Understanding Dark Matter: Proportions and Implications
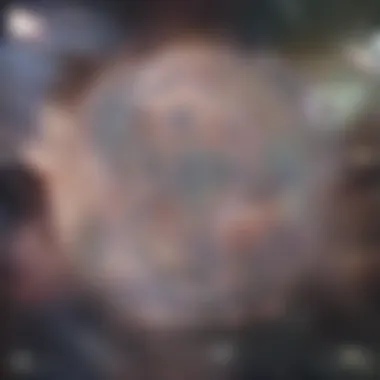
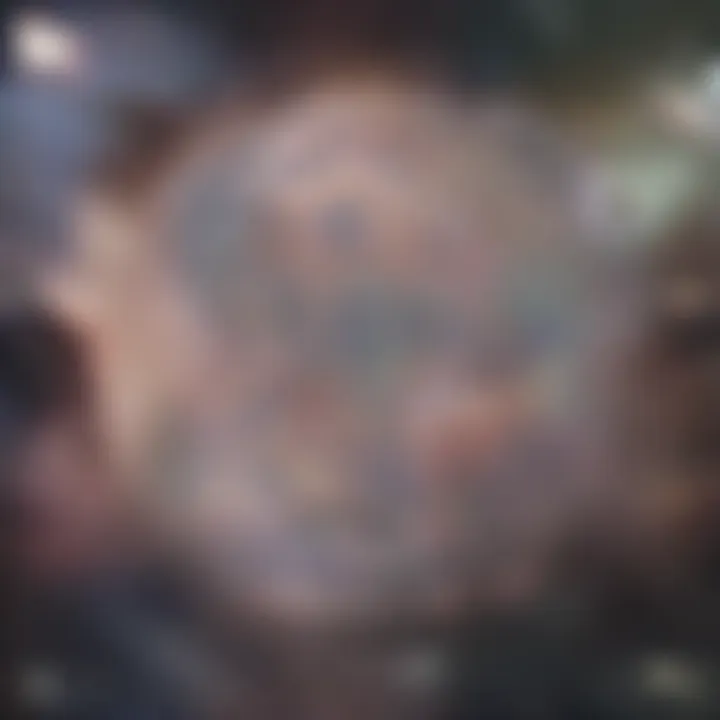
Intro
Dark matter remains one of the most compelling mysteries in cosmology and astrophysics. It is not visible in the traditional sense; rather, its presence is inferred from the effects it has on visible matter and radiation. The understanding of dark matter's role in the universe is critical for both theoretical and practical aspects of science, influencing how we understand galaxies, the formation of cosmic structures, and even the fundamental laws of physics. A myriad of recent findings has provided new insights into its properties and implications.
Key Research Findings
Overview of Recent Discoveries
In the last decade, research into dark matter has accelerated. Observations from telescopes, as well as findings from particle accelerators such as the Large Hadron Collider, have contributed to a deeper understanding of dark matter's characteristics. The prevailing consensus is that about 27% of the universe's mass-energy content is made up of dark matter, a striking figure that underscores its importance in the cosmos.
Recent studies, especially from the Hubble Space Telescope, have revealed how dark matter influences the formation and clustering of galaxies. These findings suggest that dark matter is not only a gravitational entity but may also interact through other, albeit weak, forces.
Significance of Findings in the Field
The significance of these discoveries cannot be overstated. Understanding dark matter informs the cosmic web structure, altering how we interpret galactic formations. It modifies all current models of cosmology, making it essential for further theories or predictive models about the universe's future. The realization that dark matter might interact with itself underlines the importance of developing innovative detection strategies, such as those employed in underground laboratories.
βDespite being elusive, dark matter holds the keys to understanding universeβs formation and evolution.β
Breakdown of Complex Concepts
Simplification of Advanced Theories
Understanding dark matter involves grappling with a range of complex theories, from weakly interacting massive particles (WIMPs) to axions. Each hypothesis adds layers of depth to the overall perspective of dark matter, providing potential pathways for detection and study. WIMP theory suggests that dark matter particles interact at a very low rate with normal matter, perhaps explaining its invisibility.
Visual Aids and Infographics
Visual aids can enhance the comprehension of dark matter concepts. Infographics depicting how dark matter gravitationally influences galaxies help to simplify abstract ideas. For instance, diagrams showing gravitational lensing can visually represent the effect dark matter has on light paths from distant galaxies.
