Understanding General Relativity: Gravity, Space, and Time
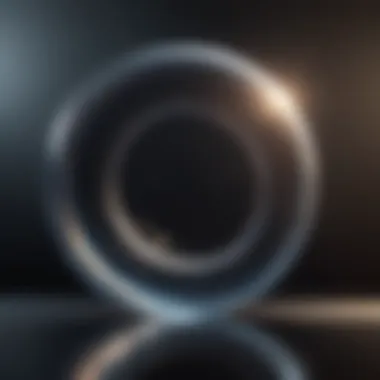
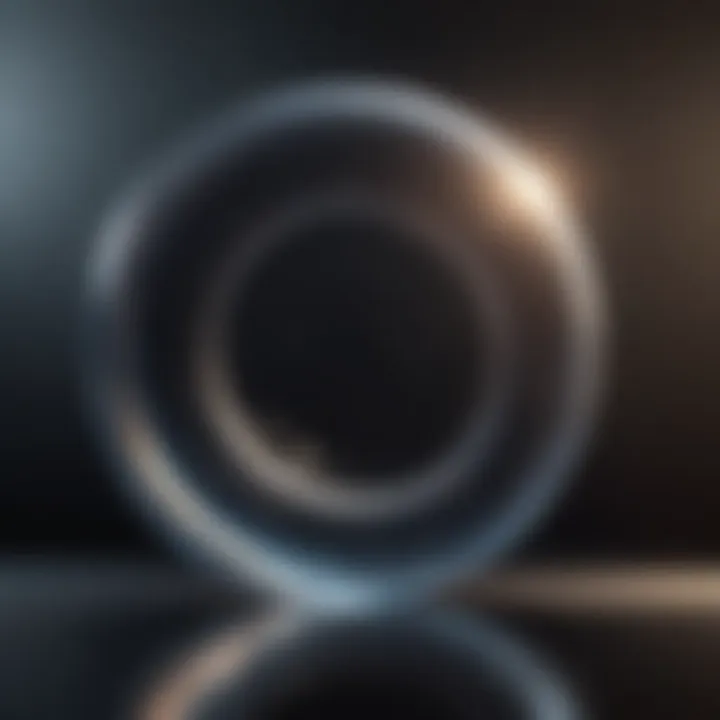
Intro
General relativity stands at the heart of modern physics, reshaping how we think about not just gravity but also the fundamental nature of time and space itself. Formulated by Albert Einstein in 1915, this theory has traversed nearly a century, fundamentally altering scientific inquiry and our understanding of the cosmos.
In essence, general relativity posits that gravity is not merely a force between masses but rather the result of how mass warps and curves the fabric of spacetime. This elegant concept has paved the way for groundbreaking discoveries in the fields of astronomy, cosmology, and theoretical physics.
The scope of this article is to delve deep into the intricacies of general relativity, pulling apart its core components while simultaneously connecting its practical implications in today's scientific landscape. Whether you are a student getting your feet wet in physics or an educator looking to enrich your teaching with profound concepts, this narrative will be instrumental in enhancing your comprehension.
Key Research Findings
Overview of Recent Discoveries
In recent years, a multitude of discoveries has emerged stemming from the principles of general relativity. For example, the detection of gravitational waves by LIGO in 2015 confirmed a key prediction of Einstein's theory. When two massive objects like black holes collide, they produce ripples in spacetime that can be measured on Earth. This not only validated general relativity further but also opened a new window into observing the universe.
Another significant finding is the observation of black holes, particularly the imaging of the Event Horizon Telescope in 2019, which offered the first visual confirmation of a black hole's existence. Such research continues to push the boundaries of science, illustrating the wonderfully unexpected consequences that follow from the study of gravitational effects in extreme environments.
Significance of Findings in the Field
The implications of these discoveries are far-reaching. By corroborating Einstein’s predictions, researchers have validated the theoretical groundwork laid over a century ago, altering our understanding of phenomena like time dilation and gravitational lensing. Every new find not only fortifies the validity of general relativity but also compels a deeper inquiry into the fabric of the universe itself. It establishes a robust framework for probing the dark components of the cosmos, like dark matter and dark energy, which play pivotal roles in the universe's expansion.
Breakdown of Complex Concepts
Simplification of Advanced Theories
General relativity can be a daunting subject for many. To break it down, the theory posits that massive objects cause spacetime to curve around them, which we perceive as gravity. Think of a trampoline with a heavy ball placed in the center; the fabric stretches and sinks under the weight, illustrating how planets and stars bend spacetime. This conceptual leap can seem challenging, but analogies like this can foster understanding.
Visual Aids and Infographics
In teaching these complex ideas, visual aids have proven invaluable. Simple diagrams can illustrate how gravitational waves travel through spacetime or how light is bent by massive objects. Infographics that reflect the interplay between mass and curvature can be particularly effective. Such resources simplify learning and provide an engaging way to visualize what might otherwise remain abstract.
