Understanding Gamma Rays: Their Origins and Impact
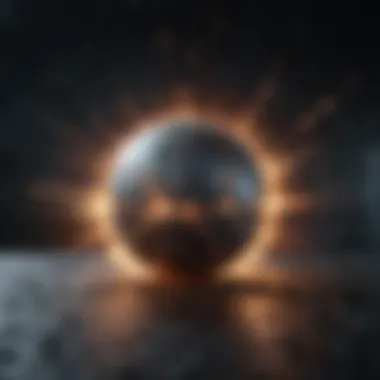
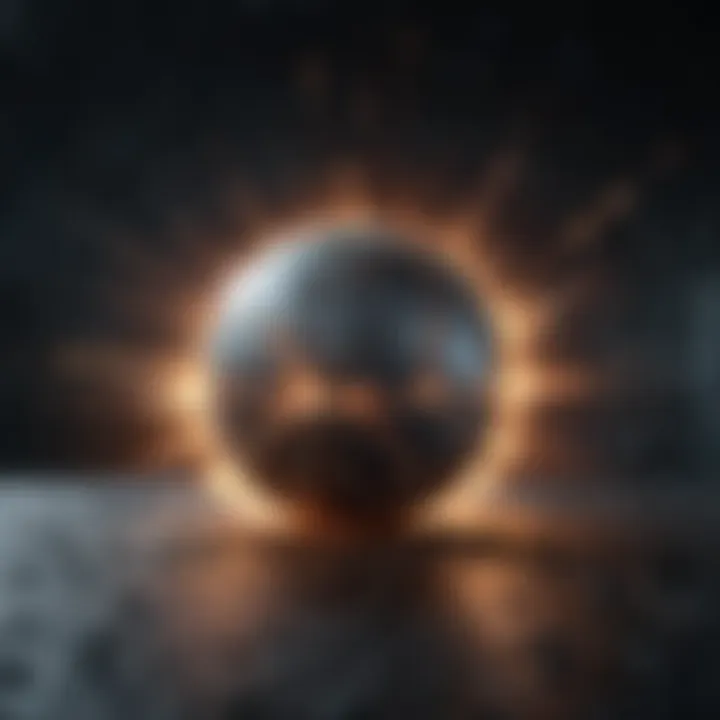
Intro
Gamma rays hold a special place in the spectrum of electromagnetic radiation. Known for their extremely high energy and unique properties, these rays originate from some of the universe's most violent phenomena, such as supernova explosions, neutron star collisions, and even radioactive decay.
In recent years, the scientific community has made substantial strides in understanding gamma rays, paving the way for exciting applications that extend from medical technology to astrophysics. The challenges and breakthroughs in detecting and analyzing these rays are not just academic pursuits; they shape practical solutions that echo through various fields including oncology, astronomy, and nuclear science. Under this lens, it becomes evident that appreciating gamma rays' intricacies requires a blend of observation, analysis, and innovative technology.
Recognizing the importance of gamma rays opens up dialogues around their origins, their role in scientific advancements, and their daily significance. And frankly, the underlying physics can get quite engaging once you peel back the layers of complexity.
Key Research Findings
Overview of Recent Discoveries
Within the past few decades, understanding of gamma rays has evolved in leaps and bounds. Researchers have utilized space-based observatories like the Fermi Gamma-ray Space Telescope, which has revealed unprecedented details about gamma-ray sources like pulsars and blazars. Key findings include:
- Cosmic Ray Interactions: Studies indicate that gamma rays often arise from high-energy cosmic ray interactions with interstellar matter. This insight not only informs astrophysics but also sheds light on potential hazards in space exploration.
- Dark Matter Signals: Some studies suggest that gamma rays might help trace the elusive nature of dark matter. The hypothetical particles known as WIMPs could potentially produce detectable gamma rays upon annihilation.
As these discoveries unfold, they bring us closer to resolving old questions while also illuminating new pathways for exploration and understanding.
Significance of Findings in the Field
The significance of these breakthroughs cannot be understated. For physicists and astronomers, these discoveries add significant layers to our comprehension of the universe. In medical science, the application of gamma radiation in imaging and cancer treatment demonstrates its practical value. It helps not just scientists but also shallow the gap between theoretical physics and practical use in hospitals around the world.
"Gamma rays might seem abstract and distant, yet they underscore much of the technology and insights we enjoy today."