Closing Remarks
This exploration into dark matter is ongoing. As technological advances continue, so too does the potential for groundbreaking discoveries. Researchers are committed to unveiling the secrets surrounding dark matter, and the implications for physics and cosmology are profound. Understanding this dimension of the universe will not only enhance theoretical comprehension but also inspire future generations of scientists.
Intro to Dark Matter
Dark matter represents one of the most significant enigmas in modern astrophysics. It is a concept that has emerged from extensive astronomical observations, yet it remains elusive and poorly understood. The significance of dark matter lies in its substantial influence on the large-scale structure of the universe. Without a grasp of this topic, one cannot fully appreciate various astronomical phenomena. Understanding dark matter is imperative not only for theoretical astrophysics but also for practical implications in cosmology and related fields.
Exploring dark matter involves delving into its definition and historical development. It fosters a better comprehension of the cosmos, allowing students, researchers, and professionals to connect various celestial phenomena to its presence and effects.
Defining Dark Matter
Dark matter is classified as matter that does not emit, absorb, or reflect any electromagnetic radiation. This characteristic makes it invisible and challenging to detect directly. Despite its undetectable nature, dark matter composes an estimated 27% of the universe's total mass-energy content. Its existence is inferred primarily through gravitational effects on visible matter, such as galaxies and galaxy clusters. The distinction between dark matter and visible matter, which makes up a mere 5% of the universe, is fundamental to our understanding of the cosmos.
Dark matter research primarily revolves around two fundamental inquiries: what it is made of and how it influences the universe's expansion and structure. Each of these aspects plays a crucial role in defining dark matter and determining its properties.
Historical Context
The concept of dark matter emerged in the early 20th century, fueled by observations made by astronomers such as Fritz Zwicky. In the 1930s, Zwicky examined the motions of galaxies within the Coma Cluster and noted discrepancies between the visible mass of galaxies and their expected gravitational influences. His findings suggested the presence of unseen massβthis was a pivotal moment in understanding the universe's structure.
Subsequent research, particularly by Vera Rubin, further validated the existence of dark matter through detailed rotational curves of spiral galaxies. Observations indicated that stars at the periphery of galaxies were rotating at speeds inconsistent with the gravitational pull of the visible mass. This led to the conclusion that galaxies must contain a substantial amount of unseen matter, which we now refer to as dark matter.
Over the decades, advancements in technology and observational techniques have deepened our understanding of dark matter. However, the exact nature of this enigmatic substance remains a vital question in contemporary astrophysics. Researchers continue to develop theories and search for concrete evidence of dark matter, striving to unlock its secrets and understand its profound implications.
The Composition of the Universe
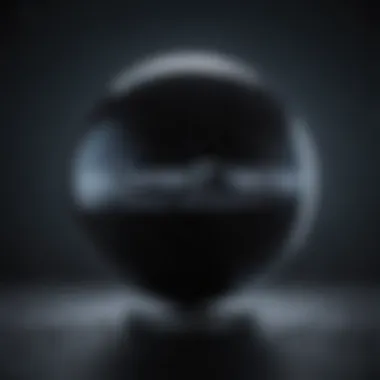
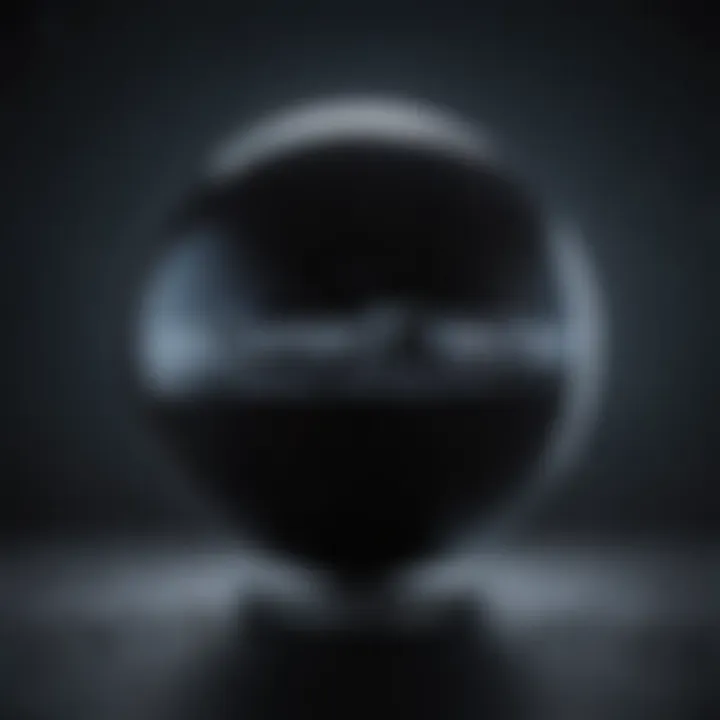
The composition of the universe is fundamental to understanding the cosmic tapestry. Comprising various elements, it shapes the roles of ordinary matter and dark energy, both crucial in a world that appears to be predominantly dark matter. While ordinary matter constitutes visible materials such as stars, planets, and gas, dark matter remains elusive, offering a stark contrast to our familiar universe. Dark energy, too, represents a mysterious force driving the expansion of the universe.
