Understanding Dark Matter: A Comprehensive Exploration
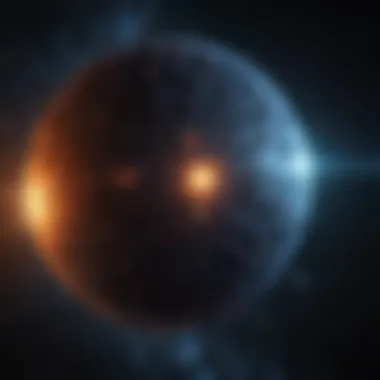
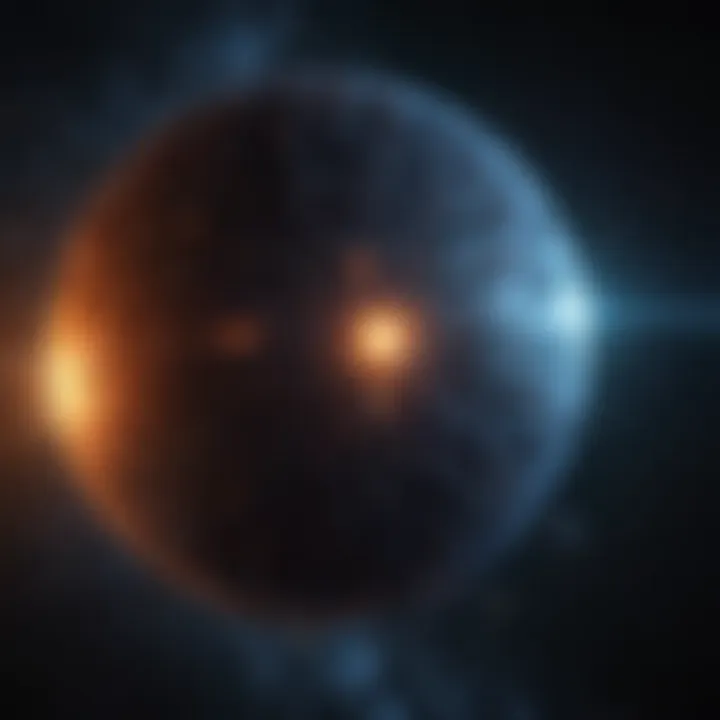
Intro
Dark matter represents a pivotal element in our understanding of the universe. Even though it cannot be seen directly, its presence is inferred through various astronomical observations. Grasping the nature of dark matter is crucial for explaining the structure and behavior of galaxies, galaxy clusters, and the universe as a whole. As such, this article seeks to illuminate the complexity surrounding dark matter by breaking down key concepts and findings that have emerged in recent research.
Key Research Findings
Overview of Recent Discoveries
Recent advancements in astrophysics have revealed significant insights into dark matter. Observations from instruments like the Hubble Space Telescope and the Atacama Large Millimeter Array have provided data supporting the existence of dark matter. One notable discovery is the gravitational lensing effect, which shows how light from distant galaxies bends around invisible mass, indicating dark matter’s influence.
Another crucial finding is related to the cosmic microwave background radiation, which serves as a relic of the early universe. Detailed measurements from the Planck satellite have helped quantify dark matter's role in shaping the large-scale structure of the universe. These breakthroughs highlight the undeniable impact of dark matter in cosmic evolution.
Significance of Findings in the Field
The implications of these findings are profound. Understanding dark matter guides researchers in formulating more accurate models of the universe. It is essential for simulating how galaxies form and evolve. Moreover, it challenges existing theories in particle physics and cosmology, thus reshaping scientific discussions surrounding the universe's composition.
"Without dark matter, the structure of the universe as we know it would be utterly different."
The recognition of dark matter's critical role reaffirms the need for continued research in both theoretical and experimental domains.
Breakdown of Complex Concepts
Simplification of Advanced Theories
While dark matter may seem elusive, breaking it down into understandable elements can facilitate better comprehension. The predominant theory is that dark matter consists of weakly interacting massive particles, or WIMPs. These hypothetical particles are expected to interact very weakly with normal matter, thus remaining undetected by conventional means.
Additionally, different models propose variations like axions and sterile neutrinos as potential constituents of dark matter. Understanding these theories allows researchers to approach dark matter from multiple perspectives, stimulating innovative approaches in the quest to uncover its nature.
