Understanding Glial Cells: The Unseen Architects of the Brain
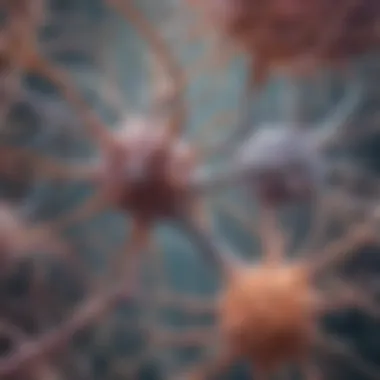
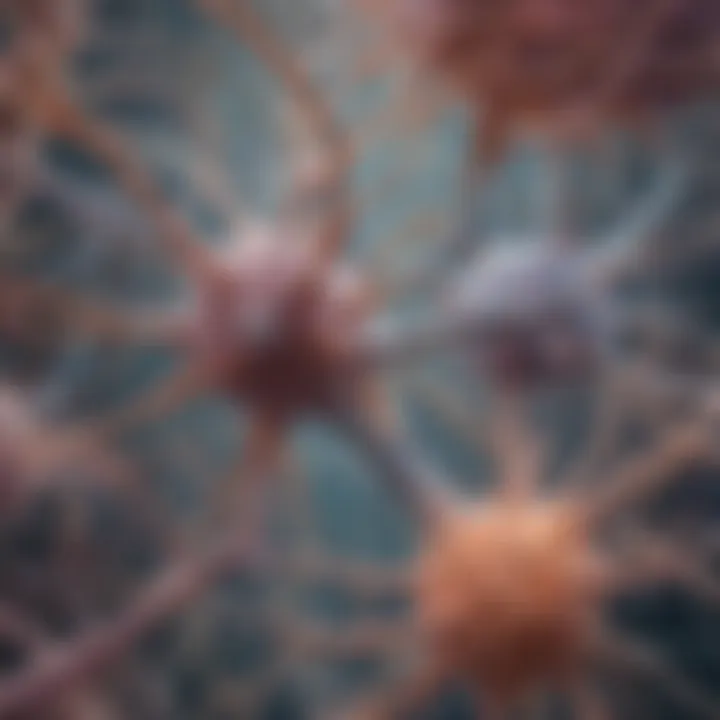
Intro
Glial cells represent a vital yet often overlooked component of the central nervous system. While neurons are celebrated for their role in processing and transmitting information, glial cells perform equally critical functions. These cells are not mere support structures; they are active participants in maintaining homeostasis, providing support, and protecting neurons. Understanding glial cells is essential for grasping the full picture of brain functionality. Their roles extend beyond what was traditionally understood, influencing various neurobiological processes.
In recent years, interest in glial cells has surged. Research indicates their involvement in numerous neurological conditions, including Alzheimerβs, multiple sclerosis, and even depression. As we deepen our understanding of these cells, new avenues for treatment and research emerge. The complexity of glial cell types allows them to fulfill specialized functions in the brain.
This article aims to provide a comprehensive exploration of glial cells, starting with recent research findings that highlight their importance, including their structure, communication mechanisms, and implications in health and disease. By enhancing our awareness of these unseen architects, we aim to clarify their contributions to neuroscience and neurobiology.
Prolusion to Glial Cells
Glial cells, often overshadowed by the more recognized neurons, are fundamental to the health and functionality of the nervous system. Their roles extend well beyond mere support; they actively shape the brain's architecture and influence biochemical processes crucial for neural health. Understanding glial cells is essential for comprehending the complete picture of brain biology, particularly concerning neurodevelopment and repair mechanisms.
Defining Glial Cells
Glial cells, generally referred to as glia, encompass a diverse group of non-neuronal cells found in the central and peripheral nervous systems. Unlike neurons, which transmit signals, glial cells perform various supporting functions. They are classified into several types: astrocytes, oligodendrocytes, microglia, and ependymal cells. Each type plays a unique role in maintaining brain homeostasis and facilitating neural activity.
Astrocytes, for example, are notorious for their star-like shape and are involved in nutrient support, ion balance, and the integrity of the blood-brain barrier. Oligodendrocytes are key players in myelination, which insulates neuronal axons to speed up signal transmission. Microglia act as resident immune cells, surveying for pathogens and clearing debris from the brain. Ependymal cells are responsible for producing cerebrospinal fluid, crucial for protecting the brain.
