Understanding My Genetic Code: Implications and Insights
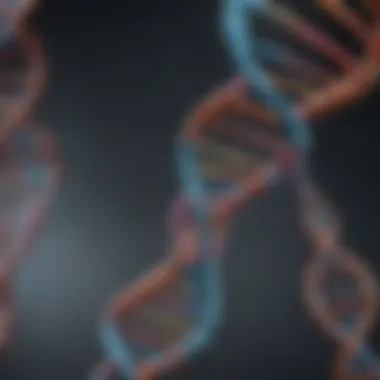
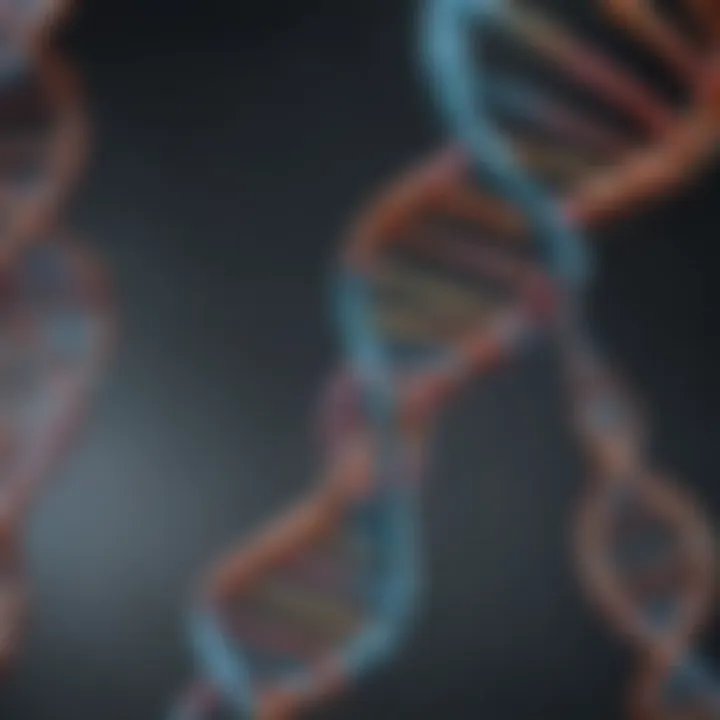
Intro
In recent years, the study of genetic codes has gained substantial prominence. Our genetic code contains the instructions that guide the development of all living things. Unlocking its secrets reveals much more than biological characteristics; it potentially shapes an individual's health, well-being, and even their identity. The ongoing advancements in genomic research offer profound insights into not just what makes us, but also how we can improve our health based on our unique genetic make-up.
This article will explore these layers of complexity, drawing on recent findings and innovations in the field of genomics. We will delve into the implications of genetic understanding for personalized medicine, the ethical challenges it presents, and how this all ties into our evolutionary journey. By the end of this exploration, readers will have a more nuanced understanding of their genetic code and its relevance in today's society.
Key Research Findings
Overview of Recent Discoveries
Recent research has broadened our understanding of genetic information. Key advances include developments in CRISPR technology and an enhanced focus on genome sequencing. For instance, scientists have shown that targeted genome editing can potentially eliminate genetic disorders. Furthermore, the Human Genome Project has laid the groundwork for identifying genes associated with various traits and diseases.
Many breakthroughs are emerging in the realms of epigenetics, where gene expression can be modified without changing the underlying DNA sequence. These findings highlight the intricate layers of control that contribute to how genes express themselves in an organism.
Significance of Findings in the Field
The implications of these discoveries are vast. Personalized medicine is one key area that stands to benefit. By understanding an individual's genetic makeup, treatments can be tailored for efficacy and minimized side effects. For instance, oncology has seen strides where specific therapies target genetic mutations within tumors, increasing success rates in patient treatment.
Moreover, the ethical implications of genetic testing are increasingly critical. We must consider data privacy, genetic discrimination, and informed consent in this era where genomic data is becoming more accessible. Establishing guidelines for responsible usage of genetic information is essential to protect individual rights while advancing scientific knowledge.
Breakdown of Complex Concepts
Simplification of Advanced Theories
Genetic concepts, while essential, can often be intricate. For instance, the basic structure of DNA consists of nucleotides - adenine, thymine, cytosine, and guanine. Understanding how combinations of these nucleotides serve as the blueprints for proteins is fundamental in genetic science. By simplifying the explanation of processes like transcription and translation, we can make these ideas accessible to a broader audience.
Visual Aids and Infographics
Visual representations can aid comprehension in genetic discussions. Infographics demonstrating the process of DNA replication, or comparing normal genes with mutated versions can assist learners in grasping these complex ideas. Images can break down the stages of genetic expression and help visualize the relationships between genes, proteins, and traits.