Visual Aids and Infographics
Visual representations can aid in understanding the more complex aspects of dark matter. Infographics illustrating the distribution of dark matter in the universe or animated models of galaxy formation can convey information succinctly.
For instance, one might encounter a diagram showcasing gravitational lensing effects or a chart detailing characteristics of various dark matter candidates. Engaging with such materials enhances the learning experience and encourages deeper inquiry.
Foreword to Dark Matter
Dark matter is a cornerstone of modern astrophysics, crucial for comprehending the structure and evolution of the universe. This section sets the stage for understanding not just what dark matter is, but why its exploration is profoundly important within the larger context of cosmology.
The significance of dark matter arises from its pervasive influence on cosmic formations and dynamics. While it remains elusive, its gravitational effects shape galaxies and govern the motions of celestial bodies. Therefore, breaking down the concept of dark matter is essential for anyone seeking to grasp the fundamentals of how the universe operates.
Overview of Dark Matter
Dark matter refers to a form of matter that does not emit, absorb, or reflect light, making it invisible to current observational technologies. It is estimated that dark matter makes up about 27% of the universe's total mass-energy content. This means that the visible matter, including stars and galaxies, constitutes only a small fraction of the universe.
Researchers have coined the term "dark matter" to encapsulate the nature of this mystery. Though it cannot be directly observed, its presence is inferred from various astronomical phenomena. The term signifies much about the challenges scientists face in trying to comprehend this unseen substance. The quest for understanding dark matter leads to deeper inquiries about the fabric of reality itself.
This section outlines how dark matter's properties differ from those of ordinary matter, laying the groundwork for subsequent discussions on its implications and evidence.
Importance in Cosmology
The study of dark matter is paramount for several reasons. Firstly, it holds key insights into the formation of the universe itself. The gravitational forces exerted by dark matter played a pivotal role in clumping matter together, leading to the formation of the first stars and galaxies. Without dark matter, the current structure of the cosmos would be vastly different.
Moreover, dark matter impacts the behavior of galaxies. The rotation speeds of galaxies cannot be accounted for by visible mass alone, leading to the conclusion that additional, unseen mass must be present. This observation helps to correct models of galaxy formation and provides context for cosmic evolution.
In summary, understanding dark matter is not just an academic exercise. It is crucial for piecing together the vast and complex puzzle of the universe. Researchers continue to seek answers because these discoveries will inevitably affect our understanding of physics at a fundamental level.
"Dark matter is the unfathomable. It's not just a component of the universe; it’s a concept that challenges the very foundations of how we view reality."
As the article unfolds, it will explore the various facets of dark matter, from historical perspectives to modern scientific endeavors, all of which illuminate its significance in the grand narrative of cosmology.
Defining Dark Matter
Defining dark matter is essential for comprehending its influence on the universe. Dark matter is not visible directly; thus, defining it requires an understanding of various indirect observations and theoretical frameworks. Recognizing what dark matter entails helps clarify its role in astrophysics and cosmology. Furthermore, it allows researchers and educators to present dark matter as a significant aspect of the cosmos.
