's Algorithm, Grover's creation prompts considerations for security and computational limits in various applications.
Practical Applications of Quantum Computing
Quantum computing represents a pivotal advancement in technology. As industries progressively seek solutions that deal with complex problems, understanding the practical applications of quantum computing becomes crucial. This section outlines key areas where quantum computing can significantly impact various domains, particularly focusing on cryptography, artificial intelligence, and drug discovery. Each application reveals the specific benefits and implications that quantum computing brings to the forefront of technology and society.
Quantum Computing in Cryptography
Cryptography serves as the backbone for securing digital communication. Traditional encryption methods rely heavily on computational difficulty, particularly with large prime factorization. Quantum computers have the potential to disrupt this landscape. Shor's algorithm, a quantum computing algorithm, enables rapid factorization, threatening widely adopted methods such as RSA encryption.
The implications are substantial. Organizations must rethink their security protocols as quantum capabilities become more accessible. Post-quantum cryptography is an emerging area of research, aiming to develop encryption systems that are secure against quantum attacks. This effort highlights an urgent need to innovate within cryptography, ensuring that sensitive data remains protected in a quantum world.
Impact on Artificial Intelligence
Artificial intelligence (AI) leverages vast amounts of data to learn and make predictions. Quantum computing can further enhance AI capabilities, allowing algorithms to analyze complex datasets much faster than classical computers. Quantum algorithms can improve optimization tasks which are fundamental in machine learning, making it feasible to tackle problems that were previously intractable.
For instance, quantum-enhanced machine learning can lead to better pattern recognition. This capability can transform industries such as finance, healthcare, and marketing. With quicker access to insights, businesses can make more informed decisions, enhancing efficiency and productivity.
Applications in Drug Discovery
The pharmaceutical industry faces immense challenges in drug discovery, with lengthy timelines and significant costs. Quantum computing presents a potential solution by simulating molecular interactions at unprecedented scales and speeds. This ability to model complex quantum systems allows researchers to explore vast chemical spaces, sifting through numerous compounds to identify promising drug candidates.
Recent studies suggest that quantum algorithms can predict molecular properties and behaviors far more accurately than classical methods. This accuracy can shorten development times and reduce wasted resources in the search for effective medications. As quantum advancements continue, the potential for breakthroughs in drug discovery becomes increasingly tangible.
"Quantum computing is not just about speed; it's about capability. It's a new way of approaching complex problems in ways that traditional computers cannot."
Challenges Facing Quantum Computing
The journey to realizing the full potential of quantum computing is fraught with significant challenges. Understanding these obstacles is crucial for students, researchers, educators, and professionals who are invested in this burgeoning field. Quantum computing presents profound capabilities that stand to reshape technology and industry. However, getting there requires addressing various technical and financial challenges to ensure sustainable progress.
Technical Limitations and Scalability
One major hurdle in the development of quantum computing is technical limitations. Quantum bits, or qubits, are the foundation of quantum computation. Unlike classical bits, qubits can exist in multiple states simultaneously, thanks to superposition. However, maintaining the delicate state of qubits is not easy due to decoherence. This phenomenon occurs when qubits interact with their environment, causing loss of information.
Currently, there are different technologies used to create qubits, such as superconducting circuits and trapped ions. Each method has its benefits but is also associated with specific technical limitations. For instance, superconducting qubits, while among the leading contenders, face challenges related to scaling up due to their susceptibility to thermal noise and electromagnetic interference. This makes creating large-scale quantum computers more difficult.
In addition to decoherence, there is the issue of scalability. Quantum systems need to enable error correction techniques to ensure reliable computing. As the number of qubits increases, the complexity of managing error rates rises exponentially. This affects the system's overall scalability and functionality, as the architecture must adapt to handle increased computational loads without additional errors intervening. The race for highly functional quantum systems continues, but identifying a path that balances robustness, scalability, and fidelity is challenging.
Funding and Research Barriers
Another significant factor in advancing quantum computing lies in funding and research barriers. Quantum research requires substantial investmentβfrom both government entities and private sectors. Many projects are often underfunded, which results in limitations on the scope and scale of research. As a result, many innovative ideas remain unexplored due to insufficient resources.
Moreover, there are specific research constraints tied to the interdisciplinary nature of quantum computing. Combining efforts from fields such as physics, engineering, and computer science can be complex. Researchers often require specialized equipment or expertise, complicating collaboration across different domains. Larger institutions may provide the necessary infrastructure, but not every research entity has access to such resources.
A related challenge is the shifting nature of funding priorities. Quantum computing is a rapidly evolving field. As new discoveries emerge, funding priorities can shift, potentially diverting attention from foundational research to more applied projects. This leads to a sporadic pattern of funding that can disrupt ongoing studies and innovations.
"The path to quantum supremacy is riddled with both technical and funding challenges, making it an intricate puzzle that demands sustained effort and collaboration."