The multitude of functions carried out by these cells signifies their importance for both normal brain operation and the response to injury or disease.
Historical Context of Glial Cell Research
The study of glial cells has evolved significantly over the years. Historically, glial cells were considered merely supportive structures with little functional importance. Early neuroanatomists often dismissed them, focusing primarily on neurons, which were thought to be the primary players in neural communication.
However, advancements in microscopy and staining techniques during the late 19th and early 20th centuries began uncovering the complexities of glial cell functions. Researchers like Santiago RamΓ³n y Cajal and Rudolf Virchow contributed to the understanding of neuroanatomy, but it was not until the second half of the 20th century that a shift occurred.
Modern methodologies, such as molecular biology and genetic engineering, have led to a resurgence of interest in glial cells. Studies have now revealed that they are crucial in areas like synaptic modulation and response to injury. The recognition of glial cells as active participants in the brain's ecosystem continues to grow, making them an exciting focus of contemporary neuroscience research.
"Glial cells are more than just packing material for neurons, they are dynamic participants in brain function and health."
This evolving understanding calls for further exploration of glial cells, particularly their implications in various neurological disorders. As glial cell research expands, we gain a deeper insight into their vital roles in the intricate workings of our brains.
Classification of Glial Cells
The classification of glial cells is crucial for understanding their diverse roles within the nervous system. Each type brings unique functions that complement the activities of neurons, establishing a symbiotic relationship necessary for maintaining brain health and functionality. By categorizing these cells, researchers can explore their specific contributions and implications in both normal physiology and pathological conditions. Therefore, an examination of glial cell classification allows for deeper insights into how these cells operate and influence neural processes.
Astrocytes
Astrocytes are star-shaped glial cells that perform multiple roles, making them one of the most abundant cell types in the brain.
Functions in neurotransmission
One significant aspect of astrocytes is their role in neurotransmission. These cells help in the recycling of neurotransmitters, particularly glutamate, which is critical for neuronal communication. Astrocytes take up excess neurotransmitter, preventing excitotoxicity and ensuring proper synaptic function. This recycling process is beneficial because it supports sustained neuronal activity without overwhelming the synaptic cleft. A unique feature of astrocytic involvement in neurotransmission is their ability to release gliotransmitters, which modulate synaptic strength and plasticity. This adds a layer of complexity to how information is processed in the brain.
Role in blood-brain barrier maintenance
Another vital function of astrocytes is their contribution to the blood-brain barrier. This barrier protects the brain from potentially harmful substances in the bloodstream while allowing essential nutrients to pass through. Astrocytes form the end-feet around blood vessels, supporting the integrity of endothelial cells. They release signaling molecules that contribute to the tight junctions of the blood-brain barrier. This role is acclaimed for maintaining homeostasis, which is critical for proper neuronal function. Any dysfunction in astrocytes can compromise the barrier, leading to neurological diseases.
Oligodendrocytes
Oligodendrocytes are specialized glial cells primarily responsible for producing myelin in the central nervous system.
Myelination of axons
The process of myelination is essential for the rapid transmission of electrical signals along axons. Oligodendrocytes extend their membrane to wrap around multiple axons, forming a protective sheath. This myelination not only increases the speed of nerve impulse conduction but also enhances the efficiency of signal transmission. The efficiency of myelination is beneficial for the overall function of the nervous system. A distinctive feature of oligodendrocytes is their ability to form and repair myelin sheaths, which has implications for recovery following injury or disease.