"Unlocking genetic codes is not just about understanding biology. It's about grasping how we can influence health and enhance our lives through our own genetic data."
Preface to Genetic Code
The genetic code serves as the blueprint for life, dictating how organisms develop, function, and evolve. This section focuses on the significance of understanding the genetic code, considering its implications for health, diversity, and the intricacies of biological systems. Recognizing how genes influence traits can empower individuals with knowledge about their own biology and potential health risks.
In recent years, advances in genetic research have unveiled new pathways for understanding genetics. The implications penetrate various fields, from medicine to anthropology. As geneticists decode sequences, they reveal factors affecting everything from physical characteristics, to disease susceptibility. The narrative around genetic code also involves ethical debates about privacy and identity that resonate in both personal and societal spheres. Although understanding it seems daunting, breaking down the complex nature of genetics brings clarity and relevance to many, enhancing both personal and collective insights into what makes us who we are.
Defining Genetic Code
Genetic code refers to the system that cells use to translate the information stored in DNA into proteins. These proteins are vital for cellular functions and dictate the physical traits of an organism. The code consists of sequences of nucleotides grouped into codons, which correspond to specific amino acids. In simpler terms, the genetic code directs the synthesis of proteins, effectively regulating growth and reproduction processes across all living things.
Key Characteristics of the Genetic Code:
- Universal: Found in all living organisms, indicating a common origin.
- Redundant: Multiple codons can code for the same amino acid, which can provide a buffer against mutations.
- Non-overlapping: Codons read sequentially and not in an overlapping manner, maintaining the fidelity of protein synthesis.
The implications of the genetic code extend far beyond mere biology. They shape our understanding of evolution, human behavior, and disease mechanisms.
Historical Context and Discovery
The history of the genetic code traces back to pivotal scientific breakthroughs. In the early 20th century, Gregor Mendel's work with pea plants laid the foundation for genetics by identifying how traits are inherited. The intricate relationship between DNA, genes, and proteins began to emerge alongside advancements in biochemistry and molecular biology.
In the 1950s, Watson and Crick proposed the double helix structure of DNA, illuminating how genetic information is stored. Then, in the 1960s, researchers worked to decode the sequences of amino acids in proteins, leading to the understanding of triplet codons. This laid the groundwork for deciphering the genetic code, ultimately revealing how biological information is transferred from generation to generation.
"The discovery of the genetic code was not just an achievement in molecular biology; it unlocked a new realm of understanding about life itself."
Over the decades, rigorous research and collaboration have continued to illuminate genetic relationships, driving the field forward. With modern techniques like gene editing and sequencing, we now stand on the brink of profound advancements that may reshape health and identity.
Understanding our genetic code is not only an academic pursuit. It directly informs medical strategies and personal decisions, increasing its relevance in today's world.
The Structure of DNA
The structure of DNA is a foundational concept in genetics. Understanding what DNA is and how it functions is crucial for grasping the implications of genetic codes. DNA contains the instructions for life, guiding the development and functioning of all living organisms. Its structure directly relates to its ability to store and transmit genetic information.
Double Helix Formation
The double helix structure of DNA was first described by James Watson and Francis Crick in 1953. This specific shape consists of two polynucleotide strands that coil around each other. Each strand is made up of a sugar-phosphate backbone, providing structural support and stability. The two strands are held together by base pairing, where the nitrogenous bases adenine pairs with thymine, and cytosine pairs with guanine. This specific pairing is important because it ensures that genetic information is accurately replicated during cell division.
The double helix shape is not just an aesthetic feature; it plays a significant role in the functionality of DNA. The grooves formed by the twisting of the strands allow proteins to access the genetic information for processes like transcription and replication. It is this intricate structure that allows DNA to be tightly packed in the nucleus of cells while remaining accessible for biological processes.
"The double helix is not just a shape; it is a symbol of the complexity and delicacy of genetic information."