Historical Context
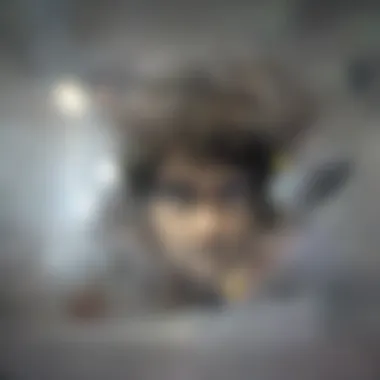
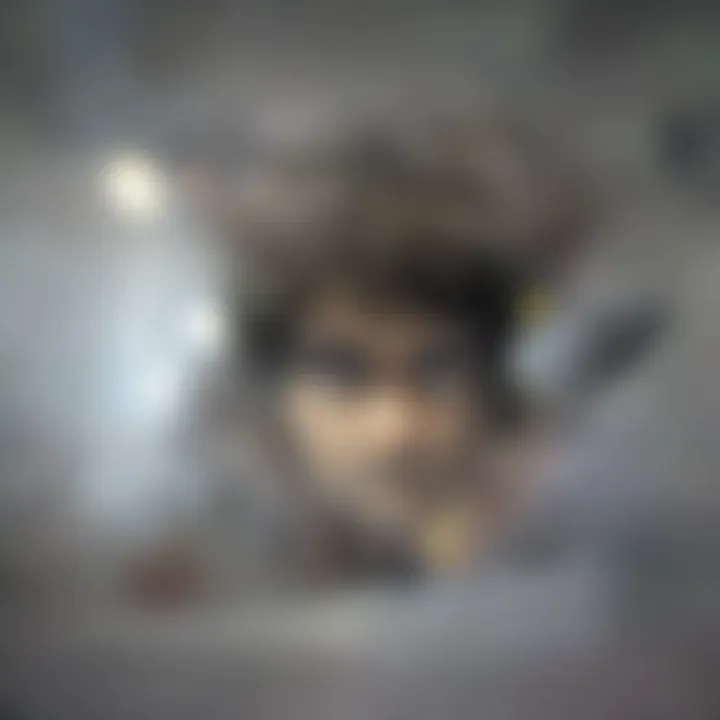
The journey to understand dark matter began in the early 20th century with scientists like Fritz Zwicky. In 1933, Zwicky studied the Coma Cluster and noted that the mass visible was not enough to account for the observed gravitational binding of its galaxies. He coined the term "dunkle Materie," or dark matter, suggesting that unseen mass existed to hold the galaxies together. Despite initial skepticism, this notion gained traction.
In the 1970s, Vera Rubin's work on galaxy rotation curves confirmed Zwicky's findings. Observations revealed that stars at the outer edges of galaxies moved at speeds that should not be possible if only visible mass were present. This reinforced the idea that a large portion of the universe's mass is dark and could not be directly detected, leading to a paradigm shift in astrophysics.
Modern Definitions
Today, dark matter is widely defined as a form of matter that does not emit or interact with electromagnetic radiation. Its presence is inferred primarily through gravitational effects. Physicists categorize dark matter mainly into two groups: baryonic and non-baryonic.
- Baryonic dark matter refers to conventional matter, which includes protons, neutrons, and electrons, but in forms that are not luminous, such as brown dwarfs, black holes, and cold gas clouds.
- Non-baryonic dark matter is composed of particles that do not interact through electromagnetic forces, including Weakly Interacting Massive Particles (WIMPs), axions, and sterile neutrinos.
The consensus in astrophysics currently leans toward a universe predominantly made up of non-baryonic dark matter, comprising about 27% of the total mass-energy content of the universe. The remaining components are dark energy, which contributes about 68%, with ordinary baryonic matter making up a mere approximately 5% of the universe.
Research continues to refine these definitions, year by year, through experiments in particle physics and observations from telescopes, exemplifying the dynamic nature of our understanding of dark matter.
Evidence for Dark Matter
The investigation into dark matter's existence is essential in modern astrophysics. Understanding the evidence for dark matter helps underscore its pivotal role in the universe's structure, dynamics, and evolution. This section reviews observational evidence and theoretical predictions. Collectively, they create a compelling case for dark matter as a necessary component in our cosmological models.
Observational Evidence
Observational evidence for dark matter emerges from various astronomical phenomena. Each type provides unique insights into the nature and distribution of dark matter in the universe.
Galaxy Rotation Curves
Galaxy rotation curves present a critical piece of evidence for dark matter. These curves portray the speed at which stars orbit the center of galaxies. Based on visible matter, one would expect that stars further from the galactic center move slower. However, observations reveal that outer stars maintain high speeds, indicating significant undetected mass.
The key characteristic of galaxy rotation curves is their flat profile at greater distances from the center. This contrast with classical gravitational predictions strongly suggests the presence of unseen mass—dark matter.
A unique feature of galaxy rotation curves is their reliance on rotational dynamics rather than electromagnetic observations. This reduces biases related to light emission. However, one disadvantage is the dependence on initial assumptions about mass distribution, which may not always be accurate.
Gravitational Lensing
Gravitational lensing describes the bending of light from distant objects due to the gravitational influence of massive bodies. This effect reveals both the presence of dark matter and its distribution in the universe. Observations show that light from galaxies behind massive clusters is distorted, indicating the existence of a significant amount of unseen mass.
The fundamental aspect of gravitational lensing is its capacity to measure mass through the geometry of light. As a tool, it provides direct evidence of dark matter's presence in galaxy clusters, situating it as a strong choice for this article.