Breakdown of Complex Concepts
Simplification of Advanced Theories
Understanding the mechanisms behind gamma rays can feel like grasping at shadows, but some fundamental concepts can clarify this. Gamma rays are a byproduct of nuclear reactions and decay; think of them as the by-product of energetic processes that happen beyond what we can typically see.
A simple way to break it down:
- Origin: Most often, they come from unstable atomic nuclei.
- Properties: Gamma rays travel at light speed and can penetrate materials, which makes them incredibly useful in a medical setting but also severe when it comes to radiation exposure.
Visual Aids and Infographics
To help visualize the complexity of gamma rays, infographics depicting their formation processes, energy levels, and applications can be invaluable. Simple charts that correlate the various sources of gamma rays alongside their applications in medicine and research could substantiate these ideas further.
Through this exploration of gamma rays, we not only appreciate their fundamental nature but also their diverse applications, pushing the boundaries of what we know and what we can achieve in science.
Prolusion to Gamma Rays
Gamma rays are an essential aspect of modern physics and astronomy, playing pivotal roles that reverberate through various fields like medicine, nuclear physics, and even astrophysics. Understanding these rays not only shines a light on the underpinnings of the universe but also reveals the intricate connections between fundamental science and its practical applications. Given their high energy and unique properties, gamma rays act as both a tool and a subject of study, giving researchers insights into processes ranging from the nuclear reactions occurring in the cores of stars to the mechanisms of diseases in humans.
Definition and Basic Properties
Gamma rays are a form of electromagnetic radiation, similar to visible light but with much higher energy levels. Here are some defining characteristics:
- Wavelength and Frequency: They possess very short wavelengths, typically less than 0.01 nanometers, which corresponds to extremely high frequencies, often exceeding 10^19 Hz. This remarkable energy enables gamma rays to penetrate matter more effectively than other forms of radiation, like X-rays.
- Ionization Ability: Gamma rays can ionize atoms, meaning they can knock electrons off atoms, creating charged particles. This property makes them useful in various applications, such as radiation therapy in cancer treatment, though it also imposes risks of radiation exposure.
- No Charge or Mass: Being neutral and massless particles, gamma rays travel at the speed of light. They do not interact strongly with matter, which contributes to their penetrating power yet requires sophisticated detection methods that can capture their elusive nature.
Distinction from Other Types of Radiation
Gamma rays are often compared to other radiation types, such as alpha and beta radiation, but several key distinctions set them apart:
- Composition: Alpha particles, made of two protons and two neutrons, and beta particles, which are electrons or positrons, differ fundamentally from gamma rays, which are pure electromagnetic energy with no particles involved.
- Penetration Ability: While alpha particles can barely penetrate human skin, and beta particles can penetrate slightly deeper, gamma rays can traverse thick materials, needing dense shielding, like lead or several centimeters of concrete, to be effectively stopped.
- Origin: Gamma rays generally arise from nuclear reactions or radioactive decay, while alpha and beta particles come from different types of decay processes. For instance, the emission of gamma rays often accompanies beta decay, but it can also occur independently, suggesting a fascinating relationship within nuclear physics.
"Gamma rays serve as a bridge between the macroscopic and microscopic worlds, revealing the hidden dynamics of atomic interactions while enabling revolutionary breakthroughs across disciplines."
Origins of Gamma Rays
Understanding the origins of gamma rays is crucial for appreciating their role in the broader context of physics and our universe. It's not just about identifying where these rays come from; it’s about recognizing the intricate mechanisms and dynamic processes that give rise to them. This article will explore the specific sources of gamma rays, shedding light on their cosmic and terrestrial origins. The study of these origins provides insights into cosmic events and the behavior of matter under extreme conditions.
Cosmic Sources
Gamma rays are birthed in some of the most violent and energetic environments known to mankind. One of their most prominent sources is cosmic events such as supernovae and the collisions of high-energy particles in the interstellar medium. Supernovae, the cataclysmic explosions of dying stars, are responsible for an intense outpouring of gamma radiation. During these events, the core collapses, and consequently, enormous energies are released.