Ordinary Matter Explained
Ordinary matter is what we interact with daily and includes protons, neutrons, and electrons. This type of matter is visible and tangible. It makes up only about 5% of the universe. Stars, planets, and other celestial bodies exist largely due to ordinary matter's presence, which is also responsible for forming galaxies and creating the structures we observe. Within this category, the periodic table of elements offers a plethora of possibilities, making up everything from the lightest hydrogen to the heavier elements like iron and gold.
In terms of implications, the quantity of ordinary matter informs us about the processes at play in the universe. For instance, by studying the light emitted from distant stars, researchers infer the motion and distribution of matter within galaxies, providing clues about how galaxies form and evolve over time.
The Role of Dark Energy
Dark energy plays a critical role in the universe's structure and behavior. While ordinary matter behaves predictably under gravity's influence, dark energy opposes it by driving the accelerated expansion of the universe. Current estimates suggest that dark energy forms about 70% of the universe's total composition, making it a significant player in cosmic dynamics.
The presence of dark energy raises questions about the ultimate fate of the universe. If the expansion continues at an accelerated rate, one possible scenario is the "Big Freeze," where galaxies drift apart, leading to a cold and lonely cosmos. Alternatively, if dark energy were to change its nature, it could influence cosmic structures in unforeseen ways.
Understanding dark energy leads to engaging insights about ongoing research and the implications on our universe's fate. While scientists remain uncertain about its exact nature, studying dark energy continues to challenge prevailing cosmological models and push for deeper understanding in the field of astrophysics.
"Dark energy's influence is profound yet remains one of the greatest enigmas in modern cosmology, highlighting how much we still have to explore."
By examining the intricate balance between ordinary matter and dark energy, we can further grasp the universe's complexities and the gravitational forces that bind or drive it apart.
Quantifying Dark Matter
Understanding the proportions of dark matter is essential in the field of astrophysics. By quantifying dark matter, scientists can better understand the universe's structure and evolution. The implications of accurate measurements are vast. They affect estimates of galaxy masses, formation, and even the way we understand gravity. The nature of dark matter remains a puzzle, and quantifying its presence is a critical step toward unraveling that mystery.
Current Estimates
Percentage in the Universe
Current estimates suggest that dark matter makes up about 27% of the universe. This significant percentage highlights its importance. Knowing that a large part of the universe is composed of dark matter is a pivotal consideration in astrophysical studies, as it influences the behavior of galaxies and large-scale structures.
One key characteristic of these percentages is their reliance on multiple observational methods, from galaxy rotation curves to cosmic microwave background measurements. They are popular choices for discussion due to their implications in both cosmology and theoretical physics. The estimation is unique because it relies not only on direct measurements but also on indirect observations.
While this percentage provides crucial insights, it also has drawbacks. Disagreements among different methods can lead to variations in reported values. These disagreements might cause confusion among researchers and can complicate discussions about dark matterβs true nature in the universe.
Mass Estimates
Mass estimates of dark matter are equally crucial, as they dictate how we perceive the gravitational forces at play in galaxies. These estimates are typically around five times the mass of visible matter in the universe. This relationship underlines the dominance of dark matter's mass in cosmic interactions.
A key characteristic of mass estimates is their ability to reveal gravitational effects that would otherwise go unnoticed. They benefit the study of structure formation in the universe, helping researchers understand how galaxies evolve and interact.