However, the unique feature of gravitational lensing lies in its indirect nature, requiring assumptions about the shapes and positions of background objects. This complexity presents challenges for precise mass measurements.
Cosmic Microwave Background Radiation
Cosmic microwave background radiation (CMB) is the remnant radiation from the Big Bang and serves as another vital piece of evidence for dark matter. The CMB provides a snapshot of the early universe, revealing temperature fluctuations, which correlate with density variations. These variations indicate the presence of dark matter.
The key characteristic of CMB is its isotropy and homogeneity, providing a uniform backdrop against which variations can be studied. This makes it immensely beneficial as a tool for understanding early cosmic structure and validating dark matter models.
Nonetheless, the unique features of CMB fluctuations can be influenced by various factors, including energy density and cosmic expansion rates. Thus, it poses challenges in isolating the direct effects attributed solely to dark matter.
Theoretical Predictions
Theoretical predictions regarding dark matter offer important frameworks that guide observational strategies. Many models speculate on dark matter's behavior and interactions based on empirical observations. Furthermore, predictions often aim to reconcile discrepancies found in existing data.
These theoretical foundations have significant implications for ongoing and future research. Focusing on specific traits of predicted dark matter candidates can effectively narrow down the type of evidence scientists seek. Continuous refinement of these theories is crucial to building a more accurate depiction of how dark matter operates within our vast universe.
Properties of Dark Matter
The properties of dark matter are essential for understanding its role in the universe. Dark matter makes up a significant portion of the total mass-energy content of the cosmos. Despite being invisible and detectable only through its gravitational effects, its properties influence galaxy dynamics, structure formation, and the overall evolution of the universe. Dark matter provides a framework for theorizing about missing mass in galaxies and clusters. Without proper knowledge of these properties, our grasp of cosmic phenomena would be severely limited.
Composition
The composition of dark matter remains one of the most critical questions in modern astrophysics. Currently, dark matter is understood to be non-baryonic, meaning it is not made up of protons, neutrons, or electrons—components that constitute ordinary matter. There are various candidates for dark matter particles, including Weakly Interacting Massive Particles (WIMPs), axions, and sterile neutrinos. Each of these candidates provides an intriguing avenue for research.
- WIMPs: WIMPs are popular in theoretical physics due to their predicted mass and interactions. They provide a robust framework under the Cold Dark Matter paradigm.
- Axions: Axions, often proposed to resolve the strong CP problem in quantum chromodynamics, are lightweight particles that may also account for dark matter.
- Sterile Neutrinos: These particles do not interact via the standard weak interactions but could play a role in the formation of cosmic structures.
The study of dark matter's composition helps to bridge findings from particle physics and cosmology. Each candidate suggests different properties and behaviors, making this area ripe for exploration. Finding the true composition could unlock fundamental mysteries about the universe.
Interactions and Forces
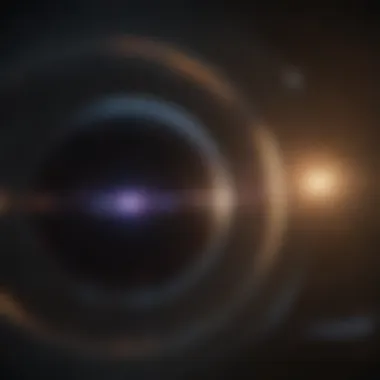
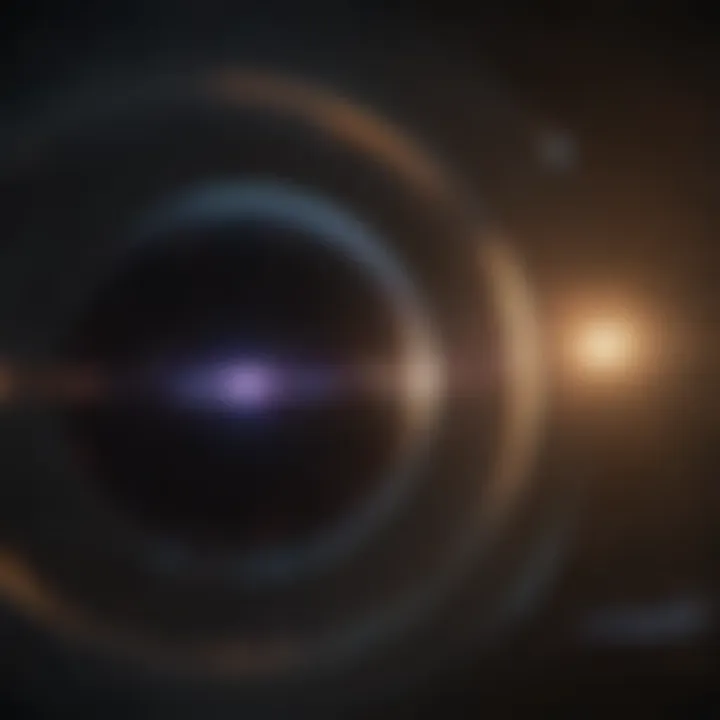
Dark matter interacts primarily through gravity, making it difficult to detect directly. However, the implications of its interactions affect cosmic structures significantly. Dark matter does not emit, absorb, or reflect light, which is why it is termed 'dark.'