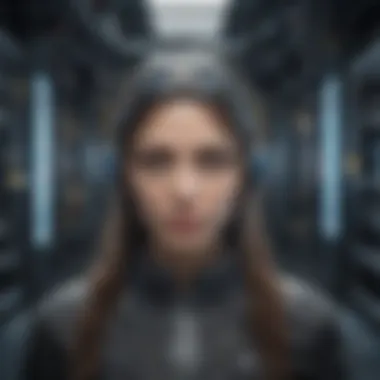
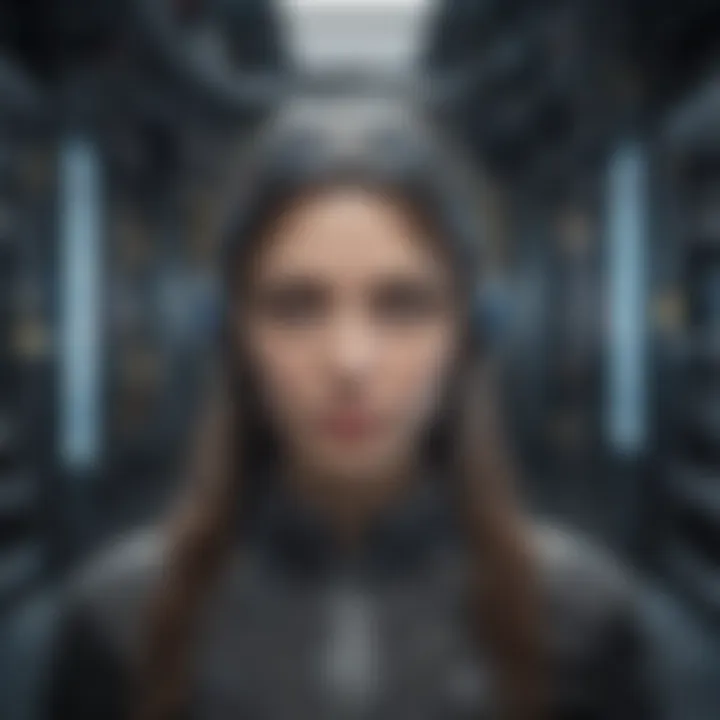
In summary, technical limitations around qubit performance and scalability, alongside funding and research barriers, represent critical challenges in quantum computing. Addressing these obstacles is fundamental to unlocking the full potential of this transformative technology.
Ethical Considerations in Quantum Computing
The rapid evolution of quantum computing brings to focus a series of ethical considerations that warrant significant attention. As Google advances its quantum technologies, the implications of these innovations become increasingly pronounced. These ethical issues are crucial to the discourse surrounding quantum computing for several reasons, particularly in terms of privacy, society, and the responsibilities of developers and researchers. Understanding these considerations is vital for ensuring that advancements serve the greater good while mitigating potential harm.
Privacy and Data Security Issues
The cornerstone of quantum computing's potential lies in its ability to process vast amounts of data at unprecedented speeds. However, this processing power could lead to serious privacy and data security challenges. The capability of quantum algorithms to break current encryption methods poses a considerable threat to personal and sensitive information.
Several key issues emerge regarding privacy:
- Vulnerability to Attacks: Current encryption methods such as RSA are susceptible to algorithms like Shorβs. If quantum computers become efficient enough, they could easily decrypt information that is currently secure.
- Data Collection: The heightened ability of quantum systems to analyze data raises questions about the extent of data collection. This could lead to potential misuse of information without adequate consent.
- Surveillance: Enhanced computational abilities can increase government and corporate surveillance, infringing on individual privacy rights.
The urgency to develop quantum-safe encryption is clear. Researchers need to focus on creating encryption methods resistant to quantum attacks. The push for ethical guidelines is crucial to strike a balance between innovation and personal privacy.
Implications for Society
The societal implications of quantum computing extend far beyond technology itself. These implications necessitate an ethical framework that guides the deployment of quantum systems in various domains.
- Inequality: There is a risk that quantum computing could exacerbate existing societal inequalities. Access to technology may become limited to those who can afford it, potentially widening the technology gap across different socio-economic groups.
- Job Displacement: As quantum computing automates processes in various industries, the potential for job displacement exists. Workers in sectors affected by automation may face significant economic challenges unless they are retrained for new roles.
- Ethical Responsibilities of Developers: Researchers and engineers at companies like Google hold significant responsibility for the ethical implications of their work. It is essential that they consider the potential consequences of their developments, ensuring they promote positive societal outcomes.
The convergence of these ethical issues illuminates the need for thoughtful and inclusive dialogue among researchers, policy makers, and the public. It is imperative that the evolution of quantum computing incorporates ethical considerations, ensuring that its deployment is aligned with the larger goals of justice, equity, and security.
"The future of quantum technology is not only about technological advancement, but also about fostering an ethical landscape that prioritizes the well-being of society."
This commitment to ethical considerations will ultimately guide the integration of quantum computing into society, influencing everything from regulatory policies to corporate practices.