Although general relativity is complex, breaking it down through analogies and visuals can illuminate its foundational ideas, making it more accessible to diverse audiences.
Preamble to General Relativity
General relativity, a cornerstone of modern physics, radically reinterprets the notion of gravity and its relationship with space and time. In this section, we will navigate the essential concepts and principles that lead to the development of this groundbreaking theory, setting a solid foundation for the discussions that follow. Its significance extends beyond mere academic curiosity; it influences technological advancements and deepens our understanding of the universe. The importance of exploring general relativity lies in its ability to challenge preconceived notions, providing fresh insights that resonate with scholars, students, and anyone fascinated by the cosmos.
The Historical Context
To truly appreciate the weight of general relativity, it’s vital to consider the historical backdrop against which it emerged. In the late 19th and early 20th centuries, physics was shaped by classical Newtonian mechanics, which described gravity as a force acting at a distance. However, as scientists grappled with the inconsistencies presented by electromagnetic phenomena and the behavior of light, a transformational shift was inevitable.
In 1905, Albert Einstein introduced the theory of special relativity, which transformed our understanding of time and space. Yet, Einstein recognized that something was amiss when it came to gravity. Newton's ideas fell short in explaining certain celestial behaviors, notably Mercury's orbit, which couldn't be perfectly accounted for using classical mechanics alone.
It was in 1915 that Einstein unveiled his theory of general relativity, proposing that gravity is not just a force; instead, it represents a curvature of spacetime caused by mass. This bold idea not only reconciled the anomalies seen in celestial mechanics but also provided a unified framework that illustrated how objects in the universe interact.
Key Figures and Their Contributions
While Einstein’s name will forever be linked to general relativity, he was not alone on this intellectual journey. Several scientists paved the way toward its conception. Here are a few pivotal contributors:
- Isaac Newton: Though his framework laid the groundwork for classical mechanics, it was Newton’s gravitational law that Einstein built upon, providing a contrasting perspective.
- Hermann Minkowski: A mathematician and physicist, Minkowski proposed the idea of four-dimensional spacetime, which provided the mathematical underpinning that Einstein used in his formulation.
- David Hilbert: Independently, Hilbert developed equations that were remarkably similar to Einstein's field equations, showing how interconnected the community of physicists was at that time.
- Arthur Eddington: His observational experiments during a solar eclipse in 1919 offered the first empirical evidence supporting Einstein's theory, turning general relativity into a cornerstone of modern physics.
"General relativity is one of the towering achievements of human thought, reshaping our understanding of the universe in profound ways."
These individuals and many others contributed significantly to the development of ideas that framed general relativity, enriching our understanding of the cosmos while establishing the base for further explorations in physics, including cosmology, black holes, and gravitational waves. The discourse around this topic continues today as we probe the depths of space with tools like gravitational wave detectors and large telescopes, seeking to untangle the universe's mysteries.
Foundational Concepts of General Relativity
In the grand tapestry of physics, the foundational concepts of general relativity serve as the very threads that weave together our understanding of the universe's fabric. This section details how space and time converge, forming a singular entity, alongside the revolutionary idea of gravity being an effect rather than a force. These ideas are not merely academic pursuits; they are essential for grasping the cosmos and understanding phenomena that occur on scales far beyond our everyday experiences.
Space and Time as a Unified Entity
The traditional view of space and time as separate realms has been thoroughly transformed by Einstein's insights. In this theory, space and time amalgamate into a four-dimensional construct known as spacetime. Imagine it like a vast canvas where every event—past, present, and future—is painted. This alteration allows us to grasp how objects move and interact in a more holistic manner.
In essence, spacetime tells a story: the history of how objects behave under the influence of gravity. With this framework, rather than picturing an object just moving through space while time ticks away independently, we see that motion in space affects the flow of time. One of the most paradigmatic examples here is the phenomenon of time dilation, which can be vividly illustrated through a well-known thought experiment involving twins. If one twin hops on a spaceship and travels at near-light speed while the other stays home, upon returning, the traveling twin will have aged less. This counterintuitive outcome reveals that space and time are inextricably linked.
"The distinction between past, present, and future is only a stubbornly persistent illusion." – Albert Einstein
Through this lens, phenomena such as black holes become more understandable; they warp spacetime to such an extent that time literally runs differently near them. Embracing this concept not only helps to navigate our understanding of gravity but also opens the door to myriad insights about the universe, from the orbits of planets to the very nature of light.
Gravity as Curvature of Spacetime
Shifting gears to gravity's role, Einstein's theory paints it not as a force pulling objects together but rather as the curvature of spacetime caused by mass. Visualize placing a bowling ball on a trampoline. The fabric of the trampoline dips, creating a well. If you were to roll a marble across the trampoline, it wouldn’t move in a straight line but would follow the contours of that dip, spiraling inward towards the bowling ball. This is how mass bends spacetime, guiding the paths of other objects—this is gravity, according to general relativity.