However, the uniqueness of mass estimates lies in their dependency on cosmological modeling and assumptions about dark matter properties. The main advantage of these estimates is that they provide a quantitative grasp of reality. Yet, they are also subject to uncertainty, especially concerning the nature of dark matter particles themselves.
Variations in Research Findings
Research in dark matter has produced varying estimates and interpretations across multiple studies. These variations often stem from differing methodologies, assumptions about dark matter candidates, or the scale of observations. Some studies might focus on small galactic regions, while others assess broader cosmological scales. Such disparities highlight the continuing complexities surrounding dark matter research.
Scientists debate these findings vigorously, and while some support certain models, others may argue for alternatives. The ongoing discourse reflects the importance of advancing understanding and refining techniques for better quantification.
The challenges in dark matter research remind us that in science, numbers don't always tell the whole story, and context and method matter significantly.
Methods of Detection
Detecting dark matter is crucial in understanding its properties and role in the universe. Since dark matter does not interact with light and is invisible to traditional observational techniques, scientists rely on various methods to infer its presence and measure its effects. These methods provide vital insights into not only dark matter's nature but also the underlying physics governing cosmic structures.
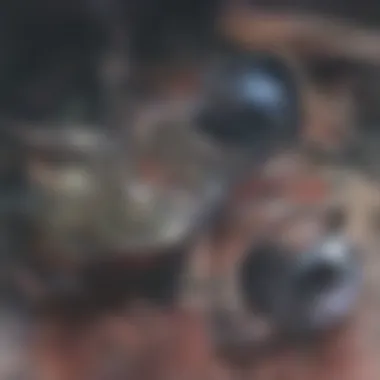
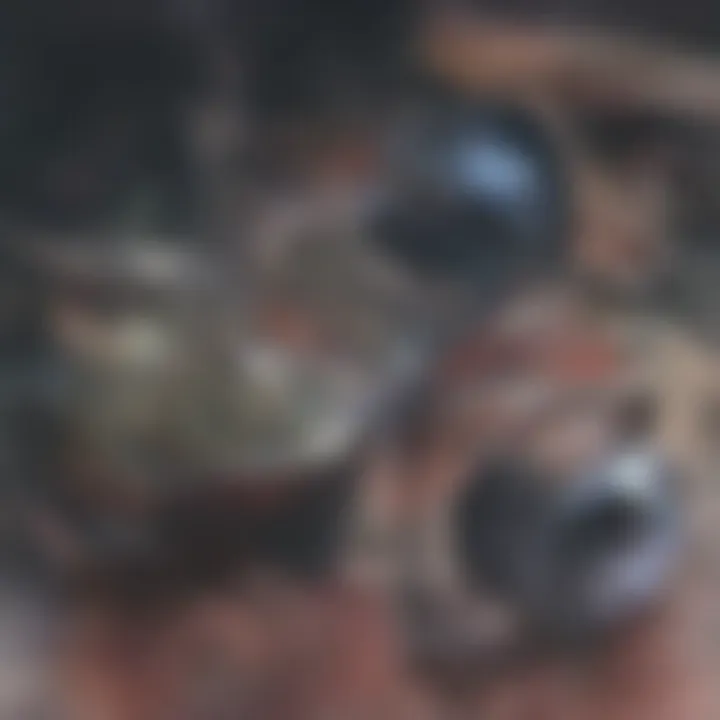
Gravitational Effects
One significant approach to detecting dark matter is studying its gravitational effects. Dark matter exerts gravitational pull on visible matter, energy, and the fabric of space itself, shaping the universe's structure and dynamics. Researchers observe this influence through several phenomena:
- Galaxy Rotation Curves: The velocities of stars in galaxies do not decrease as expected with distance from the galactic center. Instead, they maintain high speeds, suggesting an unseen mass, attributed to dark matter.
- Gravitational Lensing: Massive objects, like galaxy clusters, bend light from more distant galaxies. This effect allows scientists to map the distribution of dark matter, as the amount of bending reflects the gravity of both visible and hidden mass.
- Cosmic Microwave Background (CMB): The CMB provides a snapshot of the early universe. Analyzing its fluctuations helps in understanding density variations, which are influenced by dark matter.