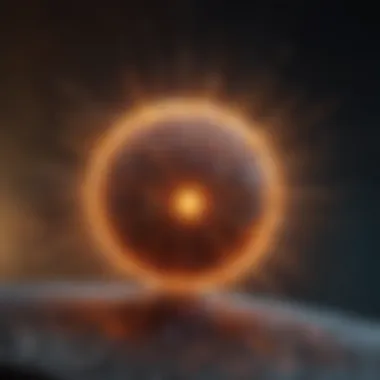
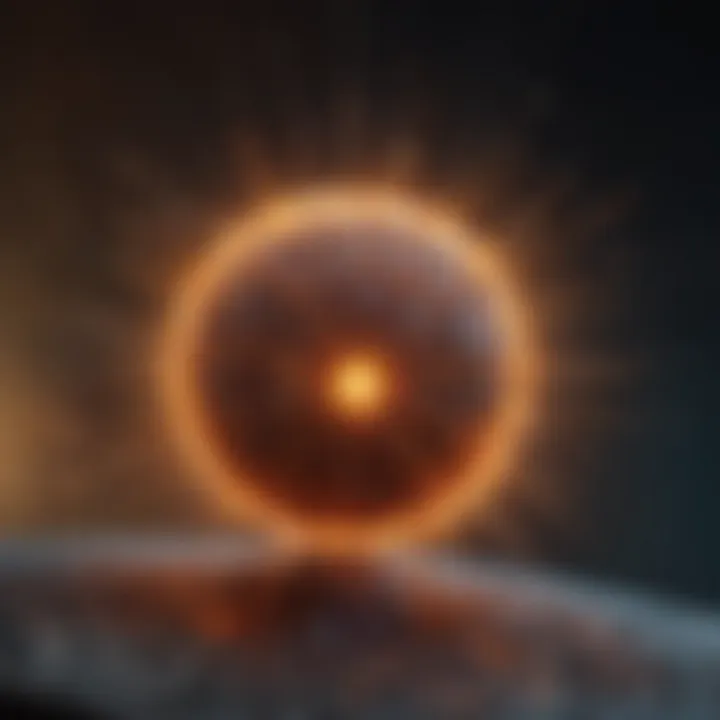
Another incredible source is the phenomenon known as gamma-ray bursts, which are brief but extraordinarily powerful outbursts of gamma radiation. These bursts can occur when massive stars collapse into black holes or during the merger of neutron stars. It's believed that these events can release more energy in a few seconds than our sun will emit over its entire lifetime.
- Distinct Characteristics of Cosmic Gamma Rays:
- Originating from extremely energetic cosmic events.
- Reflect the conditions present in the universe shortly after the Big Bang.
- Informs us about the structure and evolution of galaxies.
The study of these cosmic sources not only enriches our comprehension of gamma rays but also enhances our understanding of astrophysical phenomena, influencing theories about the universe's origins and its ongoing evolution.
Nuclear Reactions
Nuclear reactions play a pivotal role in the creation of gamma rays. This essence becomes clear when one considers the processes within atomic nuclei, where tremendous forces govern their behavior. Fusion reactions, such as those that occur in the cores of stars, are significant contributors. During fusion, lighter nuclei combine to form heavier ones, often releasing a gamma photon as a byproduct. The implications of this are profound, as fusion is the same process that fuels our sun and sustains life on Earth.
On the other hand, fission reactions, which are often harnessed in nuclear reactors, also generate gamma rays. When heavy atomic nuclei split apart, a torrent of energy is released, accompanied by the emission of gamma radiation. These reactions highlight the dual nature of gamma rays—they are simultaneously natural phenomena and byproducts of human technological endeavors.
- Key Insights from Nuclear Reactions:
- Showcases the fundamental forces at play in atomic interactions.
- Raises considerations for energy production and safety within nuclear technology.
- Reveals pathways toward understanding elemental synthesis in the universe.
Grappling with these different aspects of nuclear reactions allows researchers to refine models of nuclear behavior, deepening our knowledge potent interactions of matter.
Beta Decay and Gamma Emission
Beta decay is another critical phenomenon associated with gamma-ray production. This process involves the transformation of a neutron into a proton or vice versa, accompanied by the emission of a beta particle, which can be either a positive or negative charge. As a byproduct, the nucleus often emits gamma radiation to shed excess energy in a process known as gamma emission.
This relationship between beta decay and gamma emission is essential for understanding how unstable isotopes interact in nature. Many radioactive isotopes undergo beta decay, resulting in gamma-ray emissions that researchers can detect using sensitive instruments. This natural occurrence is not limited to laboratories; it occurs within the Earth’s crust, leading to contributions to the natural background radiation.
The interactions of beta decay and gamma emission give rise to a wealth of knowledge about atomic structure and stability, highlighting the delicate balance of forces within the nucleus.
- Significance of Beta Decay and Gamma Emission:
- Provides insight into the stability of atomic nuclei.
- Aids in the creation of various radionuclides used in medical diagnostics and treatments.
- Contributes to the understanding of radiation hazards and safety measures in environments where radioactive materials exist.