This curvature explains why planets orbit stars and why galaxies cluster, revealing the nearly poetic dance of celestial bodies. When the mass is remarkably large, like that of a star or black hole, the curvature becomes pronounced, and the pathways that other entities follow are transformed in ways that can lead to surprising results. The famous prediction of light bending around massive objects arises from this understanding, where even light, the fastest entity in the universe, cannot escape this distortion of spacetime.
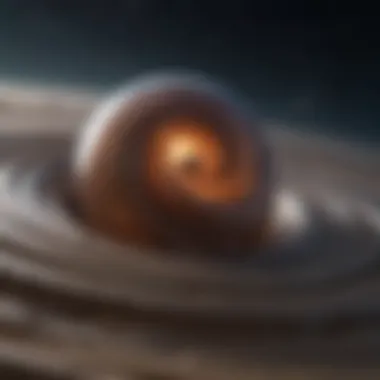
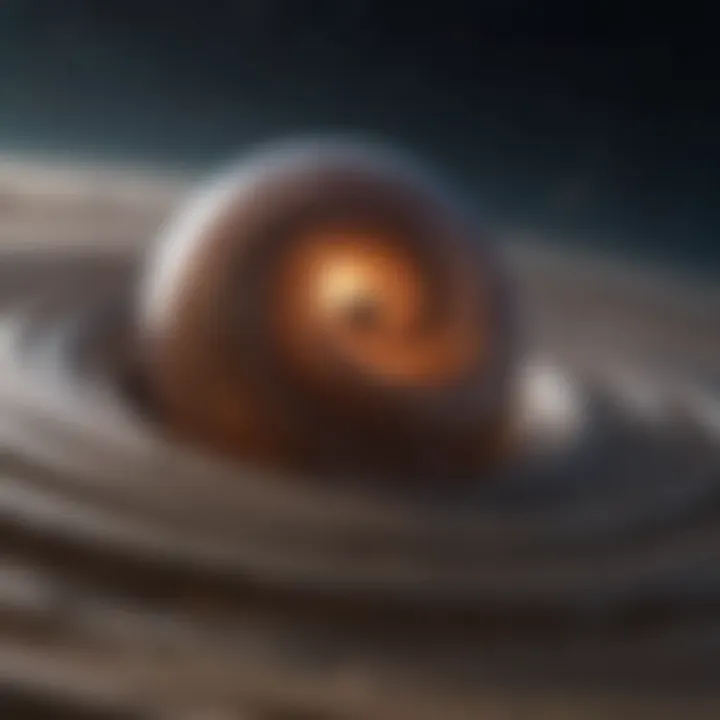
In practical terms, this notion of gravity as curvature has become a cornerstone for modern astrophysics. For example, when scientists detect gravitational waves, they are measuring ripples in spacetime caused by massive colliding objects, confirming predictions made over a century ago. Through experiments and observations, our comprehension of these foundational concepts reveals a universe that is intricate, interconnected, and yet surprisingly intuitive.
In summary, grasping these foundational concepts is crucial in not only contextualizing the implications of general relativity but also appreciating its pivotal role in shaping modern physics.
Mathematical Framework of General Relativity
Understanding the mathematical framework of general relativity is no walk in the park. It's not just an assortment of equations sprinkled together on a chalkboard; it’s a comprehensive system that, when understood, provides profound insight into the structure and behavior of the universe. This section will break down some of the core concepts behind the mathematics that Einstein put forth, unraveling the complex relationship between geometry and gravity.
Tensor Calculus Overview
Tensor calculus is the backbone of general relativity. At its core, this branch of mathematics deals with quantities that can be represented as arrays of numbers, called tensors. Tensors can describe a multitude of physical quantities, allowing us to bridge the gap between abstract mathematical concepts and real-world physics.
The way tensors interact with one another provides a powerful tool set, especially for analyzing systems involving multiple dimensions. For instance, when studying spacetime, the use of tensors permits the description of physical laws in a way that is independent of the choice of coordinate systems. This flexibility is crucial because it aligns with the principle of covariance in general relativity, which states that the laws of physics remain consistent regardless of the observer's frame of reference.
To put it plainly, tensor calculus not only gives us a language but also tools to communicate about the fabric of spacetime and gravity's role in it. It sets the stage for complex concepts and is essential in formulating Einstein's equations.
Einstein's Field Equations
Next up are Einstein's field equations, often considered the centerpiece of general relativity. Simply put, these equations relate the geometry of spacetime to the distribution of matter within it. The famed equation is expressed as:
What’s remarkable is how these equations unify gravity with the geometry of the universe. They predict that mass and energy curve spacetime, dictating how objects move. When you think about black holes, gravitational waves, and black holes—this is where the magic happens. Understanding this relationship helps us grasp not only how planets orbit stars but also how light bends around massive objects, illustrating that mass doesn't just influence motion but warps the very fabric of the universe itself.