The Future of Quantum Computing
The future of quantum computing stands as a significant focal point for both researchers and industries. The rapid advancements in this field hold the promise of redefining computational capacities. As applications for quantum technology become increasingly diverse, it is crucial to anticipate its trajectory. What follows explores emerging trends alongside long-term prospects that could shape the technological landscape.
Emerging Trends in Quantum Research
There is a visible shift in focus within quantum research, with several pivotal trends emerging.
- Hybrid Quantum-Classical Systems: Researchers are looking at combining classical computing with quantum processing. This approach aims to enhance problem-solving abilities without entirely relying on quantum systems. Hybrid systems can leverage the strengths of both paradigms.
- Increased Collaboration: Institutions worldwide are fostering partnerships. Academic research labs are joining forces with tech giants like Google to explore practical applications. Collaborative projects may accelerate breakthroughs in quantum algorithms and error correction.
- Focus on Quantum Software: As hardware matures, the necessity to develop robust quantum software becomes evident. Specialized programming languages such as Qiskit are on the rise, allowing researchers to design algorithms specifically for quantum machines, thus expanding their usability.
- Quantum Supremacy: Discussions surrounding Googleβs accomplishment of quantum supremacy mark a critical trend. This concept refers to quantum computers performing tasks beyond the capability of classical machines, which raises exciting possibilities for future computational efficiency.
"Quantum computing is a transformative technology that will unlock new capabilities in information processing and data analysis."
Long-term Prospects for Quantum Technology
As quantum technology matures, its long-term implications extend across various sectors. The potential applications suggest vast transformations.
- Revolutionizing Industries: Quantum computing is poised to influence fields such as finance, pharmaceuticals, and logistics. In finance, for instance, quantum algorithms may optimize trading strategies and risk analysis, providing competitive advantages.
- Advancements in Artificial Intelligence: The integration of quantum computing with AI could enhance machine learning tasks. This synergy can facilitate data processing speeds previously deemed impossible, potentially transforming how machines learn and adapt.
- Enhanced Security Protocols: With the rise of quantum computing, conventional encryption methods face challenges. On the other hand, quantum key distribution may emerge as a robust alternative, ensuring secure communication channels that resist traditional hacking techniques.
- Research Efficiencies in Science: Quantum computing could accelerate simulations and problem-solving in scientific inquiries. This could lead to breakthroughs in material science, climate modeling, and complex system analysis.
The roadmap ahead for quantum technology appears bright but requires careful navigation. The interplay between innovation and ethical considerations will shape its integration into society. Through a clear understanding of these emerging trends and prospects, stakeholders can strategically position themselves in this evolving landscape.
Finale
The conclusion in this article serves a vital role in synthesizing the intricate discussions on quantum computing as developed by Google. It emphasizes how the journey from theoretical concepts to practical applications is not merely significant but transformative. As we summarize the findings, it's essential to highlight both the promises and the challenges presented by quantum computing.
Understanding quantum computing opens up new avenues for solving problems that classical computing cannot efficiently address. The potential benefits extend across various sectors, including healthcare, cryptography, and artificial intelligence. The unique capabilities offered by quantum algorithms, such as Shor's and Grover's, signify a paradigm shift in computation and data processing.
However, alongside these opportunities lie substantial challenges. The technical limitations related to noise, fault tolerance, and scalability must not be overlooked. Moreover, funding and research barriers significantly impact the pacing of innovation in this field.
"The future of quantum computing is not only about expanding computational power but also about navigating the accompanying ethical dilemmas and societal implications."
As we look to the future, it is paramount to consider the ethical implications of quantum computing on privacy and data security. The society must engage in open dialogues to address these concerns while embracing the technology's potential. The promise of quantum computing is indeed substantial, but it requires a concerted effort to manage the challenges it presents. In concluding, this article underscores the importance of ongoing research, ethical consideration, and sustained collaboration to unlock the full potential of quantum technologies.
Recap of Quantum Computing's Promise and Challenges
The promise of quantum computing resides in its ability to revolutionize various fields through unprecedented computational power. This technology holds the potential to break traditional barriers, enabling solutions to complex problems in fraction of the time that classical computers take. In cryptography, for instance, quantum computers could render many current encryption methods obsolete. The implications for data privacy and security are profound.
However, significant obstacles remain. Here are some key points to remember:
- Technical Limitations: Current quantum systems struggle with errors and stability. Enhancements in error correction techniques are essential to make quantum computing practical.
- Scalability: As quantum processors increase in complexity, maintaining coherence and managing qubit interactions becomes more challenging.
- Funding and Research Gaps: Investment in quantum technologies is crucial for progress. Without sufficient financial backing, research in this promising field may slow down.
- Ethical Considerations: As this technology continues to evolve, its impact on society raises important questions about privacy, data rights, and security.
In essence, while quantum computing is poised to transform industries, the interconnected challenges cannot be ignored. The path forward requires a balanced approach, merging innovative capability with ethical responsibility.