In essence, the origins of gamma rays, traced through cosmic events, nuclear reactions, and beta decay, offer a complex, yet fascinating narrative. It intertwines cosmic scale phenomena with human curiosity and technological advancement, paving the way for advances in both scientific understanding and practical applications.
Detection and Measurement Techniques
Detection and measurement techniques for gamma rays are paramount in the study of these high-energy photons. Since gamma rays are both elusive and highly penetrating, having the right methods to detect and analyze them is crucial for understanding their origins and effects. This section discusses various techniques employed, highlighting their importance, benefits, and practical considerations.
Scintillation Detectors
Scintillation detectors sit at the forefront of gamma ray detection technology. They function by utilizing scintillating materials that emit light when interacting with gamma rays. This light is then converted into an electrical signal, allowing researchers to quantify the gamma radiation. Common scintillation materials, such as sodium iodide or cesium iodide, play a pivotal role in these detectors.
These detectors have several advantages: they offer quick response times and can be relatively compact, making them suitable for both laboratory and field use. However, they do require careful calibration and a proper understanding of their limitations, such as energy resolution and sensitivity to background radiation.
Semiconductor Detectors
When it comes to precision in gamma ray measurement, semiconductor detectors are hard to beat. Typically made of materials like germanium or silicon, these detectors capitalize on the principle that gamma rays can create electron-hole pairs in the semiconductor. By measuring these pairs, one can obtain detailed information about the energy and intensity of the incoming radiation.
The benefits of semiconductor detectors include excellent energy resolution and the ability to distinguish between different types of gamma emissions. They can provide real-time monitoring, which is particularly useful in environments where radiation levels can fluctuate rapidly. On the downside, they tend to be more delicate and require cryogenic cooling in the case of germanium detectors, which may limit their practicality in some scenarios.
Photographic Plates and Film
Though somewhat old-fashioned compared to modern digital methods, photographic plates and film have a unique place in gamma ray detection. This technique uses the principle of exposing a sensitive emulsion to gamma radiation, which then creates a latent image. When developed, the film reveals the areas where gamma rays interacted, providing a visual representation of radiation distribution.
While less common today, these methods are still relevant in certain applications, particularly in experimental setups where digital technology may not always fit. One key upside is their historical significance; many early gamma ray studies relied on photographic techniques, paving the way for today’s advancements. Nevertheless, they typically lack the efficiency and sensitivity of newer technologies.
In summary, detection techniques for gamma rays are critical in unraveling their complexities. Each advancement, whether scintillation detectors, semiconductor systems, or traditional photographic methods, brings us closer to understanding gamma radiation's vast implications across various fields.
Gamma Rays in Astronomy
Gamma rays play a pivotal role in the field of astronomy, serving as a window to some of the universe's most energetic and violent processes. These high-energy photons, with wavelengths shorter than X-rays, provide insights into cosmic phenomena that would otherwise remain hidden from our observational capabilities. By capturing gamma rays, astronomers can unveil aspects of the cosmos, leading to comprehension of matters like black holes, supernovae, and even the mysterious dark matter that seems to pervade our universe.
Gamma Ray Bursts
Gamma ray bursts (GRBs) are perhaps one of the most intriguing celestial events. These are immense explosions that occur in distant galaxies, believed to signal the birth of black holes or the collapse of massive stars. When a GRB happens, it emits a fascinating amount of energy in the form of gamma radiation, sometimes briefly outshining entire galaxies.
The detection of GRBs relies heavily on sophisticated satellite technology, such as NASA's Swift Observatory. These smaller spacecraft are designed to quickly locate and analyze gamma-ray emissions. The importance of GRBs lies not just in their immense energy, but also in their potential to help us understand the early universe. For instance, studying the afterglow of GRBs can shed light on the composition and evolution of stars and galaxies over billions of years, effectively acting as cosmic lighthouses across the vastness of space.
