Understanding Dark Matter: A Deep Dive
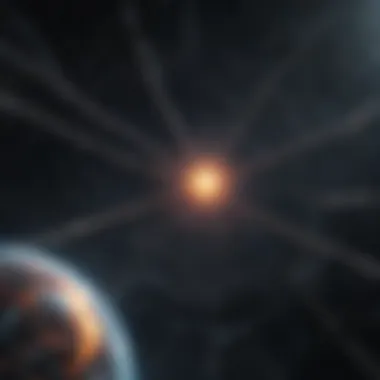
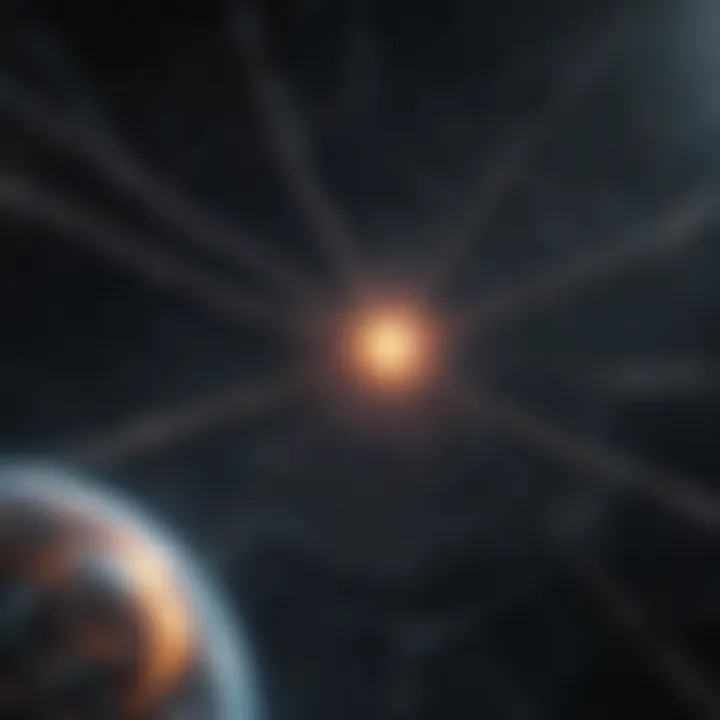
Intro
Dark matter is a concept that has intrigued scientists and the general public alike for decades. Despite being fundamental to our understanding of the universe, it eludes direct observation. Traditionally, we perceive matter as anything with mass that occupies space. However, dark matter is different; it does not emit, absorb, or reflect light, making it invisible and detectable only through its gravitational effects.
Various studies and astronomical observations have provided compelling evidence for the existence of dark matter. For instance, scientists have noted that galaxies rotate at such speeds that they should fly apart, yet they remain intact. This phenomenon suggests the presence of some unseen mass exerting gravitational pull, which we attribute to dark matter.
In the upcoming sections, we will explore the evolution of dark matter theories, key research findings that support its existence, and modern research initiatives aimed at uncovering its nature. We will also simplify complex theories regarding dark matter, making them accessible to a broad audience interested in cosmology and physics.
Key Research Findings
Overview of Recent Discoveries
Recent advancements in astronomy and particle physics have provided significant insights into the existence of dark matter. The Cosmic Microwave Background radiation, a remnant from the Big Bang, has been instrumental in shaping our understanding of the universe's composition. Data from the Planck satellite has helped map the distribution of dark matter in the universe. Additionally, measurements of galaxy clusters reveal that the visible matter we observe is only a fraction of the total mass present.
Other research avenues include investigations through gravitational lensing. This technique allows scientists to measure the bending of light from background objects by massive foreground objects, thereby inferring the presence of dark mass. These methods show that about 27% of the universe is made up of dark matter, while ordinary matter constitutes only 5%.
Significance of Findings in the Field
The findings related to dark matter are pivotal not just for cosmology but also for our understanding of fundamental physics. They challenge existing theories and encourage the development of new models to account for discrepancies in observable phenomena. For instance, the presence of dark matter provides a framework to explain cosmic structures' formation and evolution. These discoveries have implications that reach beyond individual galaxies; they influence our perceptions of the universe's fate and its overall fate in the grand cosmic timeline.
Breakdown of Complex Concepts
Simplification of Advanced Theories
Understanding dark matter requires grappling with advanced theoretical frameworks. The Cold Dark Matter model is a widely accepted theory suggesting that dark matter particles move slowly compared to the speed of light. This model helps explain how structures in the universe form and cluster over time. Dark matter is believed to be composed of yet undiscovered particles, and theories like WIMPs (Weakly Interacting Massive Particles) or axions have been proposed to fill this gap.