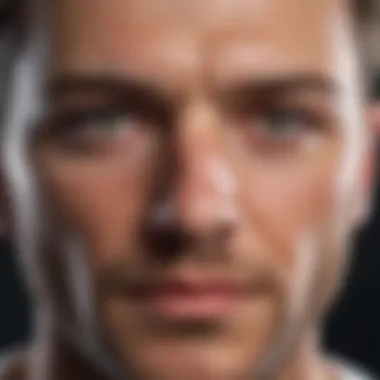
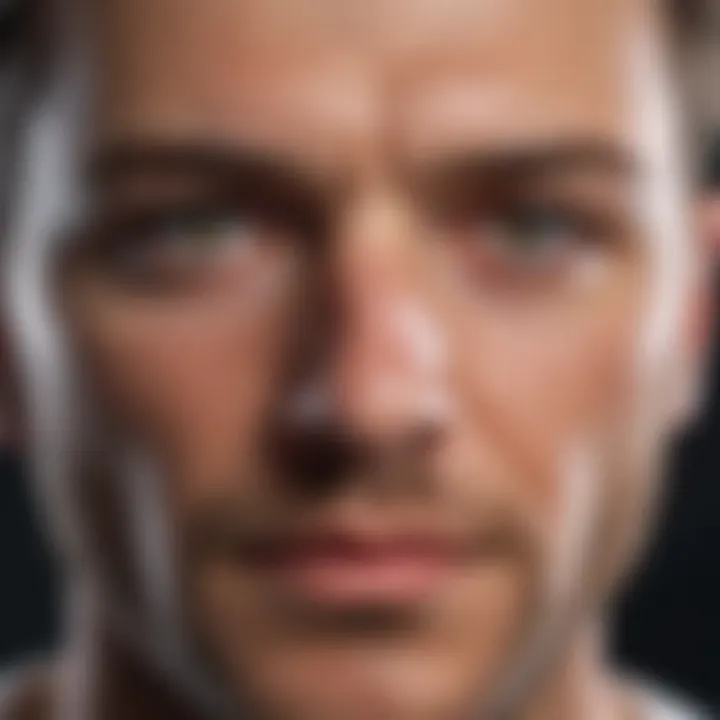
Nucleotide Composition
Nucleotides are the building blocks of DNA, and each consists of three components: a nitrogenous base, a deoxyribose sugar, and a phosphate group. There are four types of nitrogenous bases in DNA: adenine, thymine, cytosine, and guanine. The variation in the sequence of these nucleotides encodes the genetic information necessary for developing and maintaining organisms.
The nucleotide composition also determines the unique aspects of an individual’s genetic code. Mutations can occur if there are changes in the nucleotide sequence. These changes can have a variety of effects, from harmless variations to significant health implications. The study of nucleotide composition is essential not only for understanding genetic disorders but also for advancements in genetic therapies and personalized medicine. By recognizing the specific sequences that contribute to various traits, researchers can better strategize in treating genetic conditions.
In summary, the structure of DNA, formed by a double helix and made from specific nucleotide sequences, is fundamental to the understanding of genetic codes. This knowledge is relevant to a wide array of fields, including medicine, biology, and genetics research, all of which benefit from this understanding.
Mechanisms of Gene Expression
Gene expression is a fundamental process that translates genetic information stored in DNA into functional proteins. Understanding mechanisms of gene expression not only provides insight into how genes control biological functions but also reveals how variations in this process can lead to diseases. This section focuses on the critical elements of gene transcription and translation, which together allow cells to interpret genetic codes and produce the necessary proteins for life.
Transcription Process
Transcription is the initial step in gene expression where a specific segment of DNA is copied into messenger RNA (mRNA). This process is vital as it determines which proteins are produced in a cell. During transcription, the enzyme RNA polymerase binds to a promoter region of DNA, initiating the synthesis of mRNA by unwinding the DNA double helix.
The importance of transcription can be summarized as follows:
- Gene Regulation: Transcription is tightly regulated. Factors influencing transcription include transcription factors, enhancers, and silencers that can promote or inhibit RNA polymerase activity. This regulation is essential for cellular response to different environments and stimuli.
- Variability in Expression: Differences in transcription can lead to varying levels of gene expression among cells. This variability is crucial for processes like differentiation, where cells develop distinct functions.
- Role in Disease: Abnormalities in transcription can result in diseases. Mutations in regulatory regions can lead to uncontrolled cell growth, contributing to cancer development.
The transcription process can be broken down into several key steps:
- Initiation: RNA polymerase binds to the DNA promoter.
- Elongation: RNA polymerase moves along the DNA template strand, synthesizing mRNA.
- Termination: RNA polymerase reaches a termination signal, releasing the newly formed mRNA.
This mRNA then undergoes processing, including capping, polyadenylation, and splicing, ensuring that only the necessary coding sequences are preserved.
Translation Mechanism
After transcription, the next phase of gene expression is translation, where the mRNA is used to build proteins. This process occurs in the ribosomes, the cellular machinery responsible for synthesizing proteins.
Translation involves several important elements:
- Role of mRNA: The mRNA carries the genetic information from the nucleus to the ribosome, where it serves as the template for protein synthesis.
- Transfer RNA (tRNA): tRNA molecules bring the appropriate amino acids to the ribosome based on the codon sequence in the mRNA. Each tRNA molecule is specific to an amino acid and recognizes a specific codon on the mRNA.
- Ribosome Assembly: Ribosomes consist of ribosomal RNA (rRNA) and proteins. They facilitate the interaction between mRNA and tRNA, ensuring that amino acids are linked in the correct order to form a protein.
The translation process also includes three key stages:
- Initiation: The ribosome assembles around the start codon of the mRNA, and the first tRNA attaches.