Geodesics and Their Significance
Geodesics are another vital concept in this vast mathematical terrain. Think of a geodesic as the shortest path between two points, similar to a straight line on a flat surface. However, when we’re speaking of a curved surface—like the basketball-shaped model of Earth—it gets trickier. On the sphere, the shortest distance between two points is actually along the curve of the sphere’s surface, not a straight line in three-dimensional space.
In the context of general relativity, geodesics are essential for understanding how objects move through curved spacetime. Essentially, when an object is freely falling through gravity—like a satellite orbiting Earth—it traces a geodesic in the curvature created by the mass of Earth.
This is a groundbreaking perspective shift: gravity is not simply a force pulling objects together, as it was classically viewed, but rather a result of objects following the natural curvature of spacetime. Recognizing the significance of geodesics in motion informs gravitational concepts and has dramatic implications, from GPS accuracy to understanding structure formation in the universe.
"The real surprise of Einstein's theory is that gravity is not a force in the usual sense, but a manifestation of the geometry of spacetime itself."
In summary, the mathematical framework of general relativity is a blend of elegant mathematics and riveting physics. It empowers scientists to decode the cosmos, pushing the boundaries of what we know about the universe. For students and explorers of science, this framework is not merely a hurdle to overcome; it is an invitation to quantifying the universe’s most profound mysteries.
Key Predictions of General Relativity
The predictions of general relativity fundamentally reshape our conception of astronomical phenomena. They are not merely theoretical musings; they are testable claims about the way the universe operates. These predictions hold immense significance, driving advancements in a variety of fields. Understanding these concepts deepens our grasp of gravitational interactions, providing a lens through which we observe cosmic events that might otherwise seem detached from our earthly experiences.
Gravitational Time Dilation
One of the most intriguing outcomes of general relativity is gravitational time dilation. In simple terms, time is not experienced uniformly across different gravitational fields. An observer in a strong gravitational field, say near a massive object like a black hole, will perceive time passing more slowly compared to an observer in a weaker field, such as far away in space.
This prediction has practical implications. For instance, satellites in orbit around Earth, such as those providing GPS services, have to account for time differences due to both their speed and the gravitational influence of Earth. Without accounting for gravitational time dilation, these systems would be off by several kilometers if uncorrected, leading to inaccuracies in navigation.
Then one could imagine how time behaves differently in various conditions, almost like a rubber band stretched across the universe.
Light Bending around Massive Objects
Another striking illustration of general relativity's predictions is the bending of light around massive objects. Einstein posited that gravity does not merely pull objects towards each other but warps the fabric of spacetime itself. Essentially, mass distorts spacetime, and light, which typically travels in straight lines, follows this curvature.
This phenomenon has been observed directly. For example, during a solar eclipse in 1919, astronomers noted that starlight passing near the Sun was deflected. Such observations corroborated Einstein’s theory and showcased how massive celestial bodies could alter the paths of light from distant stars. Just imagine the spectacle of light dancing around a giant cosmic organism!
Gravitational Waves
Perhaps one of the most groundbreaking confirmations of general relativity came with the detection of gravitational waves. These ripples in spacetime are generated by some of the universe’s most violent and energetic processes, like colliding black holes or neutron stars spiraling into each other. In 2015, the LIGO observatory made history by observing these waves, marking an unprecedented moment in physics.
Gravitational waves carry clues about their cataclysmic origins and the very nature of gravity itself. They are a direct manifestation of the dynamic nature of spacetime, as described by Einstein's equations. Imagine a stone thrown into a pond causing ripples; gravitational waves travel through the universe, but instead of water, they disturb the very fabric of spacetime, allowing researchers a new way to observe cosmic phenomena that were otherwise invisible.
As we delve into these key predictions, we not only appreciate the profound implications of Einstein's theory but also open the door to understanding the intricate tapestry of our universe.
"General relativity elegantly illustrates that time, space, and gravity are entwined, shaping the cosmic dance of the cosmos."
Through these predictions, general relativity continues to inspire inquiry and innovation, compelling each generation to explore further the depths of what is known and what remains to be discovered.
Experimental Evidence Supporting General Relativity
The phrase "theory in science" often raises eyebrows, mostly because the word theory evokes images of guesswork. Nevertheless, in the realm of physics, particularly with General Relativity, evidence is king. Its reliance on experimental validation is fundamental, establishing the theory not just as a mere hypothesis but as a cornerstone of our understanding of the universe.
Why is Experimental Evidence Crucial?
Understanding General Relativity through empirical evidence allows us to comprehend its societal and scientific ramifications. The theory is not just an abstract concept; it has real-world applications that change how technology like GPS systems function. Furthermore, it offers insights into the workings of the universe from black holes to the expansion of galaxies.
Early Tests and Observations
In the early years following Einstein’s formulation, several observations were undertaken to assess the validity of General Relativity. Though some experiments were limited in their scope, they were groundbreaking nonetheless. One of the first significant tests was conducted during a solar eclipse in 1919.