The forces at play include gravitational attraction, which facilitates the clustering of matter in galaxies and affects the cosmic web's large-scale structure. There is growing interest in research related to possible non-gravitational forces. If dark matter particles can interact through other forces, it may open new paths for detection and understanding. Some potential interactions being explored include:
- Elastic scattering: If dark matter can scatter off normal matter, it could lead to detectable signals in specific experimental setups.
- Self-interaction: This concept pertains to the interactions between dark matter particles themselves, which could have implications for galaxy formation and evolution.
Understanding these interactions is vital to framing dark matter theories. Insights into how dark matter behaves under different conditions may offer hints at its fundamental nature. As future experiments refine detection techniques, new knowledge about these properties will likely reshape our understanding of dark matter and its role within the cosmos.
Theoretical Frameworks
The study of dark matter requires robust theoretical frameworks to explain its properties and behavior. These frameworks help scientists to form predictions about the universe's structure and evolution. Understanding these concepts provides insights into how dark matter influences galaxies and cosmic phenomena. Researchers can design experiments and observational studies based on these theoretical models. Furthermore, different frameworks suggest various interactions between dark matter and ordinary matter, guiding the direction of ongoing research.
There are primarily two commonly accepted frameworks concerning dark matter. These models not only characterize dark matter's nature but also its role in cosmic history. The frameworks differ in their approach and assumptions, which leads to various implications for future research.
Cold Dark Matter Models
Cold dark matter models propose that dark matter consists of particles moving slowly compared to the speed of light. This behavior implies that they have negligible thermal motion. The assumption of a slow-movement scenario allows the clustering of dark matter to occur in denser regions of the universe. Such clustering is essential for forming structures, like galaxies, in the early universe.
Cold dark matter is often associated with weakly interacting massive particles, or WIMPs. Many experiments are designed to detect WIMPs, but so far, no conclusive evidence has been found. This model is crucial because it lays the foundation for understanding cosmic structure formation, including the observable universe we see today.
Warm and Hot Dark Matter Models
In contrast to cold dark matter, warm dark matter models suggest that the particles possess some thermal velocity that is significant. Warm dark matter could lead to different clustering behavior. Particles classified under this model may include sterile neutrinos or lighter particles that can travel at considerable speeds. This variation in velocity would alter how structures form and evolve in the universe. The warm and hot dark matter paradigms can provide alternative explanations for the large-scale structures and specific cosmic phenomena that cold dark matter may struggle with.
Both warm and hot models hold distinct implications for how scientists understand the universe. Theoretical physicists must rigorously examine these concepts to assess their viability when contrasted with observational evidence. The ongoing interplay among these frameworks will continue to shape the future of dark matter research and cosmology.
Alternative Theories to Dark Matter
Alternative theories to dark matter are essential to the ongoing quest for understanding the universe. While dark matter is a widely accepted explanation for certain cosmic phenomena, some scientists propose different frameworks to help explain observations that current models struggle to account for. These alternative theories can shed light on the limitations of dark matter as an explanation and encourage exploration of novel concepts that could transform our understanding of the cosmos.