- Elongation: tRNA molecules sequentially add amino acids to the growing polypeptide chain, extending the protein.
- Termination: When a stop codon is reached, the completed polypeptide chain is released.
The coordination between transcription and translation is critical for gene expression, as it ensures that proteins are made accurately and efficiently.
Understanding these mechanisms is critical in fields such as molecular biology and medicine. By grasping how genes are expressed, researchers can explore avenues for therapeutic interventions or innovations in biotechnology.
Genetic Variability and Mutations
Genetic variability and mutations are critical components of evolutionary biology and genetics. They provide the raw material for natural selection and influence how organisms adapt and survive in changing environments. Understanding this concept is essential in fields such as medicine, agriculture, and conservation. Those who study genetics can observe how mutations lead to diversity within species and how this diversity affects traits and survival.
Mutations are changes in the DNA sequence that may occur due to various factors including environmental influences or errors during DNA replication. They can lead to differences in traits among individuals in a population. These variations can be beneficial, neutral, or harmful, depending on their effects on the organism's fitness.
The role of genetic variability is fundamental for a population's adaptability and resilience. In a rapidly changing world, for example, a population with greater genetic diversity may withstand challenges like diseases or climate shifts better than a genetically uniform group. This adaptive potential highlights the importance of understanding how genetic variability arises and its implications on a broader scale.
"Genetic variability is the cornerstone of evolution; it fuels the mechanisms through which natural selection operates."
Types of Mutations
Mutations can be classified in several ways, including their origin or their impact on the organism. Common types of mutations include:
- Point mutations: These are changes in a single nucleotide and can result in a different amino acid being incorporated into a protein or even a premature stop codon.
- Insertions and deletions: These alterations add or remove nucleotides from the DNA sequence. They can shift the reading frame of a gene, potentially leading to significant changes in the protein produced.
- Copy number variations: These involve duplications or losses of large sections of DNA, which can influence overall gene dosage and expression levels.
- Chromosomal mutations: These mutations affect the structure or number of chromosomes, impacting multiple genes simultaneously.
Each of these types of mutations contributes uniquely to genetic diversity. They may arise from spontaneous errors in DNA replication or be induced by environmental factors, such as radiation or chemicals.
Impact on Phenotype
The phenotypic consequences of mutations can be profound. Phenotype refers to the observable traits of an organism, which can include morphology, development, biochemical properties, and behavior. When mutations occur in coding regions of genes, they can alter the function of the resulting proteins, resulting in changes to an organism's traits.
For instance, a single point mutation in a gene responsible for pigmentation can lead to variations in color patterns in a population of animals. Such changes can affect how these organisms interact with their environment, including their ability to find mates or evade predators.
Moreover, not all mutations manifest externally. Some may influence internal processes, such as metabolism or susceptibility to diseases. The impact of mutations thus extends beyond individual organisms, shaping populations over generations. Understanding these mechanisms can yield insights into evolutionary processes and inform areas like personalized medicine, where the knowledge of specific mutations can guide therapeutic interventions.
By studying the nuances of genetic variability and the implications of mutations, researchers continue to uncover the intricate tapestry of life, revealing how diversity fuels adaptation and evolution.
Ethical Considerations in Genetics
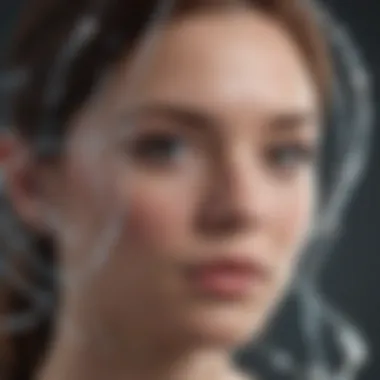
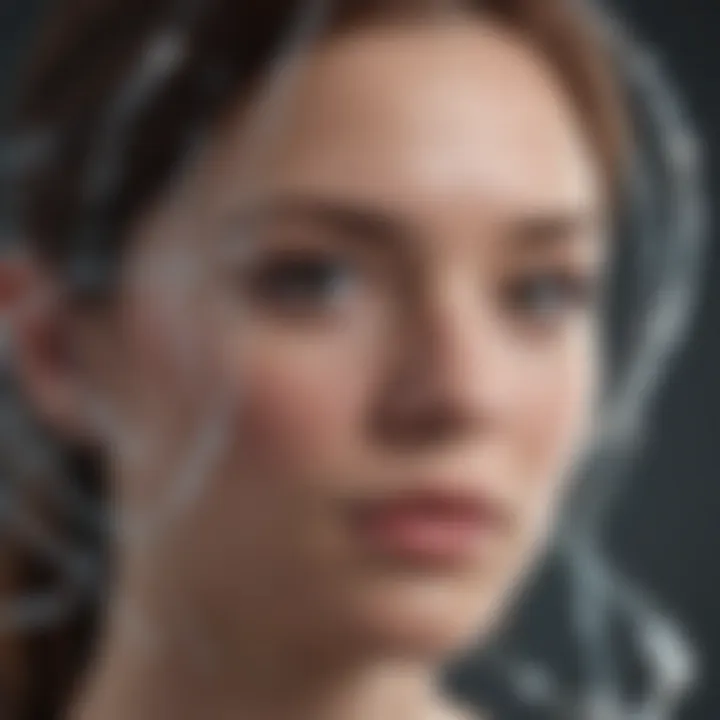
Ethical considerations in genetics are paramount in today's rapidly evolving landscape of genetic research and application. As we uncover and manipulate the intricacies of the human genome, the implications of such discoveries extend far beyond scientific curiosity. They touch on profound issues such as privacy, identity, and social equity. This section will explore these elements, focusing on the importance of addressing ethical dilemmas that arise in genetic research and healthcare, ensuring that advancements benefit all of society without compromising individual rights or well-being.