- Solar Eclipse of 1919:
During this event, Sir Arthur Eddington verified Einstein's prediction that light from distant stars would bend around the sun due to gravitational field. Photographic plates taken before and after the eclipse illustrated this curvature, lending credence to Einstein's ideas. - Mercury's Perihelion Precession:
Another early observation involved the orbit of Mercury. The planet's elliptical path around the Sun presented an anomaly that classical Newtonian mechanics could not fully explain. General Relativity, however, corrected this discrepancy, accurately predicting the tiny shift in Mercury's position.
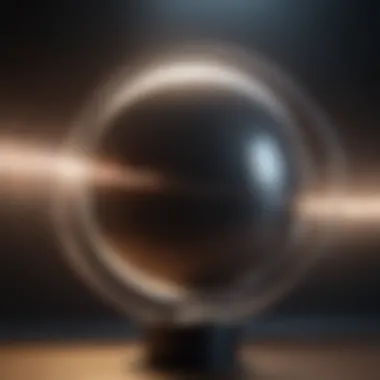
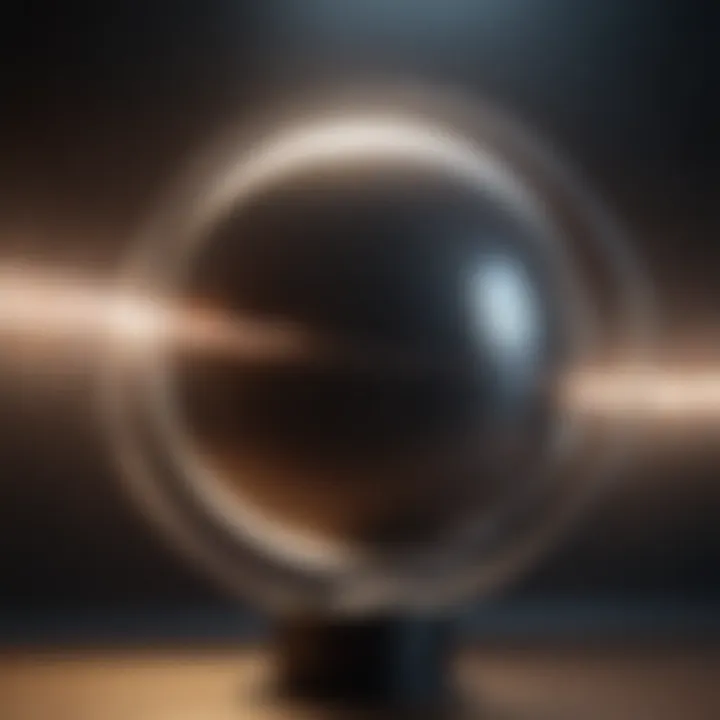
These investigations not only fostered widespread interest but eventually became definitive proof that Einstein's theory held merit.
Modern Validation through Astrophysics
Fast forward to today, and the landscape of astrophysics has changed dramatically. Advanced technology has provided researchers with the tools necessary to conduct precise measurements and observations that were previously inconceivable.
- Gravitational Waves:
One of the milestone achievements of modern astrophysics is the detection of gravitational waves by the LIGO (Laser Interferometer Gravitational-Wave Observatory) in 2015. These ripples in spacetime were directly predicted by General Relativity and confirmed its validity in a completely new realm. - Observations of Supermassive Black Holes:
In studying the centers of galaxies, scientists have gathered robust evidence supporting the existence of supermassive black holes through the motions of surrounding stars. The behavior of these stars conforms to the equations of General Relativity, offering yet another reassuring nod to Einstein’s framework.
"Experimental evidence does not merely affirm a theory; it frames the cornerstone on which scientific discourse rests."
Applications of General Relativity
General relativity is much more than just a theoretical framework; it has profound implications and practical applications across various fields. This section aims to underscore the significance of this theory in modern advancements and technology, emphasizing how its principles can be seen operating in our daily lives and pushing the boundaries of human understanding.
Navigating Satellite Technology
One of the most tangible benefits of general relativity can be observed in satellite technology, particularly through the Global Positioning System (GPS). GPS satellites orbit the Earth at high speeds and altitudes, providing precise location data that is essential for navigation.
However, to ensure accuracy, these satellites must account for several relativistic effects:
- Gravitational Time Dilation: Satellites experience less gravitational pull than objects on Earth, causing their onboard clocks to tick faster than clocks on the ground.
- Special Relativity Effects: Due to their speed, satellite clocks also experience time dilation because of their velocity, causing them to run slightly slower.
If these relativistic effects were not corrected, GPS navigation would drift by about ten kilometers each day, rendering it useless. Hence, the successful function of GPS technology is a direct application of general relativity, demonstrating how Einstein’s ideas have become crucial for modern society.
Understanding Black Holes
Black holes represent one of the most fascinating predictions of general relativity. These regions in spacetime have such strong gravitational forces that not even light can escape them. The study of black holes not only enhances our understanding of gravity but also addresses fundamental questions about the universe's structure and destiny.