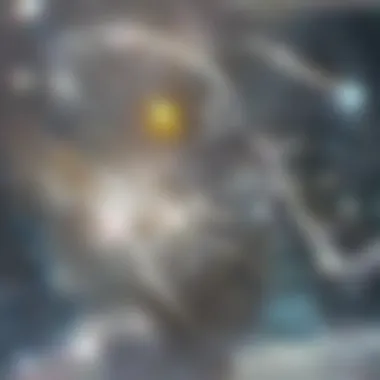
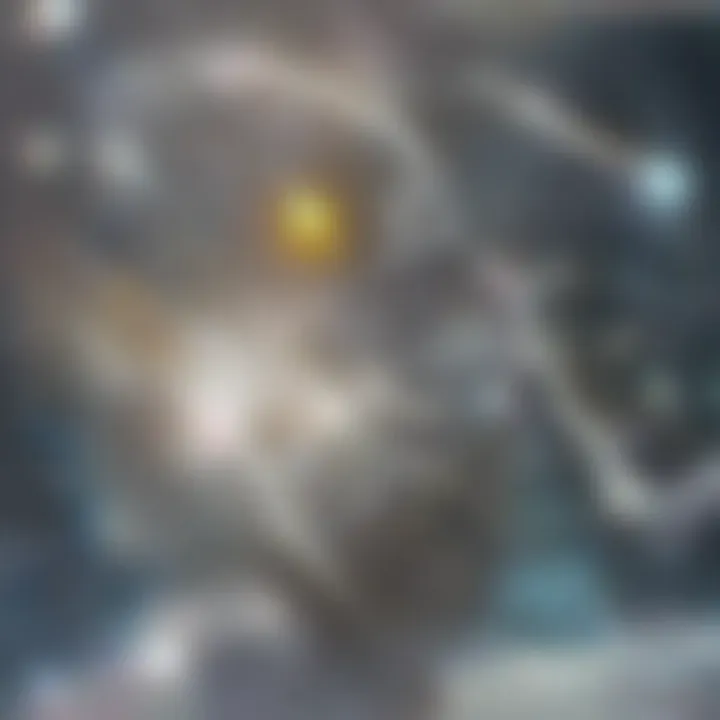
Influence on neural signal propagation
Oligodendrocytes also influence the propagation of neural signals. The myelin sheath acts as an insulator, allowing action potentials to jump between gaps known as nodes of Ranvier. This process, called saltatory conduction, is more energy efficient than continuous conduction along unmyelinated axons. This unique characteristic ensures faster communication between neurons, enhancing overall brain responsiveness. Efficient neural signal propagation is critical, affecting everything from reflexes to complex cognitive tasks.
Microglia
Microglia are the immune cells of the central nervous system, offering protection through surveillance and response.
Immune surveillance in the central nervous system
Microglia continuously monitor the environment in the central nervous system. They detect pathogens and respond to injury. This immune surveillance is beneficial, as it allows for a swift response to potential threats. A unique aspect of microglial function is their ability to change shape and mobilize quickly to sites of injury or infection, demonstrating their adaptiveness.
Response to injury and disease
When faced with brain injury or disease, microglia exhibit a distinct response. They can undergo activation, leading to phagocytosis of debris and cellular waste. However, chronic activation can have detrimental effects, potentially contributing to inflammation and neuronal damage. This duality of microglial functionβbeing protective yet potentially harmful when overactiveβestablishes their complex role in brain health. Understanding these dynamics is key in research concerning neurodegenerative diseases.
Ependymal Cells
Ependymal cells are distinct in their ciliated appearance and location within the ventricular system of the brain.
Cerebrospinal fluid production
One of their main functions is the production and circulation of cerebrospinal fluid (CSF). Ependymal cells line the ventricles and play a pivotal role in the homeostasis of the CSF. This production is essential for cushioning the brain and spinal cord, as well as removing waste. Their unique cilia promote the flow of CSF, ensuring it reaches all parts of the central nervous system.
Role in neurogenesis
Ependymal cells are also implicated in neurogenesis, particularly in specific brain regions like the hippocampus. They can act as stem cells, giving rise to new neurons and glial cells. This aspect of their function makes them a subject of increasing interest, especially in the context of brain repair. The ability of ependymal cells to contribute to neurogenesis offers potential therapeutic avenues in neurodegenerative conditions.
Functions of Glial Cells
Glial cells, often overshadowed by their neuronal counterparts, perform essential roles in maintaining brain health and function. While neurons are responsible for transmitting signals, glial cells support, protect, and nourish these signaling units. The functions of glial cells can be grouped into various categories, each underscoring their significance in the nervous system. Understanding these functions not only provides insight into the basic biology of the brain but also into potential therapeutic avenues for neurological disorders.
Support and Nourishment
Energy metabolism
Energy metabolism in glial cells supplies the essential ATP needed for neuronal activity. Glial cells, particularly astrocytes, play a critical role in the uptake of glucose and its conversion into lactate, which neurons utilize as fuel. The unique feature of this process is that while neurons depend on rapid energy sources during intense activity, astrocytes can efficiently store energy substrates for later use. This capacity not only supports synaptic transmission but also mitigates energy deficits during periods of increased neuronal demand. Thus, this mechanism of energy metabolism is crucial for optimal brain function, highlighting how glial cells act as a reservoir for neuron energy needs.