Genetic Privacy
Genetic privacy refers to the right of individuals to control access to their genetic information. In an era where personal data can be easily shared and stored, the protection of genetic information has become increasingly crucial. Sensitive information related to one’s genetic makeup can reveal predispositions to certain diseases, ancestry, and even traits that form part of one’s identity.
The importance of genetic privacy is underscored by several factors:
- Potential for Discrimination: Knowledge about genetic predispositions can lead to discrimination by employers, insurance companies, or even society at large. An individual may face unjust limitations based on information that, while informative, should not define their character or capabilities.
- Informed Consent: Individuals should have autonomy over their genetic data. When genetic samples are collected for research, it is vital that consent be informed and specific. People should know how their data will be used, stored, and shared.
- Legislation: Various regulations, such as the Genetic Information Nondiscrimination Act in the United States, seek to safeguard against misuse of genetic data. However, continuous updates to legislation are essential as technology evolves.
"The complexities surrounding genetic data underscore the need for robust legal frameworks to protect individuals and maintain ethical standards in genetics."
Gene Editing Controversies
Gene editing techniques, like CRISPR, present groundbreaking potential for curing diseases and enhancing human traits. However, these advancements also generate substantial ethical debates. The controversies surrounding gene editing primarily focus on the following aspects:
- Manipulation of Human Embryos: Editing genes in embryos raises questions about the long-term impacts on future generations. The potential to alter human evolution is profound, and societies must grapple with the moral implications.
- Designer Babies: The ability to select or enhance traits in offspring leads to discussions about societal inequality. If only a privileged few can afford such technologies, it may create a divide between those who can access enhancements and those who cannot.
- Ecological Impact: Editing genes in organisms, particularly in agriculture, could have unpredictable effects on ecosystems. It is essential to consider both the benefits of increased crop yields and the possible disruptions to natural systems.
Emerging Technologies in Genomics
The rapid advancement of technology has transformed the field of genomics, enhancing our understanding of genetic material in ways once thought impossible. Emerging technologies are not just about examining the genome; they significantly impact health, disease treatment, and our understanding of life itself. Key facets of these technologies include precision, efficiency, and capability to decipher complex genetic information at an unprecedented scale.
Moreover, the consideation of these innovations showcases how they interact with ethical questions and regulatory frameworks. It is vital to remain aware of both the profound benefits and potential risks that accompany these transformative tools in genetics.
CRISPR and Genome Editing
CRISPR, which stands for Clustered Regularly Interspaced Short Palindromic Repeats, represents a groundbreaking revolution in genome editing. This tool allows precise modifications to DNA in living organisms. The mechanism is based on the natural defense system found in bacteria that protects against viral infections. By utilizing CRISPR, scientists can cut DNA at targeted locations, leading to gene alterations that can correct genetic disorders or enhance desirable traits.
The impact of CRISPR on genetic research is profound:
- Gene Therapy: CRISPR can potentially treat genetic disorders, such as sickle cell disease, by correcting mutations.
- Agricultural Advancements: It can be used to create crops with improved traits, like resistance to diseases.
- Research Efficiency: Scientists use CRISPR to model diseases more efficiently in laboratory settings.
However, this technology raises questions about safety, especially regarding off-target effects where unintended parts of the genome are altered. Regulatory insight is needed to ensure that the applications of CRISPR do not lead to unforeseen consequences. As of now, ongoing research strives to refine techniques and minimize risks.
Next-Generation Sequencing
Next-Generation Sequencing (NGS) has fundamentally redefined how we sequence DNA and RNA. Unlike traditional methods that required significant time and resources, NGS enables rapid sequencing of entire genomes at a fraction of the cost. This efficiency allows researchers to explore genetic variations with remarkable depth and precision.