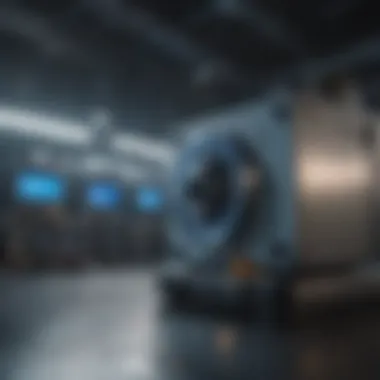
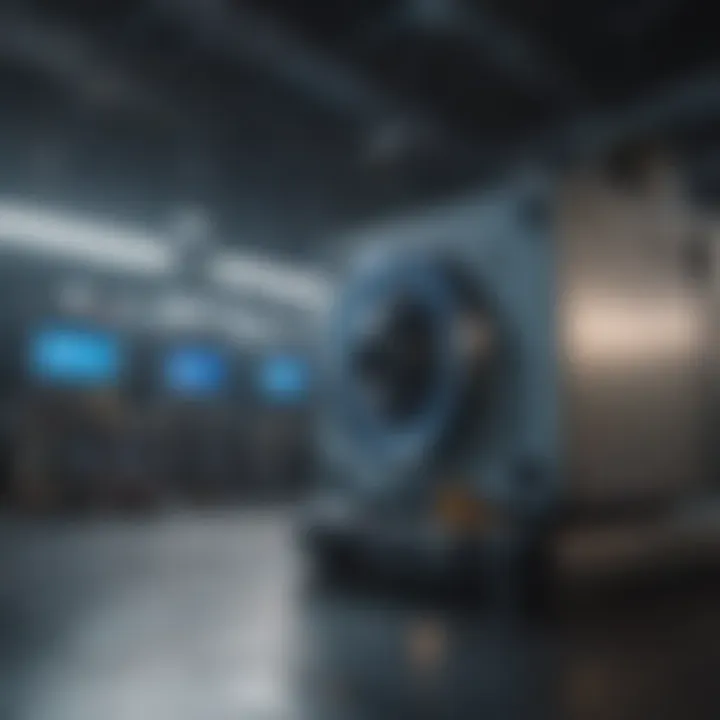
Moreover, the research on GRBs can give insights into the conditions leading to cosmic events, enhancing our grasp of special relativity and the dynamics of massive stellar evolution.
Active Galactic Nuclei
Another captivating application of gamma rays in astronomy is the study of active galactic nuclei (AGN). These regions at the center of some galaxies host supermassive black holes surrounded by swirling, hot gas. The gravitational forces at play can generate vast amounts of energy, resulting in jets of particles being ejected at nearly the speed of light, which emit gamma rays detectable from Earth.
Understanding AGN is essential, as they represent a significant portion of the universe’s energy output. Through the detection of gamma rays emanating from these active regions, astronomers can explore the mechanisms of energy production and the growth of black holes over cosmic time. Some prominent examples include quasars, which are extremely luminous AGN, serving as indicators of how galaxies evolve and form over billions of years.
By utilizing gamma-ray observations, researchers can delve into the processes behind jet formation, particle acceleration, and ultimately the role black holes play in galaxy formation and evolution.
Applications in Medicine
In the theater of modern healthcare, gamma rays play a star role. Their ability to penetrate tissues allows them to be utilized for both therapeutic and diagnostic purposes. The significance of gamma rays in medicine is not merely academic; it holds the potential to directly influence patient outcomes and improve healthcare practices.
Radiation Therapy
One noteworthy application of gamma rays lies in radiation therapy for cancer treatment. This method leverages high-energy gamma rays to target and obliterate malignant cells. When it comes to treating tumors, precision is paramount. Gamma rays can be focused tightly on the cancerous area, resulting in minimized damage to surrounding healthy tissues.
The process typically involves a device known as a gamma knife, which is a form of stereotactic radiosurgery. This advanced equipment can deliver doses of radiation with pinpoint accuracy. Many patients find it less intrusive than traditional surgical methods, leading to shorter recovery times.
Studies have shown that combining gamma radiation with other treatment modalities can enhance efficacy. For example, when used in conjunction with chemotherapy, the combination can yield better results than either approach alone. Even though it is effective, there are significant considerations: side effects can include fatigue, skin irritation, and changes in appetite, which clinicians must closely monitor.
Diagnostic Imaging
Turning our focus to diagnostic imaging, gamma rays also offer incredible value in medical imaging, particularly through a technique called gamma camera imaging. This method enables physicians to visualize internal organs and systems in real-time.
In practice, a patient is given a radioisotope that emits gamma rays. This isotope is usually administered via injection or ingestion, and it accumulates in specific organs depending on its chemical properties. The gamma camera detects these rays, generating detailed images that assist in diagnosing conditions ranging from heart disease to cancers.
Gamma imaging has several advantages:
- Detailed Diagnosis: It provides images that can highlight abnormalities that other imaging methods may not detect.
- Functional Perspective: Unlike X-rays that show structural details, gamma ray imaging provides insights into how organs function.