These gravitational interactions support the existence of dark matter and hint at its substantial mass component within galaxies and larger cosmic structures.
Direct Detection Attempts
Efforts to detect dark matter directly involve sophisticated experiments aimed at identifying dark matter particles as they interact with ordinary matter. Such attempts are challenging due to the expected weak interactions. Some notable methodologies include:
- Cryogenic Detectors: These devices cool materials to very low temperatures to detect the minuscule energy deposits from dark matter interactions. Experiments like the Cryogenic Rare Event Search with Superconducting Thermometers (CRESST) utilize this method.
- Noble Gas Detectors: These detectors, such as the LUX-ZEPLIN experiment, use large volumes of liquid xenon. When dark matter particles collide with the nuclei, they produce detectable scintillation light and ionization.
- Underground Laboratories: To shield these experiments from cosmic radiation and other background noise, many detectors are located deep underground, such as the Sanford Underground Research Facility.
While direct detection remains elusive, advancements in technology and methodology provide hope for future breakthroughs. As researchers continue to refine these approaches, understanding dark matter becomes more attainable.
"An increasingly sophisticated range of experimental techniques is being developed which may ultimately allow us to probe the nature of dark matter itself."
As new findings emerge, the quest for direct detection forms a critical part of the broader mission to unravel the mysteries of dark matter.
Dark Matter Theories
Understanding dark matter is incomplete without exploring the theories that attempt to explain its nature and behavior. These theories not only provide insights into the composition and effects of dark matter but also open new avenues for research and experimentation. Theoretical approaches are crucial in guiding scientists through the profound gaps in our knowledge of the universe. By examining the various hypotheses surrounding dark matter, researchers can better direct their efforts in efforts to uncover and potentially detect this elusive substance.
The primary theories that have gained traction include Weakly Interacting Massive Particles (WIMPs), axions, and modified gravity theories. Each of these frameworks presents unique perspectives and implications for astrophysics and cosmology.
WIMPs and Axions
WIMPs are among the most investigated candidates for dark matter. They are hypothetical particles that interact through the weak nuclear force, which makes them challenging to detect. Their predicted mass ranges from a few GeV (giga-electronvolts) to several TeV (tera-electronvolts), placing them within the reach of various experimental setups designed to identify them. WIMPs are integral to many current models aiming to elucidate the structure of dark matter. Researchers believe that a significant portion of dark matter in the universe might consist of these particles.
On the other hand, axions are another compelling candidate. First proposed as a solution to the strong CP problem in quantum chromodynamics, axions are theorized to be extremely light and weakly interacting, making them difficult to observe. Their existence could explain several astrophysical phenomena, such as the rotation curves of galaxies, without resorting to modifications of known physical laws.
The interplay between WIMPs and axions suggests that multiple types of dark matter could exist and that upcoming experiments may one day offer insights into their actual nature.
Modified Gravity Theories
Modified gravity theories challenge the essential understanding of gravity that stems from Newtonian mechanics and Einstein's general relativity. The most notable among these is Modified Newtonian Dynamics (MOND), which suggests that galactic dynamics can be explained without invoking dark matter. According to MOND, the laws of gravity behave differently at low accelerations, leading to the observed phenomena that appear to require dark matter for explanation. This theory has drawn both interest and skepticism within the scientific community.
Another significant theory is the TeVeS (Tensor-Vector-Scalar) theory, which extends MOND by introducing additional fields to describe gravitational interactions. While these modified gravity theories offer alternative explanations, they still need to be reconciled with various observational evidence that currently aligns with dark matter models. The pursuit of answers in the realm of modified gravity may lead to a deeper comprehension of cosmic phenomena and a reconsideration of established physical principles.
By investigating both the particle candidates and modified gravity ideas, scientists continue to piece together the puzzle of dark matter. The implications are profound, not only for our understanding of the universe but also for the fundamental forces at play.
Astrophysical Implications
Dark matter plays a crucial role in the cosmos, influencing various astrophysical phenomena. Understanding its implications is essential for comprehending the structure and evolution of the universe. Without considering dark matter, current models of cosmology would fall short. This section will address significant aspects, including the formation and evolution of galaxies, along with cosmic structure and distribution.