The relevance of NGS encompasses:
- Whole Genome Sequencing: This enables a comprehensive view of the genome, facilitating deeper understanding of genetic diseases.
- Exome Sequencing: Focuses on the protein-coding regions, thus identifying variants that could affect protein functions.
- Personalized Medicine: By sequencing an individual’s genome, healthcare providers can tailor medical treatments based on a person’s unique genetic makeup.
As researchers gather more data through NGS, it encourages collaboration and opens pathways for innovations in disease prevention and treatment. Yet, the vast amount of data also necessitates advancements in bioinformatics to process and interpret results effectively. Maintaining ethical standards in data handling remains crucial as the capabilities continue to expand.
Emerging technologies like CRISPR and NGS hold promise for bridging gaps in our understanding of genetic codes, ultimately leading to heightened insights in health and identity.
In summary, the significance of emerging technologies in genomics cannot be overstated. Innovations like CRISPR and NGS are reshaping the landscape of genetic research and healthcare, paving the way toward a future rich with possibilities. Maintaining a balance between innovation and ethical responsibility will be vital as the field continues to evolve.
Personalized Medicine
Personalized medicine stands at the intersection of genetics and healthcare, bringing forth a revolution in how medical treatments are approached. This field tailors healthcare specifically to individual genetic profiles, ensuring that treatments are not just effective but also efficient and well-suited to each patient. With the rapid advancements in genomic technologies, personalized medicine has begun to redefine the landscape of healthcare by offering unprecedented opportunities for optimizing treatment protocols.
One of the key elements of personalized medicine is the integration of genetic information into clinical practice. By understanding the genetic basis of diseases, healthcare providers can predict individual responses to various treatments. This allows for a more precise approach to prescribing medications, which can lead to improved patient outcomes. For instance, patients with certain genetic markers may respond better to specific drugs, while others may experience adverse effects. Recognizing these nuances is critical for the development of effective treatment plans.
The benefits of personalized medicine extend beyond individual patients to health systems as a whole. Cost-effectiveness is a significant consideration. When treatments are customized to align with genetic profiles, there is a reduced likelihood of trial-and-error prescribing. This minimizes wasted resources on ineffective treatments, ultimately fostering a more economical healthcare system. Furthermore, personalized medicine encourages preventive health measures. By identifying genetic predispositions to certain conditions, proactive steps can be undertaken to mitigate risks, potentially lowering the incidence of diseases in the broader population.
However, the rise of personalized medicine also comes with challenges. Ethical considerations around genetic information privacy must be addressed. How personal data is stored and shared poses a significant concern for patients who may be apprehensive about their genetic data being exposed. Transparency and robust regulatory frameworks are paramount to ensure trust in this emerging approach.
In summary, personalized medicine is reshaping the future of healthcare, guided by genomic insights that promise to enhance treatment efficacy and patient outcomes while also streamlining healthcare costs. As this field evolves, collaboration between genomic researchers, healthcare professionals, and patients will be essential to navigate ethical challenges and harness the full potential of personalized medicine.
Role of Genomics in Healthcare
Genomics plays a pivotal role in personalized medicine. By analyzing an individual's genetic makeup, healthcare providers can tailor medical treatments and interventions specifically to their unique biological characteristics. This area of medicine is focused on not just treating diseases, but also predicting them. Genomic sequencing technology enables the identification of genetic predispositions to various conditions, allowing for targeted prevention strategies.
Through genomic studies, healthcare can achieve a more profound understanding of complex diseases such as cancer, diabetes, and cardiovascular diseases. Identifying specific mutations or variations can assist in designing customized treatment plans. Additionally, genomics helps in determining the right drug and dose for each individual, potentially increasing the effectiveness of medications while diminishing the risk of harmful side effects.
"Genomics enables us to move from a one-size-fits-all approach to a more tailored strategy that respects individual differences in genetic makeup."
As genomics continues to advance, its role in healthcare will likely expand, leading to more precise diagnostics and interventions.