Regulation of ion balance
The regulation of ion balance is another vital function of glial cells. Astrocytes help maintain the ionic environment of the brain by monitoring and buffering potassium ions that are released during neuronal firing. This characteristic is beneficial since excessive accumulation of potassium can lead to neuronal excitability and even neurotoxicity. By rapidly absorbing and redistributing these ions, astrocytes ensure a stable extracellular space that is essential for normal electrical activity in neurons. This balancing act is indeed crucial for preventing imbalances that could disrupt signaling pathways in the brain.
Facilitating Neural Communication
Role of gliotransmitters
Gliotransmitters are signaling molecules released by glial cells that impact neuronal communication. These substances, such as glutamate and ATP, can modulate synaptic events, influencing how neurons communicate. The advantage of gliotransmitter action is their ability to finely tune synaptic strength and plasticity, offering a level of control that complements traditional neurotransmission. By affecting receptor activity and influencing signaling cascades, gliotransmitters play a role in learning and memory, reinforcing the importance of glial cells in cognitive functions.
Influence on synaptic plasticity
The influence on synaptic plasticity is a key aspect of how glial cells contribute to brain function. Glial cells modulate the structural and functional changes that occur at synapses in response to activity. They do this through pathways involving calcium signaling and the release of gliotransmitters. A major characteristic of this role is its potential for long-term potentiation and depression, processes that are foundational to memory formation and learning. While this mechanism shows great promise in enhancing our understanding of synaptic changes, it also raises concerns regarding the dysregulation found in various neurological disorders.
Maintenance of Homeostasis
Cerebrovascular regulation
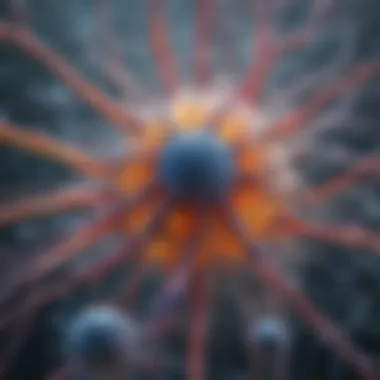
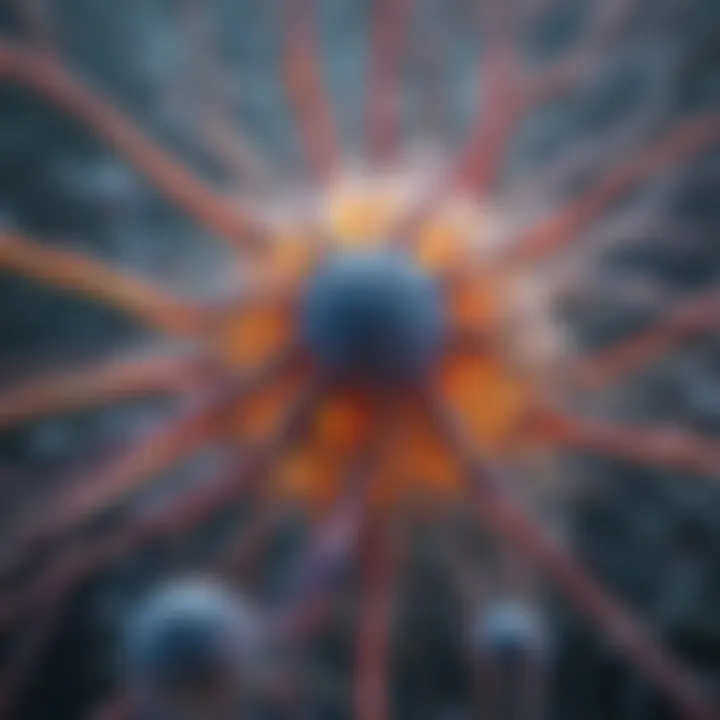
Glial cells are integral to the regulation of cerebrovascular function, influencing blood flow in relation to neuronal activity. Astrocytes, in particular, express receptors that respond to neurotransmitters and can signal vasodilation or vasoconstriction of blood vessels. This characteristic ensures a sufficient supply of oxygen and nutrients that are essential during periods of heightened brain activity. By bridging neuronal signaling with vascular responses, glial cells maintain a dynamic equilibrium necessary for sustaining brain metabolism.