Visual Aids and Infographics
Visual representation can significantly enhance comprehension of dark matter's impact on structures within the universe. For example, infographics that illustrate the rotation curves of galaxies emphasize the difference between expected and observed rotation speeds. Such aids can provide clarity on how dark matter contributes to gravitational frameworks on a cosmic scale.
"The distinction between dark matter and regular matter highlights the immense unknowns in our understanding of the universe."
Prelude to Dark Matter
Dark matter is a pivotal concept in modern astrophysics. It presents significant implications for our understanding of the universe. Essentially, it is forms a substantial part of the total mass in galaxies and galaxy clusters, yet it is invisible and does not emit or interact with electromagnetic radiation. Understanding dark matter is crucial as it affects the structure and behavior of galaxies. Moreover, it plays a critical role in the formation of the universe and the cosmic structure we observe today.
It is estimated that dark matter constitutes about 27% of the universe. This statistic underlines its dominance compared to ordinary matter, which makes up only about 5%. Addressing the mysteries of dark matter entails an intersection of various scientific domains, including physics and cosmology. This knowledge is equally important for students and researchers alike, inspiring further investigations.
What is Dark Matter?
Dark matter refers to a type of matter that does not emit light or energy. As a result, it cannot be detected directly through existing telescopes or sensors. Scientists infer its presence through gravitational effects on visible matter. This was first indicated in the early 20th century, but the concept gained substantial traction following the observations of galaxies and galaxy clusters.
The evidence lies in phenomena such as the rotation curves of galaxies. Stars that are further from the center of a galaxy rotate slower than expected based on visible matter alone. The implication is that there is more mass in these galaxies, exerting gravitational pull that is not accounted for merely by observable stars and gas.
Why it Matters in Cosmology
The importance of dark matter in cosmology cannot be overstated. Dark matter acts as a framework around which galaxies form and galaxy clusters evolve. Understanding dark matter is essential for constructing accurate models of the universe. Without accounting for dark matter, many observed cosmic structures would be inexplicable.
Additionally, its influence extends beyond galaxies. It helps explain the large-scale structure of the universe itself. As scientists attempt to create reliable cosmological models, dark matter remains a key component. It sparks critical questions about the fundamental nature of the universe and the unseen forces governing it.
In summary, the role of dark matter is fundamental in both theoretical and practical aspects of cosmology, allowing researchers to interpret vast amounts of astronomical data comprehensively.
Historical Context
Understanding the historical context of dark matter is crucial for grasping how it fits into the broader narrative of cosmology. The evolution of thought regarding dark matter shows the shift from classical views of the universe to the more complex models we understand today. This section discusses significant milestones that shaped our recognition of dark matter as an integral part of cosmic structure.
Early Theories of the Universe
Before the existence of dark matter was recognized, early theories of the universe focused more on visible celestial bodies and their interactions. Ancient astronomers such as Aristotle considered the universe to be made up of five elements: earth, water, air, fire, and aether; the latter being a celestial quintessence. However, these theories did not consider the gravitational effects of unseen mass.
As the centuries passed, the heliocentric model proposed by Copernicus in the 16th century and the laws of planetary motion devised by Kepler began to shift the focus toward a more mathematical understanding of the cosmos. Newtonโs law of universal gravitation further enhanced this view by showing how mass attracts mass. Yet, the universe remained largely limited to what could be observed through telescopes.
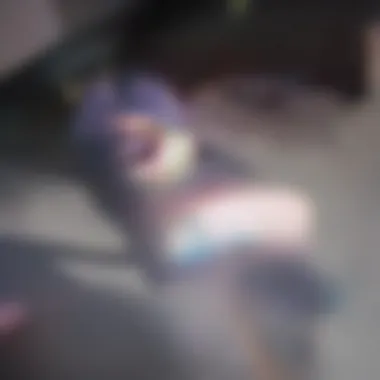
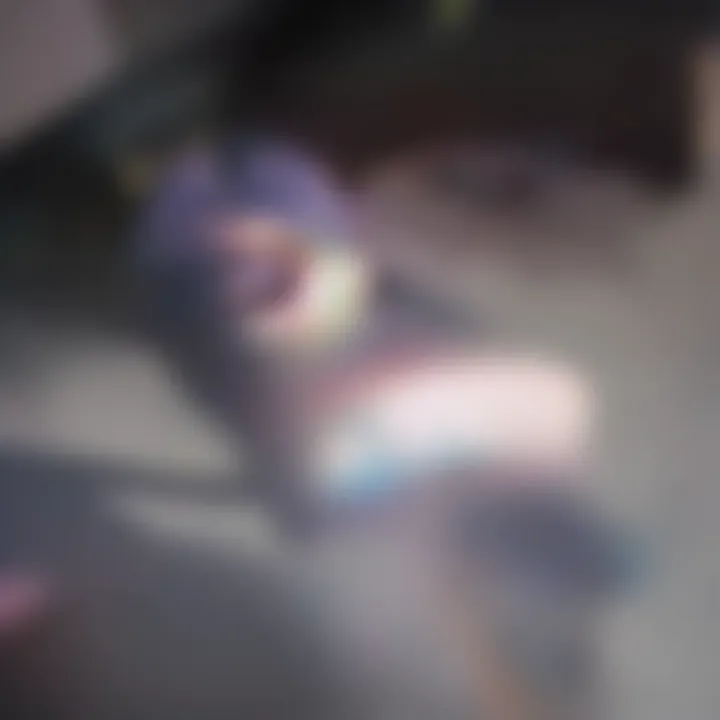
The 20th century witnessed groundbreaking developments as the field of astronomy expanded. Edwin Hubbleโs observations in the 1920s suggested that our universe is expanding, leading to considerable speculation about its overall structure. Despite these advancements, many questions remained. Notably, an explanation for the rotation of galaxies was still lacking. This laid the groundwork for theories that would eventually support the idea of dark matter.