Galaxy Formation and Evolution
The process of galaxy formation is heavily reliant on the presence of dark matter. Galaxies are not merely collections of stars and gas; rather, they form within vast halos of dark matter. These halos act as gravitational wells, attracting ordinary matter. This is important because dark matter helps to explain how galaxies could have formed so quickly after the Big Bang.
Key facts about this process include:
- Initial Conditions: Dark matter influenced the initial density fluctuations in the early universe, which eventually led to gravitational collapse.
- Hierarchy of Structure: As dark matter clumped together, it facilitated the hierarchical merging of smaller structures into larger galaxies.
- Stability of Galaxies: The gravitational pull of dark matter contributes fundamentally to the stability of galaxies, preventing them from being torn apart by their own angular momentum.
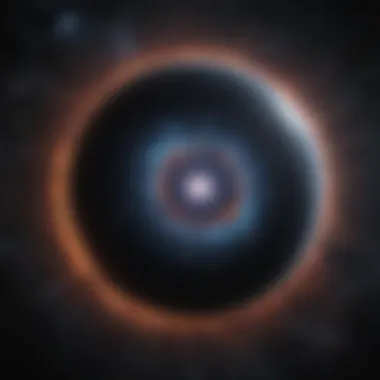
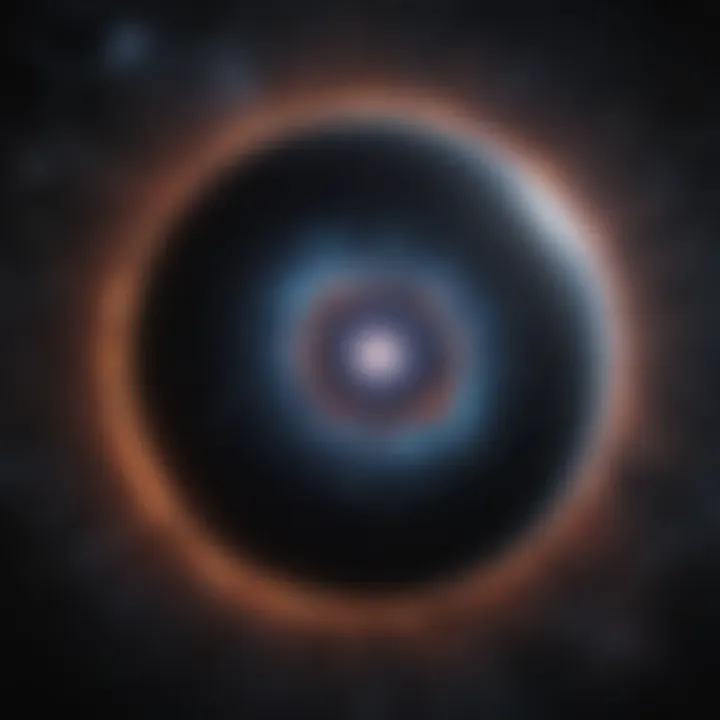
Dark matterβs role in galaxy evolution also extends to phenomena like galaxy collisions. When galaxies interact, the distributions of dark and ordinary matter do not overlap completely. This leads to observable differences in the dynamics and outcomes of such interactions. Understanding these processes can lead to insights about the past and future of galaxies, contributing to the broader narrative of cosmic history.
Cosmic Structure and Distribution
The distribution of dark matter throughout the universe affects cosmic structures on a grand scale. Dark matter is not homogeneous; instead, it forms intricate web-like structures, known as the cosmic web. This structure provides a framework for understanding how galaxies and clusters of galaxies are positioned within the universe.
Key points about cosmic structure include:
- Galaxy Clusters: The presence of dark matter influences the formation of galaxy clusters, the largest gravitationally bound structures in the universe.
- Lensing Effects: Gravitational lensing, caused by dark matter's mass, distorts the light from distant objects. This effect serves as a critical tool for mapping dark matter distributions indirectly.
- Large Scale Structure: Large scale surveys of the universe, like the Sloan Digital Sky Survey, reveal how dark matter influences the arrangement of galaxies, confirming theories about structure formation.
"Dark matter is hardly seen, but its effects are all around us. Understanding these effects is the key to grasping the universe's underlying fabric."
In summary, the astrophysical implications of dark matter go beyond academic interest. They touch on fundamental aspects of how the universe operates, shaping the trajectory of galaxies while influencing cosmic order itself. A thorough comprehension of dark matter leads to a richer understanding of both local and expansive astronomical phenomena.