Pharmacogenomics
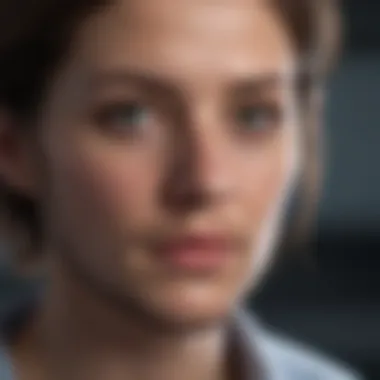
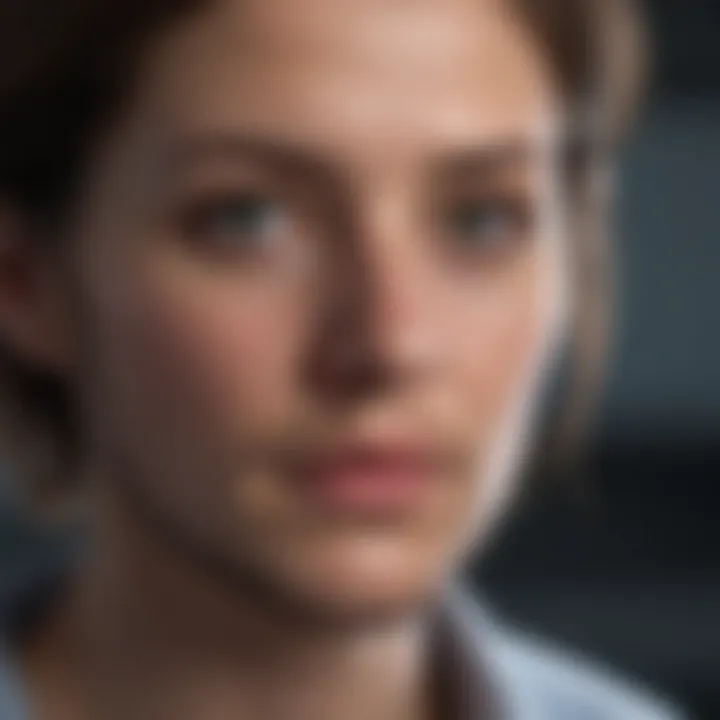
Pharmacogenomics is a specialized subset of genomics that investigates how genes affect individual responses to drugs. This field is crucial for personalized medicine as it helps in understanding why certain medications work for some people but not for others. By analyzing specific genetic variations, pharmacogenomics provides insights into the best therapeutic strategies for individuals based on their genetic profiles.
For instance, individuals with variations in the CYP2D6 gene may metabolize certain antidepressants differently, necessitating dosage adjustments. Pharmacogenomic data can inform healthcare providers on which medications may be most effective and safe, based on the patient’s genetic makeup. This not only enhances therapeutic efficacy but also prioritizes patient safety, reducing the incidence of adverse drug reactions.
Moreover, pharmacogenomics significantly contributes to the field of oncology. It allows for the selection of targeted therapies that align with the genetic characteristics of a patient’s tumor. This precision medicine approach leads to better management of cancer treatments and outcomes.
The ongoing integration of pharmacogenomics into clinical practice continues to unfold new avenues for enhancing personalized medicine, presenting both clinical and ethical considerations. As pharmacogenomic testing becomes more accessible, healthcare systems must prepare for changes in how medications are prescribed and managed. This dialogue between genomics and pharmacology ensures that healthcare will be increasingly individualized.
The Future of Genetic Research
The realm of genetic research is on the cusp of unprecedented advancements. This section highlights the importance of understanding these innovative developments. Research in this area does not only enhance our understanding of human biology, but also transforms medical practices and therapeutic approaches. The future of genetic research promises to deepen our insights into health, disease, and personal identity.
Innovative Frontiers in Genomics
Innovations in genomics are paving the way for breakthroughs in several key areas.
- Gene Therapy: This technique aims to resolve genetic disorders by correcting defective genes. Accessing and modifying genes may lead to cures for conditions previously deemed untreatable.
- Synthetic Biology: This discipline involves redesigning organisms for useful purposes. It combines principles of engineering and biology, potentially leading to novel solutions in medicine, agriculture, and environmental management.
- AI in Genomics: Artificial intelligence is being used to analyze vast datasets, discover genetic signatures, and predict disease susceptibility. By processing information faster and more efficiently, AI can identify patterns that humans might overlook.
- Single-Cell Genomics: This cutting-edge approach enables researchers to analyze the genetic material of individual cells. It provides insights into cellular diversity and functions, vastly improving our understanding of complex biological processes.
As these fields advance, they will likely result in new therapeutic strategies and diagnostic tools that personalize healthcare on an unmatched scale.
Global Collaborative Efforts
Collaboration on a global scale is essential for the acceleration of genetic research. International partnerships bring together diverse expertise and resources, allowing for more comprehensive research initiatives.
- Large-Scale Projects: Initiatives such as the Human Genome Project have demonstrated the power of collaborative efforts. By sharing knowledge and discoveries, these projects lead to significant advancements across the globe.
- Data Sharing Platforms: Researchers are establishing platforms to share genomic data freely. This open-access approach promotes transparency and speeds up the discovery of genetic associations. Open access can foster innovation and help address health disparities by extending knowledge to under-resourced areas.
- Standardized Protocols: Implementing standardized methodologies ensures that research findings are reliable and reproducible. Global consortia work towards unifying techniques and practices, enhancing the validity of results.