Through astrophysical observations:
- Event Horizon: This boundary around a black hole marks the point of no return. Understanding the event horizon has led to breakthroughs in theoretical physics.
- Hawking Radiation: Proposed by Stephen Hawking, this concept suggests that black holes can emit radiation, providing a bridge between quantum mechanics and general relativity.
Researching black holes fuels numerous theoretical inquiries, stimulating advancements in cosmology and physics. The recent imaging of a black hole by the Event Horizon Telescope represents a significant leap forward, giving us concrete visual evidence supporting Einstein's theories.
Cosmology and the Large-Scale Structure of the Universe
General relativity also underpins our understanding of cosmology—the study of the universe's origin, evolution, and ultimate fate. The theory provides the framework to comprehend the cosmos as a dynamic and expanding entity.
Key concepts influenced by general relativity in cosmology include:
- Big Bang Theory: It describes the universe's initial condition and expansion. General relativity helps model this expansion and predict the cosmic microwave background radiation.
- Dark Energy and Dark Matter: These mysterious components of the universe are inferred from the gravitational effects seen on large scales. Understanding how general relativity applies to their behavior is pivotal in modern astrophysics.
Furthermore, gravitational lensing, a phenomenon predicted by general relativity, enables astronomers to study distant galaxies by observing how massive objects warp the light from those galaxies. Such techniques have opened avenues for exploring the universe's vastness and complexities.
"General relativity isn't just a pillar of physics; it’s the key to understanding the cosmos."
The applications of general relativity extend across various disciplines, unlocking mysteries of the universe and driving innovations that permeate our daily lives. By applying its principles, science thrives in areas that were once thought to be unattainable, leaving a lasting imprint on our view of reality.
Philosophical Implications of General Relativity
General relativity does more than merely describe the laws of physics; it extends into the realm of philosophy, inviting us to reconsider our perceptions of space, time, and the universe at large. This theory has provoked a deep reevaluation of foundational concepts that have dominated human thought for centuries. Its implications challenge us to rethink our place within the cosmos and how we interpret the nature of reality. This section explores two pivotal areas: the redefinition of space and time and the overall nature of reality, both reshaped profoundly by Einstein's groundbreaking work.
Redefining Space and Time
Space and time, once viewed as mere backdrops to human activity and celestial movements, have taken on a newfound significance through general relativity. Einstein's insight that space and time are not separate entities but rather interwoven aspects of the same fabric—spacetime—has major repercussions. This marriage of space and time implies that what we perceive to be static and absolute is, in fact, dynamic and flexible.
Consider this: as objects with mass warp spacetime, they not only alter the paths of other objects but also affect time itself. For example, a clock positioned closer to a massive object will tick slower compared to one farther away. This time dilation isn't just a theoretical construct; it's observable in practical applications, like in GPS technology where corrections must be made to account for the differences in time experienced by satellites in orbit compared to people on Earth.
- Key elements of this redefinition:
Spacetime is a unified construct.
Gravity is understood as curvature rather than a force.
Time is influenced by speed and mass.
This new understanding of space and time leads to profound philosophical questions. If our perception of the universe hinges so much on the gravitational influences around us, do we even have an objective view of reality? What constitutes the passage of time when it can alter depending on our position in relation to mass?
"The distinction between past, present, and future is only a stubbornly persistent illusion." - Albert Einstein
The Nature of Reality
The ramifications of general relativity extend beyond mere scientific calculations, delving deep into the philosophical domain of existence itself. If spacetime can bend and flow, where does that leave the concept of objective reality? In essence, it suggests a universe that is contingent on the observer's frame of reference. This challenges the long-held notion of an independent reality waiting to be discovered. Instead, reality itself may be an intricate tapestry, woven from the experiences and gravitational influences that shape our understanding.
Engaging with these ideas often leads to ponderings about determinism and free will. If spacetime influences our perceptions and the unfolding of events in our lives, can we argue that we have autonomy over our choices? Or are we simply following a path dictated by the gravitational pull of prior events—a cosmic script?
Moreover, the implications of black holes, where spacetime curvature becomes extreme, challenge traditional notions of knowledge and understanding. What exists beyond the event horizon remains speculative, invoking philosophical inquiries about the limits of human comprehension and the nature of infinity.
- Aspects to consider:
Reality may be observer-dependent.
Determinism vs. free will in a relativistic framework.
Mysteries surrounding black holes and what they entail about knowledge and existence.
As we navigate the intricacies of general relativity, we should prepare ourselves to confront these existential dilemmas. Ultimately, the interplay of science and philosophy in this realm does much more than clarify our understanding of the universe; it compels us to reflect on our existence within it.