Response to physiological changes
The response to physiological changes is crucial for maintaining homeostasis in the brain. Glial cells actively adjust to fluctuations in environmental conditions, such as changes in osmolarity or acid-base balance. This adaptability allows glial cells to protect neurons from adverse effects that could arise from such changes. The unique function of glial cells in response to physiological shifts presents both advantages and challenges in understanding the full scope of their regulatory capabilities. Their plasticity can be beneficial in some scenarios, yet also a risk factor in pathological states.
In summary, the multifaceted functions of glial cells emphasize their role as indispensable components of the nervous system. Adequate support, effective communication, and maintenance of homeostasis are cornerstones of healthy brain function, further indicating the need for continued research into these critical cells.
Intercellular Communication Among Glial Cells
Intercellular communication among glial cells is a crucial aspect of their function in the brain. Unlike neurons, which communicate primarily through electrical impulses, glial cells often use chemical signals and electrical coupling to coordinate their activities. This communication is essential for maintaining homeostasis and supporting neuronal functions. A deeper understanding of these interactions unveils the complexity and significance of glial cells in the overall brain architecture.
Chemical Signaling Pathways
Chemical signaling pathways are fundamental for glial cells to share information with each other and with neurons. These pathways involve the release of signaling molecules, such as neurotransmitters and gliotransmitters. For example, astrocytes can release glutamate, which influences synaptic activity. Moreover, they can also produce other molecules like ATP and D-serine, contributing to neuronal signaling and plasticity.
The interplay between various glial cells occurs through this chemical communication. Microglia, for instance, respond to signals from astrocytes during an injury, indicating a damaged area that requires repair. This communication is not merely reactive; it also shapes developmental processes and governs responses to environmental changes.
Chemical signaling is not just about protection; it is also about enhancing communication and support within the neural network.
Electrical Coupling
Electrical coupling represents another layer of intercellular communication. Glial cells are connected by gap junctions, allowing ions and small molecules to pass directly between cells. This connection can facilitate rapid signaling across networks of glia. For instance, astrocytes can synchronize their activity, leading to coordinated responses to neuronal activity or changing conditions in the brain.
This coupling has implications for how efficiently glial cells can perform their roles. In situations where there is an emergency, such as an injury, the ability to convey signals quickly can be vital. Electrical coupling enhances the overall responsiveness of the glial network, allowing it to adapt to various physiological demands.
In summary, intercellular communication among glial cells through chemical signaling and electrical coupling is fundamental to their diverse roles in the brain. These interactions are key to understanding how glial cells manage to maintain neural health and support cognitive functions.
Glial Cells in Neurological Disorders
Glial cells play a crucial role in various neurological disorders, their functions extending far beyond mere support for neurons. Understanding the connection between glial cells and these disorders can lead to better insights into the underlying mechanisms of diseases that affect millions worldwide. This section will explore how glial cells contribute to both neurodegenerative and psychiatric disorders, highlighting their importance in each context.
Role in Neurodegenerative Diseases
Alzheimer's disease
Alzheimer's disease is characterized by progressive cognitive decline and memory loss, making it a significant area of focus in glial cell research. The accumulation of beta-amyloid plaques and tau tangles are hallmark features of this condition. Glial cells, particularly astrocytes and microglia, are involved in responding to these pathological changes.
Astrocytes play a vital role in clearing amyloid-beta, but in Alzheimer's, their function often becomes impaired. This dysfunction leads to a failure in removing toxic substances and contributes to increased inflammation. The inflammatory response mediated by microglia further exacerbates neuronal damage, highlighting a detrimental cycle where glial cells, although primarily supportive, end up participating in neurodegeneration. The study of glial cells in this context reveals how their activities can shift from protective to harmful, making it a pertinent subject in neurobiology discussions.
Multiple sclerosis
Multiple sclerosis (MS) is an autoimmune disorder that targets the central nervous system, leading to demyelination and various neurological symptoms. Oligodendrocytes are the principal glial cells affected in MS, as they are responsible for the formation of myelin sheaths around nerve fibers.
In MS, the immune system mistakenly attacks oligodendrocytes, disrupting their ability to produce myelin. This results in communication breakdown between neurons, which manifests as motor and cognitive impairments. The unique aspect of multiple sclerosis is its episodic nature, with periods of relapse and remission. Understanding the involvement of glial cells in MS can foster new therapeutic approaches aimed at protecting oligodendrocytes and restoring myelin.