- Non-invasive Nature: Patients often appreciate that this method doesn't involve invasive procedures, making it less daunting.
However, care must be taken regarding radiation exposure. Medical practitioners follow strict guidelines to ensure that the benefits outweigh potential risks associated with repeated exposure to gamma radiation.
In summary, gamma rays are indispensable in the modern medical landscape, providing both therapy and diagnostic capabilities that are pivotal for effective patient management and treatment. As technology evolves, ongoing research could unveil even more ways to leverage this powerful tool.
Role in Nuclear Physics
Gamma rays play a significant role in the field of nuclear physics. Their behavior and interaction with matter are essential for comprehending various nuclear processes. Understanding gamma rays can greatly enhance our knowledge about nuclear reactions, radioactive decay, and even the stability of atomic nuclei. This area of study nurtures cross-disciplinary applications, including advancements in safety protocols, medical technologies, and fundamental research in particle physics. The importance of gamma rays in nuclear physics can’t be overstated, as they bridge various aspects of nuclear science.
Nuclear Reactions and Stability
In nuclear physics, the concept of nuclear reactions is paramount. These reactions often release or absorb energy, and gamma rays are frequently emitted during such processes. For instance, when heavy nuclei undergo fission, they break apart and emit gamma radiation as a byproduct of this disintegration. The stability of atomic nuclei is also influenced by the emission of gamma rays.
Gamma emissions can happen spontaneously or can be triggered by external factors. When a nucleus becomes excited, it seeks to return to a more stable state, often emitting a gamma photon in the process. This rapid release of energy is not just a byproduct; it carries vital data on the structural integrity and composition of the nucleus involved. Understanding these reactions enables researchers to develop models predicting how substances will behave under various conditions, which proves valuable in many applied fields.
A deeper understanding of gamma emissions is essential for achieving safer nuclear energy production and improving medical radiation applications.
Radionuclide Production
Radionuclides, unstable isotopes that emit radiation, are heavily influenced by gamma rays in their production processes. The generation of radionuclides is crucial for numerous applications, particularly in medicine, where they are employed in diagnostic imaging and treatments.
For instance, some radionuclides are created through the capture of neutrons in nuclear reactors, where gamma rays are emitted during the decay processes. Another classic example can be observed in the production of Technetium-99m, widely used in medical imaging. This radionuclide, born from Molybdenum-99 decay, emits gamma rays which allow clinicians to visualize the internal systems of the body non-invasively.
- Key considerations regarding radionuclide production include:
- The purity of the produced isotopes.
- The half-life, which determines how long the radionuclide remains effective for its application.
- Safety measures, especially regarding exposure to gamma radiation during production and usage.
The combination of gamma rays and radionuclide production not only advances technological practices but enriches our understanding of fundamental nuclear processes, laying a pathway for future discoveries.
Environmental Impact of Gamma Radiation
Understanding the environmental impact of gamma radiation is critical for evaluating both its natural occurrence and the consequences of human activities. Gamma rays, being a form of high-energy electromagnetic radiation, have the potential to affect ecosystems, human health, and even the geological structure over time. The information below outlines the pivotal elements regarding the environmental implications of gamma radiation.
Natural Background Radiation
Gamma radiation is a natural part of the background radiation that surrounds us. It originates from cosmic rays, terrestrial sources like uranium and thorium in rocks, and even from certain types of food we consume. This radiation levels vary based on geographical location and the natural composition of the environment.