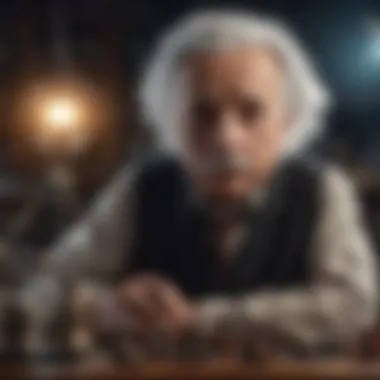
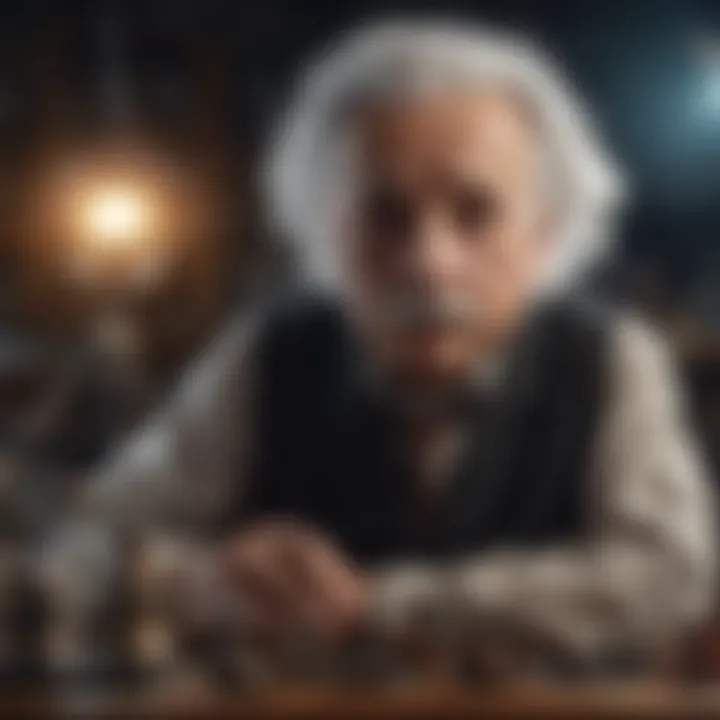
Challenges and Considerations in General Relativity
Understanding the challenges and considerations that stem from general relativity is vital for a comprehensive grasp of modern physics. This section will delve into how the theoretical frameworks of general relativity and quantum mechanics often clash and the implications of singularities. The investigation of these topics unearths not only the limits of our understanding of gravity but also poses questions about the very fabric of reality itself.
Incompatibility with Quantum Mechanics
One of the most prominent hurdles that physicists face is the apparent incompatibility between general relativity and quantum mechanics. General relativity describes gravity as the curvature of spacetime, while quantum mechanics deals with the physics of the very small, like subatomic particles. The two frameworks operate under different principles. For example, quantum mechanics successfully predicts phenomena at microscopic scales, whereas general relativity shines a light on the large-scale structure of the universe.
- Different Realms of Focus
- Mathematical Frameworks
- General relativity explains gravity and its effects on galactic and cosmic scales.
- Quantum mechanics focuses on the interactions of particles at very small scales.
- The equations governing quantum mechanics (like the Schrödinger equation) differ vastly from Einstein's field equations.
- In general relativity, spacetime is depicted with smooth geometrical shapes, while quantum mechanics often portrays particles as point-like.
To put it simply, when scientists attempt to integrate laws of quantum mechanics within the framework of general relativity, the result is often chaotic and counterintuitive. The search for a unified theory that encapsulates both realms is ongoing, with contenders like string theory and loop quantum gravity leading the charge.
"The conflict between quantum mechanics and general relativity is one of the greatest unsolved problems in physics today."
Singularities and Their Controversies
Another intriguing aspect of general relativity is the presence of singularities. These are points in spacetime where the gravitational forces compress matter to an infinite density, such as at the center of black holes. Singularities serve as both a fascinating mystery and a point of contention among physicists. The problems they introduce raise questions about the limits of our understanding.
- Mathematical Paradoxes
- Physical Implications
- At singularities, the mathematical models break down, leading to solutions that are often considered nonsensical (e.g., dividing by zero).
- They challenge the expectation of continuity and predictability within physics.
- Singularities suggest regions where the laws of physics cease to function as we know them.
- They prompt discussions on concepts like "the Big Bang," which is thought to have originated from a singularity—hence, theorizing about the birth of the universe itself and what came before it serves as fertile ground for debate.
Addressing the controversies surrounding singularities calls for reevaluation of fundamental principles of physics. These discussions often lead to innovative ideas and could ultimately result in breakthroughs that bridge the gap between relativity and quantum physics.
Future Directions in Research
The landscape of physics is ever-changing, continuously evolving as we push the limits of our understanding. In the realm of general relativity, the future beckons with exciting prospects that promise to deepen our insight into the universe. The significance of delving into future directions in general relativity lies not only in the theoretical advancements but also in practical applications that could transform technology and enhance our comprehension of cosmic phenomena. These developments hold the potential to bridge gaps in current knowledge and tackle long-standing challenges.