Modified Gravity Theories
Modified gravity theories offer a perspective that challenges the existence of dark matter by proposing that our understanding of gravity may be incomplete. One prominent theory in this domain is Modified Newtonian Dynamics (MOND). MOND posits that at very low accelerations, typical of galactic outskirts, the laws of motion behave differently than predicted by Newton's laws. This can potentially explain the observed rotation curves of galaxies without invoking dark matter.
- Key Attributes:
- Redefines the force experienced by a body at low accelerations
- Suggests that gravitational effects can mimic dark matter's effects
Another approach is TeVeS (Tensor–Vector–Scalar Gravity), which extends general relativity by introducing additional fields. This theory aims to address not only the dynamics of galaxies but also the cosmic microwave background radiation observations.
The exploration of modified gravity theories is crucial because it invites a reevaluation of existing models and assumptions about fundamental physics. Critics often argue that these theories, while intriguing, struggle to explain phenomena universally observed across the universe. Thus, the debate continues in the scientific community about their validity as alternatives to dark matter.
Emerging Hypotheses
Emerging hypotheses reflect the cutting edge of research into cosmic phenomena. These proposals often arise from anomalies or new data that existing frameworks cannot explain adequately. One such idea involves the concept of self-interacting dark matter. It posits that dark matter particles could interact with one another through forces outside of the conventional electromagnetism or gravity.
- Implications for Research:
- Could explain certain clustered galactic phenomena
- Offers a unique explanation of cosmic structure formation
Another idea is inspired by quantum mechanics. This hypothesis suggests that dark matter might fundamentally be tied to properties of quantum fields, possibly linking it to known particles or forces via mechanisms not yet understood.
Exploring these emerging hypotheses allows for a more nuanced understanding of the universe. They challenge scientists to adapt and expand their theoretical models, potentially leading to significant discoveries.
Ongoing research is vital, as each proposed alternative can expand our grasp of the universe and ultimately lead to a more cohesive theory of dark matter, or its feasible replacements, that satisfactorily accounts for observed phenomena.
Experimental Searches for Dark Matter
Experimental searches for dark matter hold significant importance in advancing our understanding of this elusive cosmic component. The exploration of dark matter is not merely a theoretical exercise; it shapes the future of astrophysics and cosmology. Through various experimental approaches, researchers aim to uncover evidence that could point toward the nature and properties of dark matter particles. This section examines two prominent methods employed in the quest to detect dark matter: direct detection experiments and indirect detection approaches.
Direct Detection Experiments
Direct detection experiments focus on identifying dark matter particles by observing their interactions with regular matter. These experiments are often conducted underground or in shielded environments to minimize interference from cosmic rays and other background noise. By using sensitive detectors typically made from materials like germanium or xenon, scientists aim to spot the rare collisions between dark matter particles and atomic nuclei.
One prominent example of a direct detection experiment is the LUX-ZEPLIN (LZ) project, which operates in the Sanford Underground Research Facility in South Dakota. The design of such setups allows researchers to measure tiny energy changes when dark matter particles collide with a nucleus. The results hold significant implications for existing models of particle physics and our overall understanding of the universe.
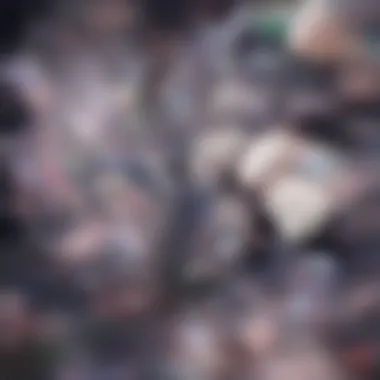
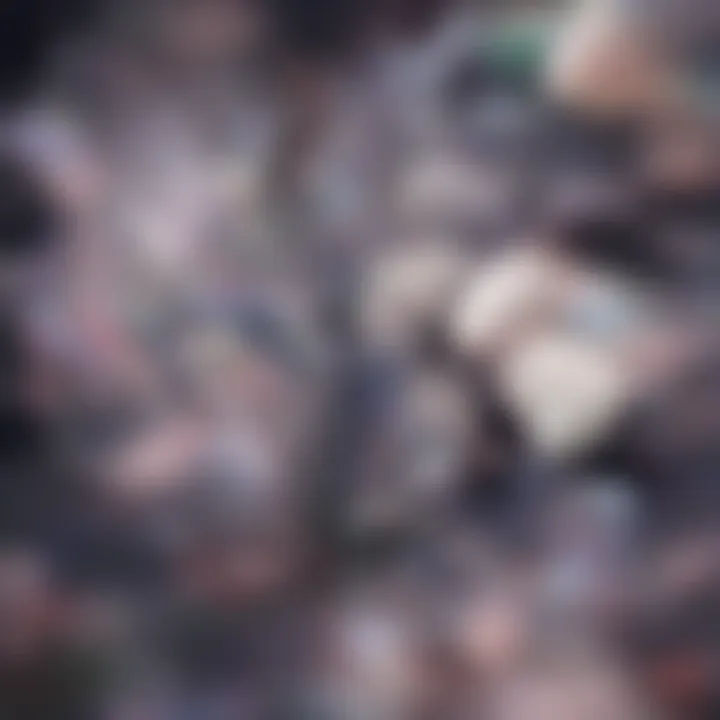
The success of direct detection experiments hinges on the ability to achieve high sensitivity. Advances in technology have improved the performance of these detectors. However, challenges remain. The predicted interaction rates of dark matter particles can be exceedingly low, making it a difficult task to discern a signal from the noise.