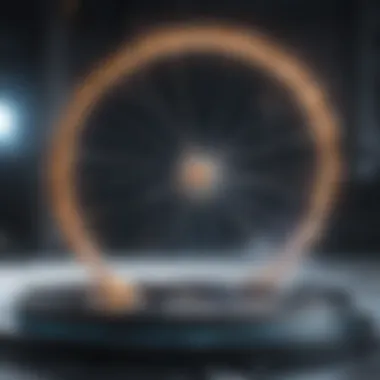
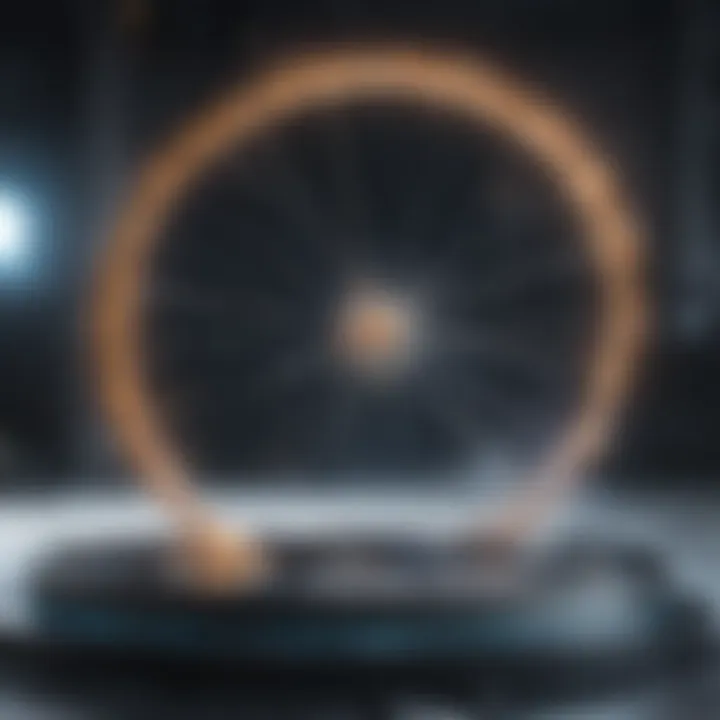
- Sources of Natural Background Radiation:
- Cosmic radiation from outer space, mostly at high altitudes.
- Radon gas, often found in homes, contributes significantly to exposure and comes from uranium decay in soil.
- Built environment factors, as various materials can emit low levels of gamma rays.
The normal environmental gamma radiation levels are generally considered safe for human health, but high concentrations can pose risks. It's essential to recognize that while some exposure is unavoidable, it is also vital to monitor these levels to protect population health and the environments people inhabit. The knowledge of everyday exposure helps in making informed choices about living spaces and potential mitigation strategies.
Effects of Human Activity
As mankind advances technologically, the interaction with gamma radiation has markedly escalated due to nuclear energy production, medical uses, and even military applications. The repercussions of these activities can be profound.
- Nuclear Power Plants: Despite the benefits of clean energy, the operation of nuclear power plants can result in the release of gamma radiation, particularly during accidents or malfunctions.
- Medical Applications: Procedures like radiation therapy for cancer treatments involve calculated doses of gamma rays, aimed at destroying malignant cells. While effective, they necessitate rigorous safety protocols to prevent unintended exposure to personnel and patient environments.
- Weapons Testing: Historically, nuclear weapons tests resulted in widespread, uncontrollable dispersal of gamma radiation in the environment. The long-term effects of such events have been significant, impacting flora, fauna, and human health.
"The environmental consequences of human activities related to gamma radiation are not merely transient; they can leave an indelible mark on both ecosystems and societal health."
In summary, while natural gamma radiation is mostly benign, human activities have the potential to amplify its harmful effects. Recognizing the sources and mitigating these impacts through thoughtful regulation and monitoring will be pivotal in ensuring a balanced coexistence with this potent form of radiation. Understanding both the natural background and the anthropogenic influences aids not only in safety but also in the sustainable development of technologies using gamma rays.
Safety and Health Considerations
Understanding safety and health considerations when dealing with gamma rays is paramount. Many might think of radiation solely through the lens of its scientific significance, yet it’s the implications for human health and safety that can’t be brushed aside. A host of factors exist regarding exposure, nuclear practices, and medical applications; adherence to safety measures is crucial for minimizing risks associated with gamma radiation.
Gamma rays, due to their high energy and penetrating ability, pose a distinct challenge in both industrial and medical contexts. In particular, workers in environments where gamma radiation is prevalent—such as nuclear facilities, research labs, or even hospitals—must arm themselves with knowledge of radiation exposure guidelines. It's not just about protecting oneself; it’s about fostering a culture of safety.
"Radiation safety isn't merely an option; it's a necessity that safeguards both individuals and communities, ensuring that benefits do not come at the cost of health."
Radiation Exposure Guidelines
When it comes to radiation exposure, the guidelines established by organizations like the International Commission on Radiological Protection (ICRP) provide essential benchmarks. Understanding how these guidelines translate to everyday exposure is crucial. Typically, guidelines are broken down as follows:
- Occupational exposure: The recommended limit is often around 20 millisieverts (mSv) per year, averaged over five years.
- Public exposure: For those not involved in radiation work, the limit is generally 1 mSv per year, excluding natural background radiation.
- Emergency situations: Temporary exposure limits may rise significantly, particularly in nuclear accidents, but remain tightly regulated.