The Search for Dark Matter
Understanding dark matter involves both theoretical explorations and practical experiments. As scientists attempt to decipher the universeβs dark components, the search for dark matter becomes a linchpin in cosmic research. It informs our comprehension of not just the makeup of the universe, but also how galaxies and cosmic structures form and evolve. Hence, the importance of this topic cannot be overstated.
The complexity of dark matter necessitates innovative methods for its detection and study. Numerous scientific ventures are underway; they aim to identify the traits and behaviors of dark matter. This ongoing search is crucial for confirming existing theories and potentially discovering new ones.
Ongoing Experiments
Current experiments focused on dark matter cover a range of approaches. These ongoing investigations primarily aim to detect dark matter particles directly or indirectly. Various methods are utilized to scrutinize cosmic phenomena for evidence of dark matter's influence.
- Large Underground Xenon (LUX) Experiment: Located in South Dakota, this experiment uses liquid xenon to search for weakly interacting massive particles (WIMPs). The darker, deeper underground placement reduces interference from cosmic rays, increasing the chances of detecting dark matter.
- Cryogenic Rare Event Search (CRESST): This experiment focuses on detecting energy deposited by dark matter interactions in cryogenic detectors. By cooling its materials to near absolute zero, CRESST enhances sensitivity to low-energy recoils.
- PandaX: Conducted in China, PandaX is another experiment searching for WIMPs. It also employs liquid xenon technology, similar to LUX, and aims to analyze interactions through high-resolution detection methods.
These experiments showcase the breadth of approaches being utilized in the search for dark matter. Their results provide invaluable data that can validate or challenge prevailing theories.
Future Directions in Research
Looking ahead, the search for dark matter will evolve with advancements in technology and deeper understanding of quantum mechanics. Future directions may include:
- Larger Scale Detectors: Scientists envisage building larger and more sensitive detectors. These new instruments would enhance the ability to gather data and discover rare events linked to dark matter.
- Astrophysical Observations: Continued observations of galaxies and cosmic structures are needed. Future telescopes could detect signatures of dark matter by analyzing gravitational effects on visible matter.
- Multi-Wavelength Astronomy: Using information from different wavelengths could reveal a more holistic view of dark matter. Collaborating across various disciplines in astrophysics will likely yield new insights.
"The hunt for dark matter continues, driven by the universal quest to understand the cosmos."
As we enter new frontiers in exploration, understanding dark matter is likely to influence many scientific developments. The commitment to unraveling this mystery reflects the inherent curiosity and ingenuity of the human spirit.
Culmination
The conclusion of this article encapsulates the critical aspects of dark matter, addressing both its theoretical significance and empirical implications. Understanding dark matter is essential as it composes approximately 27% of the universe's total mass-energy content. This knowledge shapes our interpretation of cosmic phenomena and underlying physical laws.
Summary of Findings
Throughout this exploration, we identified key points regarding dark matter:
- Dark matter is a foundational element in modern cosmology, impacting our understanding of galaxy formation and cosmic structure.
- Current estimates suggest that dark matter significantly outweighs ordinary matter, yet it remains undetectable through conventional means.
- Various detection methods are ongoing, including experiments designed to observe gravitational effects and potential direct interactions.
These findings highlight the dual nature of dark matter as both a theoretical concept and a subject of active research. Grasping these facets is crucial for anyone involved in astrophysics or related fields.
The Importance of Dark Matter
Recognizing the importance of dark matter extends beyond academic curiosity. It influences several aspects:
- Scientific Endeavors: Understanding dark matter could revolutionize physics, offering insights into forces and particles beyond the Standard Model.
- Astrophysical Understanding: Dark matter's role in galaxy formation elucidates the history of the universe and its evolutionary processes.
- Technological Advancement: Continuous research on detection methods has the potential to lead to new technologies benefiting other fields, from energy to materials science.
Ultimately, the significance of dark matter lies in its ability to challenge existing paradigms, pushing us toward a deeper comprehension of the universe. Advancements in this domain will likely yield profound revelations, shaping our future understanding of cosmic realities.
"The search for dark matter is not just about solving a scientific puzzle; it is about expanding the very definitions of the universe we inhabit."
This article invites readers to continue exploring the enigma of dark matter, underscoring its relevance for both theoretical research and practical applications.