Involvement in Psychiatric Disorders
Schizophrenia
Schizophrenia is a complex mental disorder characterized by symptoms such as hallucinations and delusions. Emerging research highlights the involvement of glial cells in its pathophysiology, particularly the dysfunction of astrocytes and microglia.
Astrocytes are known to regulate neurotransmitter levels, and their impairment can lead to an imbalance of dopamine, which is crucial in schizophrenia. Additionally, the altered activation of microglia has been linked to neuroinflammation and synaptic pruning processes that might contribute to the disorder. This connection makes schizophrenia a valuable case for exploring how glial cells influence mental health and offer potential avenues for new treatments.
Depression
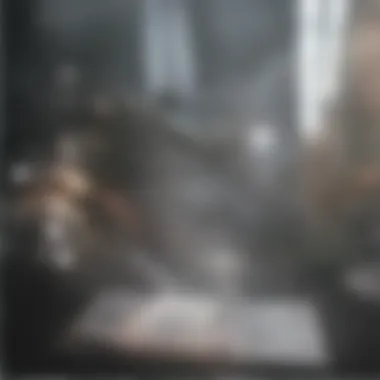
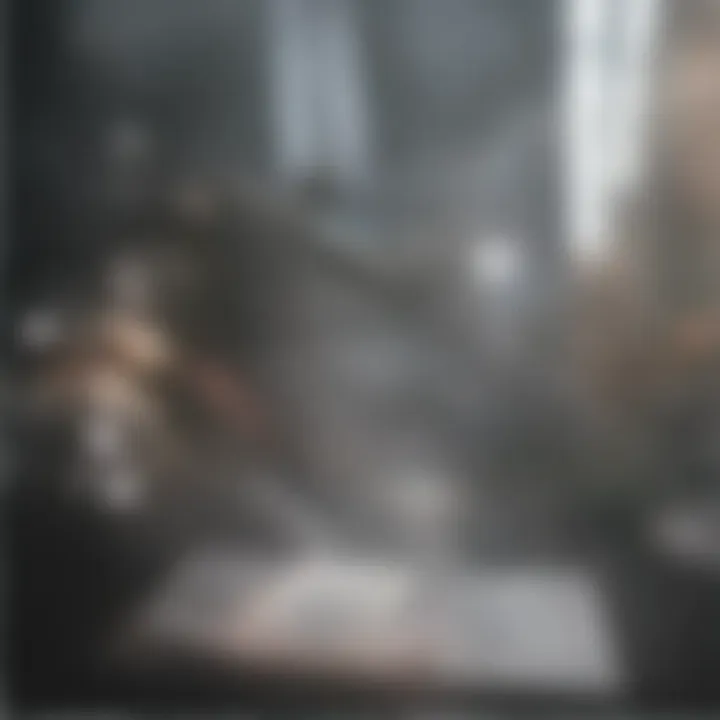
Depression, one of the most prevalent psychiatric disorders, has also been connected to glial cell activity. Several studies suggest that inflammatory processes involving microglia contribute to the development and persistence of depressive symptoms.
The key characteristic of depression in this context is the observed increase in pro-inflammatory cytokines, which can affect the functioning of neurons and glial cells. Moreover, alterations in dopamine and serotonin systems, both influenced by glial cells, further complicate the pathophysiology of the disorder. By examining glial cells' roles in depression, we can develop a richer understanding of how mental health is intertwined with glial cell dynamics.
The Future of Glial Cell Research
The exploration of glial cells is entering an exciting era, with numerous advancements paving the way for new understanding and applications. The future of glial cell research holds promise not just in basic science but also in translational medicine. As we delve deeper into how these cells function, we can identify their potential roles in various neurological conditions. This is particularly relevant as we consider innovative techniques that can further our comprehension of glial cells and their interactions within the nervous system.
Emerging Techniques in Study
Imaging technologies
Imaging technologies are at the forefront of modern neuroscience, providing dynamic insights into glial cell behavior. These methods, particularly those like two-photon microscopy and magnetic resonance imaging, allow researchers to visualize glial cells in real-time within live tissues. This provides significant advantages over traditional methods, which often rely on fixed specimens that do not capture the activity of these cells under physiological conditions.
The key characteristic of imaging technologies is their ability to gather detailed images of cellular processes as they occur. This allows for a more comprehensive understanding of glial cells in both health and disease. A major benefit is the capacity to monitor changes over time, revealing how glial cells respond to various stimuli.