Various factors can influence these limits, including age, health conditions, and the duration of exposure. For instance, children are considered more sensitive to radiation, prompting stricter guidance for their protective measures. Awareness of these benchmarks plays a crucial role in ensuring a safe environment when interacting with gamma radiation, either through work or medical treatment.
Protective Measures
Taking effective protective measures is non-negotiable in scenarios where gamma rays are involved. Here are some approaches that can be categorized into design, procedural, and personal safety measures:
- Shielding: For instance, lead and concrete are commonly used materials due to their effective attenuation properties against gamma radiation. Enclosures made from these materials are standard in nuclear facilities and radiotherapy units, minimizing exposure.
- Distance: In practical situations, such as in hospitals during radiological procedures, maintaining a safe distance from the source of radiation can significantly reduce exposure. This simple concept is essential; as the distance doubles, the exposure significantly decreases due to the inverse square law of radiation.
- Monitoring: Utilizing devices like dosimeters helps track exposure in real-time, providing immediate feedback to workers in radiation-heavy environments, thus enabling timely protective actions.
Incorporating a safety culture that emphasizes training and awareness is fundamental. Regular workshops to educate staff about radiation risks and safety protocols not only bolster the protection strategy but offer a sense of collective responsibility within teams.
Future Research Directions
Exploring the future directions in gamma ray research is crucial for advancing our understanding and applications of this unique form of radiation. Researchers are constantly probing the complexities that gamma rays present, which can lead to groundbreaking developments in multiple disciplines such as medicine, physics, and even environmental science. As we dive into the emerging technologies and potential discoveries in this field, the implications can be both transformative and enlightening.
Emerging Technologies
In the realm of gamma ray research, the development of new detection technologies is a cornerstone of future directions. Current detection methods, while effective, have their limitations—especially in terms of sensitivity and resolution. New technologies aim to overcome these hurdles by harnessing the potential of advanced materials and innovative design. For instance, the use of graphene-based sensors could revolutionize the way gamma rays are detected, allowing for quicker and more precise measurements in various environments.
Furthermore, the use of machine learning algorithms is gaining traction in the analysis of gamma-ray data. By training models on existing datasets, researchers could identify patterns and anomalies far beyond human capabilities.
"Emerging technologies not only improve detection and analysis but also help in the understanding of cosmic phenomena and fundamental particle physics."
Potential Discoveries
The path ahead in gamma ray research holds an array of potential discoveries that could reshape our understanding of the universe. One significant area of interest is the investigation of gamma ray bursts (GRBs). These brief but intense flashes of gamma radiation are among the universe's most powerful events, yet many mysteries shroud their origins and mechanisms. Advancements in observational technology, combined with robust theoretical models, may eventually uncover what triggers these violent phenomena and their role in cosmic evolution.
Delving further, researchers are also focused on the connections between gamma rays and dark matter. Some speculations indicate that gamma rays could provide hints about the nature of dark matter and its interactions. Identifying gamma ray emissions from dark matter annihilation could open a new chapter in our understanding of what constitutes the universe.
Closure
The study of gamma rays, as outlined in this article, signifies more than just a scientific endeavor; it represents a key pillar in our understanding of both the universe and practical applications in various fields. Gamma rays are not ordinary forms of radiation; their unique properties allow us to glimpse into the far reaches of space as well as deeper into the human body. The richness of this topic encompasses several vital elements that merit attention.
Summary of Key Points
Every section of the article has drawn a vivid image of gamma rays, focusing on:
- Definitions and Properties: An introduction to what gamma rays are, distinguishing them from different types of radiation.
- Origins: Insights into where these rays come from, including cosmic events and nuclear reactions.
- Detection Methods: Various techniques employed to measure gamma radiation, including scintillation and semiconductor detection.
- Applications: Areas such as medicine, astronomy, and nuclear physics where gamma rays play crucial roles.
- Future Directions: The ongoing research path and the potential new technologies and discoveries that could emerge.
These key points intertwine to showcase how vital a complete understanding of gamma rays is for scientific advancements and everyday applications.
The Importance of Ongoing Research
Research on gamma rays is not stagnant; it evolves and opens new doors to understanding. The potential for discovering new particles or phenomena related to gamma rays could fundamentally alter our comprehension of physics and cosmology. Moreover, ongoing investigations into their applications in medicine could lead to more effective therapies and imaging techniques. As scientists push the boundaries of what we know, the pursuit of knowledge regarding gamma rays not only enhances science as a discipline but also refines practical methodologies that directly influence everyday lives. The future is bright, and this light often comes in the form of gamma rays—reminding us that the universe has much more to reveal.