Next-Generation Gravitational Wave Detectors
With the discovery of gravitational waves by the LIGO observatory in 2015, a new chapter opened in astrophysics. This year marked an awakening in our ability to perceive the universe's most violent events—such as black hole mergers and neutron star collisions—in ways that were previously only dreams of scientists. However, to further enhance our perception, next-generation gravitational wave detectors are being envisioned and developed.
These advanced instruments aim to enhance sensitivity and increase the detection range. For instance, the proposed Einstein Telescope and the Cosmic Explorer are poised to detect fainter signals from more distant astronomical events. These detectors will utilize novel technologies based on cryogenic sensors and optical techniques that can amplify the faintest ripples in spacetime.
The impact of these advancements is profound. By gathering more data, researchers can refine their models of gravitational waves, leading to a better understanding of the universe's evolution. Importantly, such technology could also contribute to finding signals of new physics beyond general relativity, providing insights into the fabric of reality itself.
The Quest for a Unified Theory
As we gaze toward the horizon, the desire for a unified theory of physics remains a relentless pursuit. For years, physicists have grappled with integrating general relativity with quantum mechanics, a marriage that seems so elusive yet tantalizingly within reach. The quest for such unification is not merely academic; it has the potential to resolve discrepancies between the small-scale and large-scale behaviors of the universe.
One promising avenue involves string theory, which posits that fundamental particles are one-dimensional objects, or strings, rather than point-like entities. This perspective could harmonize the differences in fundamental physical laws, aligning gravity with the other three forces—electromagnetism, weak force, and strong force. Moreover, loop quantum gravity offers an alternative framework, aiming to describe gravity by directly incorporating the principles of quantum mechanics.
By resolving long-standing paradoxes such as the nature of black holes and the behavior of singularities, the quest for a unified theory could redefine our understanding of reality. Additionally, a unified approach may lead to groundbreaking technologies, enhancing our capability to manipulate the forces of nature on unprecedented scales.
"The future belongs to those who believe in the beauty of their dreams."
Eleanor Roosevelt
In summary, the journey ahead in the field of general relativity is rich with possibilities. As researchers develop next-generation gravitational wave detectors and strive for a unifying framework for physics, the implications for science and technology are immense. The pursuit of these goals not only enhances our understanding of the cosmos but also inspires future generations of scientists to look beyond the stars and question the very nature of existence.
Epilogue: The Lasting Impact of General Relativity
The consequences of general relativity stretch far beyond its initial unveiling in the early 20th century. This theory is not just a set of equations describing gravity; it stands as a monumental shift in the way we understand the universe. It has redefined all aspects of physics, laying the groundwork for further explorations into the nature of reality, the fabric of spacetime, and the fundamental forces at play in our galactic surroundings.
One of the most significant aspects of this theory is its incredible predictive power. From black holes to the expanding universe, general relativity has provided the framework for deciphering phenomena that were once cloaked in mystery. Without this lens, our grasp of the cosmos would remain dim.
For instance, the gravitational waves predicted by Einstein have not only been detected, confirming his work but also opening a new field of astrophysics. Such discoveries signal the advent of a new era in which scientists can observe cosmic events in ways that were previously unimaginable. Every discovery paints a clearer picture of our universe, culminating in a legacy that intertwines with contemporary scientific inquiry.
"General relativity is the most influential theory of the 20th century, touching all domains of modern physics and even beyond, from GPS systems to the understanding of cosmological phenomena."
Legacy in Science and Beyond
The legacy of general relativity is felt across multiple disciplines, transcending its origins in theoretical physics. Fields like astrophysics, cosmology, and even technology utilize its principles.
- Astrophysics: The theory has driven the investigation of black holes, neutron stars, and cosmic background radiation, explaining how these entities interact in space.
- Cosmology: It lays the basis for the Big Bang theory, enhancing the comprehension of the universe's origin and its ongoing expansion.
- Technology: We rely on general relativity for accurate GPS navigation. Satellite signals must account for time dilation; otherwise, navigating our world would be akin to sailing without a compass.
In education, the theory challenges students to rethink their conceptions of space and time, fostering critical thinking and inquiry-based learning.
Encouraging Future Inquiry
Encouraging future inquiry in general relativity is essential, as the world of physics remains fraught with unanswered questions. As we delve deeper, we must ultimately confront the compatibility of this esteemed theory with quantum mechanics, which remains one of the pivotal puzzles of modern science.
The quest for a unified theory that coherently merges general relativity with quantum principles is in full swing. Innovative research in gravitational wave astronomy may generate unprecedented insights into the heart of black holes or the very fabric of spacetime itself.
Researchers should also engage with the general public. Initiatives aimed at demystifying physics can inspire the next generation of scientists. Bridging the gap between complex theories and public understanding can foster a culture of curiosity and inquiry.
As the universe unfolds its secrets through the lens of general relativity, the importance of continual exploration, questioning, and validation becomes paramount. The journey of understanding is never truly finished; every answer leads to new questions, keeping the flame of intellect alive as we progress through the cosmos.