However, imaging technologies also present challenges. For instance, the need for sophisticated equipment and the complexity of data analysis can limit accessibility for some labs. Despite this, the advantages largely outweigh the drawbacks, making these techniques a valuable part of future research.
Genetic manipulation
Genetic manipulation techniques, such as CRISPR-Cas9, are transformative tools in studying glial cells. They allow researchers to alter specific genes in these cells, thereby examining the consequence of those alterations. This specificity enables targeted studies on how changes in glial cell genetics influence neural functioning and pathology.
A notable feature of genetic manipulation is the precision it offers. Researchers can knock out genes or insert new sequences to assess their roles in glial function. This contributes significantly to identifying genetic factors linked to neurological disorders.
Despite the promise, genetic manipulation does raise ethical considerations. Potential off-target effects or unintended consequences pose risks that researchers must navigate carefully. However, the opportunity to delve deeper into genetic roles in glial cells makes genetic manipulation an essential focus for the future.
Potential Therapeutic Targets
Drug development
The study of glial cells is crucial for drug development aimed at treating neurological disorders. Identifying glial cell-specific pathways can lead to novel therapeutic agents that target these critical players in the brain. The focus on glial cells offers a unique approach, particularly for conditions like Alzheimer's disease and multiple sclerosis, where glial functionality is often compromised.
A major benefit of targeting glial cells is the possibility of addressing underlying causes of disease, rather than just managing symptoms. This could lead to more effective and long-lasting treatments. Current research is actively exploring drugs that can modulate glial cell responses or repair their functions.
However, challenges in drug delivery to the brain, along with potential side effects, remain a concern. Future drug development efforts must continue to consider these factors to enhance efficacy and safety, paving the way for innovative therapies.
Stem cell therapies
Stem cell therapies present another promising avenue in glial cell research. These therapies aim to restore or replace damaged glial cells in the nervous system, offering hope for conditions that currently have limited or no treatments. The unique aspect of stem cell therapies is their regenerative potential, which could help in repairing the intricate networks within the brain affected by disease.
The main advantage of stem cell therapies is their ability to regenerate inflammatory processes and create new glial cells where they are deficient. This not only addresses symptoms but may also reverse some neurological damage.
Nonetheless, challenges persist in ensuring the safe integration of stem cells into existing neural circuits and avoiding tumor formation. Researchers must address these issues thoroughly as they work towards clinical applications.
The future of glial cell research continues to evolve, offering exciting possibilities for both understanding and treatment of neurological disorders. Through emerging techniques and innovative therapeutic targets, we may unlock new pathways for restoring brain health.
The End
The conclusion of this article serves as a synthesis of the critical insights regarding glial cells. It emphasizes their importance in the broader context of neurobiology. While neurons have traditionally received most attention, glial cells are central to understanding brain function. Their multifaceted roles cannot be overstated.
Glial cells are not just supporting players in the brain's architecture; they actively participate in numerous functions. They regulate neurotransmission, maintain the blood-brain barrier, and respond to injury. They also contribute to energy metabolism and the overall homeostasis of the brain environment. Understanding these functions is essential for grasping how the entire central nervous system operates.
By recognizing the importance of glial cells, we open new avenues for research. Their involvement in neurological disorders, such as Alzheimerβs disease and schizophrenia, highlights the potential for discovering novel therapeutic targets. Research in this area is imperative as it may lead to innovative treatments that could improve outcomes for patients.
In summary, this article has aimed to not only highlight the roles of glial cells but also to reiterate their fundamental contributions to neural health. The future of neuroscience may well depend on our ability to appreciate and further explore the complexities of these remarkable cells. Therefore, fostering a deeper understanding of glial cells is not just beneficial; it is essential for the advancement of neuroscience as a whole.
Recapitulating the Importance of Glial Cells
Glial cells are essential components of neural tissue and their roles encompass support, defense, and active participation in signaling mechanisms. In recapping their importance, several factors become evident:
- Support and Nutrition: They provide essential nutrients to neurons and maintain an optimal environment for neural activity.
- Regulatory Functions: Glial cells help regulate ion concentrations and neurotransmitter levels, ensuring neurons can fire effectively.
- Immune Response: As the immune defenders in the central nervous system, microglia protect against pathogens and clear debris, playing a vital role in maintaining brain health.
- Facilitation of Neural Communication: They are involved in gliotransmission, which influences synaptic plasticity and neuronal communication.