Indirect Detection Approaches
Indirect detection approaches take a different route. Instead of seeking to register direct collisions, these methods look for the byproducts of dark matter interactions. When dark matter particles annihilate or decay, they can produce standard particles like photons, neutrinos, and other particles detectable by telescopes and observatories.
One notable project in this category is the Fermi Gamma-ray Space Telescope. It observes gamma rays emitted from regions with high dark matter density. This includes the centers of galaxies and potential dark matter halo regions surrounding galaxies. The strength and nature of these emissions can shed light on the properties of dark matter particles.
Additionally, astrophysical neutrinos detected by experiments such as IceCube can provide insights into dark matter characteristics. Understanding these interactions amplifies our knowledge of cosmic events and the role of dark matter in shaping the observable universe.
In summary, both direct detection and indirect detection approaches are essential for uncovering the mysteries surrounding dark matter. Each method offers unique advantages and encounters specific challenges that researchers must navigate. As studies progress, they promise to reveal more about this key component of our universe.
Implications of Dark Matter
The study of dark matter carries significant implications for our understanding of the universe. Not only does it influence the structure and formation of galaxies, but it also reshapes our comprehension of cosmic evolution. Understanding dark matter is essential for several reasons.
- Galaxy Formation: Dark matter plays a critical role in how galaxies form and evolve. The gravitational effects of dark matter serve as the scaffolding for ordinary matter, allowing galaxies to coalesce and grow. This means that without dark matter, stars and galaxies may not exist as we know them today.
- Cosmic Structure: The distribution of dark matter in the universe impacts large-scale structures such as clusters of galaxies. These massive structures are crucial for studying the history and dynamics of the universe. Dark matter’s properties help scientists to understand the patterns observed in cosmic web structures.
- Testing Theories of Physics: Dark matter challenges existing theories of physics, particularly in terms of gravity and fundamental forces. Research into dark matter could potentially lead to groundbreaking discoveries that reshape our understanding of natural laws.
- Future Research Directions: Dark matter is a fundamental focus of ongoing astrophysical research. Improvements in technology and detection methods may one day unravel the mysteries surrounding it, leading to new theories and insights about the universe’s composition.
"The study of dark matter is not merely an academic exercise; it touches the very foundations of our understanding of the universe."
Role in Galaxy Formation
The role of dark matter in galaxy formation is profound. When the universe was still young, it was mostly homogeneous. However, over time, slight density fluctuations led to regions where dark matter accumulated. This accumulation provided the necessary gravitational pull, allowing gas and dust to gather and eventually form stars and galaxies.
The distribution of dark matter influences the shapes and sizes of galaxies. For instance, galaxies with a higher concentration of dark matter tend to be more massive. This influences not just their formation, but also their fate.
Influence on Cosmic Structure
Dark matter affects the influence on cosmic structure significantly. Its presence dictates how galaxies and galaxy clusters are organized in the universe. The invisible mass influences gravitational interactions, leading to larger structures forming a cosmic web.
Understanding this influence helps scientists in several areas:
- Simulation of Universe Evolution: Models that incorporate dark matter are essential for simulating how the universe evolves over billions of years.
- Mapping Structures: By studying the gravitational effects of dark matter, astronomers can map regions of the universe that are otherwise invisible.