- Cross-Disciplinary Collaboration: Engaging experts from various disciplines, such as bioinformatics, ethics, and social sciences, brings a holistic view. This is necessary to navigate the complexities of genetic data usage and interpretation.
"The future of genetics will depend not only on scientific discovery but also on how well society adapts to the implications of those discoveries."
Genetics and Identity
Genetics serves as a crucial element in understanding various aspects of personal and collective identity. In contemporary society, individuals increasingly look to their genetic data to uncover insights about their health, ancestry, and even family dynamics. The exploration into genetics also raises significant questions regarding the implications of this information on self-perception and societal roles.
Genetic information is not merely a compilation of DNA sequences. It embodies narratives about ancestry, familial connections, lineage, and sometimes even cultural identity. As research advances, the ability to interpret genetic data has expanded, providing access to vast repositories of ancestral knowledge that can enrich personal context.
Furthermore, the implications of genetic testing overwhelmingly influence various contexts. In medical settings, understanding one's genetic predispositions can guide health decisions. For example, being aware of specific genetic markers might alert individuals to potential health risks, thus empowering proactive health management. Here, the intersection of genetics and identity becomes essential, as one's genetic makeup can impact lifestyle choices and medical decisions.
Understanding Ancestry
Recognizing one’s ancestry through genetic testing allows individuals to trace their roots back through generations. This understanding can provide clarity regarding geographical origins, migrations, and the interconnections that shape cultures. Genetic tests, like those offered by 23andMe and AncestryDNA, can reveal ethnic backgrounds and the genetic strands that link an individual to specific populations.
The process of exploring ancestry through genetics involves examining markers, known as Single Nucleotide Polymorphisms (SNPs), which vary between individuals. By analyzing these markers, one can obtain a breakdown of their genetic composition, revealing familial ties and cultural heritages. This knowledge can act as a bridge to understand personal narratives, enriching an individual’s sense of self and community.
Benefits of Understanding Ancestry
- Cultural Connection: Provides insight into familial traditions and cultural backgrounds.
- Health Awareness: Certain ancestry traits may highlight predispositions to specific health conditions.
- Social Networks: Connects individuals with distant relatives and shared heritage communities.
Impact on Personal Identity
Genetic insights have powerful ramifications on personal identity. The knowledge gained from genetic testing can lead to new understandings of oneself, often reshaping self-perception and sense of belonging. It offers an empirical framework for individuals to grasp their identity, often influencing how they relate to others.
The impact can be both empowering and disconcerting. Some individuals find strength in understanding their ancestry and genetics, leading to a stronger sense of social identity. Others may encounter challenging revelations about family dynamics or heritage, which can disrupt established self-concepts. This duality underscores the complex relationship between genetics and identity.
Moreover, genetics plays a role in forming personal beliefs and values. With insights gained from genetic information, individuals may explore questions of heritage, race, and nationality, weighing them against societal constructs. Such exploration can promote dialogue about identity in multicultural contexts.
"Understanding one's genetic code is a key to unlocking personal and collective identities that may otherwise remain hidden."
As the conversation surrounding genetics continues to evolve, so too does the understanding of its role in shaping identities. The dialogue around genetics and identity invites ongoing exploration and reflection, fostering a deeper appreciation for the complexities that lie within our genetic fabric.
Finale
The conclusion of this article aims to encapsulate the pivotal themes of understanding our genetic code. This section lays out the significance of genetic knowledge, which has become increasingly essential in today's world. The intricacies of genetic codes not only inform personal health decisions but also contribute to the wider discourse on identity and biology.
Summarizing Key Insights
Throughout the article, several key insights emerge:
- Complexity of Genetic Structures: Our DNA showcases a rich tapestry of information, highlighting the sophistication of biological systems.
- Role of Genetics in Health: Genetics plays a direct role in determining health risk factors, guiding personalized medicine approaches that consider individual genetic backgrounds.
- Ethical Implications: The advancements in genetics bring forth critical ethical considerations regarding data privacy and genetic manipulation.
- Future of Research: Ongoing research promises new technologies and applications that could revolutionize how we approach medicine and understand human biology.
These insights illustrate the profound impact genetics has on our lives, shaping our health choices, influencing our identity, and propelling scientific advancements.
The Path Forward in Genetics
As we look ahead, the future of genetics is promising yet complex. Several key areas warrant attention:
- Innovative Technologies: Tools like CRISPR enable targeted gene editing, fostering potential breakthroughs in treating genetic disorders. Continued research and ethical considerations in this realm remain paramount.
- Integration of Genomics in Health: Understanding how genomic data can be integrated into clinical practice will be crucial for enhancing patient care.
- Public Awareness and Education: Educating the public about genetic information is essential, particularly regarding its implications for health and ancestry.
"The exploration of genetic codes is not just a scientific endeavor; it is a journey that shapes our understanding of life itself."