- Insight into Cosmic Events: Events like mergers between galaxies or the formation of superclusters are better understood with the role of dark matter taken into account.
In summary, the implications of dark matter are vast and multifaceted. They extend from galactic formation to the very structure of the cosmos. Understanding these implications allows scientists to paint a clearer picture of the universe's history and its underlying mechanics.
Future Directions in Dark Matter Research
Future directions in dark matter research not only shape how scientists engage with the mysteries of the universe but hold potential to dramatically alter our understanding of the cosmos. With advancing technology and increasing collaboration among researchers worldwide, the study of dark matter is on the brink of finding answers to long-standing questions. World patterns can emerge from these answers, influencing both theoretical physics and astronomical observations. This section will explore the anticipated technological advances and the potential discoveries that could arise in the near future.
Predicted Advances in Technology
Technology plays a pivotal role in unraveling the dark matter mystery. The quest for dark matter detection relies on innovations that improve sensitivity and precision. Several key technological advances are predicted:
- Sensitivity in Detectors: Equipment like the Large Hadron Collider and underground laboratories, such as the Large Underground Xenon experiment, are expected to enhance the sensitivity of detection techniques. These advancements aim to capture weaker signals associated with dark matter interactions.
- Quantum Sensors: Utilizing quantum mechanics, researchers are investigating sensors that could provide unprecedented precision in detecting dark matter particles. Such sensors might significantly improve the ability to measure gravitational effects related to dark matter.
- Machine Learning: The application of machine learning in data analysis allows for more effective mining of vast datasets. It can help in identifying patterns and anomalies that suggest dark matter signals.
The integration of these cutting-edge technologies will enable scientists to approach dark matter research from fresh angles. Collaboration among institutions will also drive progress.
Potential Discoveries
The future is ripe with possibilities, and potential discoveries in dark matter research could have far-reaching implications for physics and cosmology. Some anticipated discoveries include:
- Identification of Dark Matter Particles: As detection methods improve, researchers are hopeful they will finally identify dark matter particles and unveil their properties. Understanding the composition of dark matter could revolutionize physics.
- Understanding Cosmic Evolution: Discoveries related to dark matter may provide crucial insights into the formation and evolution of galaxies. This could lead to deeper comprehension of how cosmic structures develop.
- Connections to Other Physics: The study of dark matter might bridge gaps between established physics and new theories. It may help explain anomalies in particle physics, such as neutrino behavior.
The implications of these discoveries extend beyond academia. They may reshape educational curriculums and influence technological advancements in various fields.
"Understanding dark matter is not just a quest to find a piece of the universe; it’s an invitation to rethink the very laws that govern our reality."
End
Summary of Key Points
In this article, we explored the multifaceted concept of dark matter, delving into its definition, significance, and the evidence that supports its existence. We discussed how dark matter plays a crucial role in cosmology, impacting galaxy formation and cosmic structure. Furthermore, we reviewed the theoretical frameworks that scientists have developed to explain dark matter, including cold, warm, and hot dark matter models. We also acknowledged alternative theories that challenge traditional views, alongside ongoing experimental searches aimed at detection.
An overview of the future directions in dark matter research highlighted the promise of technological advancements and potential discoveries that could reshape our understanding of the universe. This exploration allowed us to bridge the gap between advanced theoretical models and accessible knowledge, making the topic relatable to a wider audience.
Final Thoughts on Dark Matter
Dark matter remains one of the profound mysteries in modern astrophysics. Its elusive nature encourages continuous inquiry and represents a significant frontier in scientific research. The quest to understand dark matter not only enhances our grasp of the universe's structure but also compels us to question fundamental aspects of reality itself.
Given that dark matter constitutes about 27% of the universe, its influence on cosmic phenomena cannot be overstated. The interplay between dark matter and visible matter, as well as the forces that govern these interactions, stimulates critical thought and innovative research. Continued exploration is essential, as the implications of dark matter extend beyond astrophysics, potentially influencing other fields such as particle physics and cosmology.
By fostering a deeper understanding of dark matter, we move closer to grasping the intricate workings of the cosmos. This pursuit is not merely academic; it has the capacity to unveil critical truths about our existence. As scholars and scientists continue this journey, there lies a hope for transformative revelations that could illuminate the enigma of dark matter.