The Discovery of Dark Matter
The term "dark matter" emerged after astronomers began observing phenomena that could not be explained solely by visible matter. In the 1930s, Swiss astronomer Fritz Zwicky studied the Coma Galaxy cluster and noticed that it moved in a way that suggested a significant amount of unseen mass was influencing its motion. He estimated that about 90% of the mass was not visible, proposing the existence of dark matter to account for the discrepancy.
Further evidence arose from the analysis of galactic rotation curves in the 1970s. Vera Rubinโs work indicated that stars in galaxies were rotating at such speeds that the visible mass could not account for the gravitational forces required to keep the stars in orbit. This reinforced the need for dark matter in explaining the observed behaviors of galaxies.
The 1980s and 1990s saw a proliferation of studies supporting dark matter theories, further solidifying its acceptance within the scientific community. The development of the Lambda Cold Dark Matter model (ฮCDM) became foundational for modern cosmology, specifying that dark matter plays a crucial role in the structure and evolution of the universe.
Evidence for Dark Matter
The concept of dark matter, an invisible component of the universe, is underscored by several strands of compelling evidence. This section explores the key pieces of data that reinforce the existence of dark matter and discusses the implications of these findings for our understanding of cosmic architecture. The significance of evidence in the realm of dark matter cannot be overstated; it is the backbone of the scientific inquiry into phenomena that challenge conventional physics.
Galactic Rotation Curves
Galactic rotation curves provide one of the most direct pieces of evidence for dark matter. Observations show that stars orbiting in the outer regions of galaxies move at velocities that do not decrease as expected from Newtonian physics. Instead, they maintain high speeds, which suggests that galaxies contain much more mass than we can see. This discrepancy implies the presence of a significant amount of unseen mass, which is what we term dark matter.
In a typical spiral galaxy, like the Milky Way, the distribution of visible matter alone cannot account for the rotation speeds observed. When plotting a graph of velocity against distance from the galactic center, the expected curve based on visible mass drops off quickly. However, the actual observed curves remain flat. This flat rotation curve means that there is more mass present in the galaxy than just the stars and gas. This missing mass points toward the existence of dark matter enveloping these galaxies and contributing to their gravitational pull.
Gravitational Lensing
Gravitational lensing is another critical phenomenon supporting dark matter's existence. General relativity predicts that massive objects should bend light that passes nearby, acting like a lens. When astronomers observe distant galaxies that seem distorted around clusters of galaxies, it enhances our understanding of dark matter.
The degree of this lensing effect provides a way to map the mass of the lensing clusters. Surprisingly, the distribution of mass derived from gravitational lensing calculations often reveals more mass than can be seen through optical measurements. This additional mass is attributed to the presence of dark matter, which shapes the gravitational field and influences the path of light.
Cosmic Microwave Background Radiation
The Cosmic Microwave Background Radiation (CMB) is the afterglow of the Big Bang, permeating the universe. Measurements of the CMBโs minute fluctuations are pivotal for understanding the early universeโs composition, including dark matter. These fluctuations reveal information about the density of matter and energy in the universe shortly after the Big Bang.
Studies show that the density of baryonic matter, or visible matter, is insufficient to account for the observed structure of the universe. The presence of dark matter is inferred from the observations of clumping in the CMB. The nature of the fluctuations creates models that predict how matter would coalesce under the influence of gravity. The resulting predictions include a dark matter component, which is essential for explaining the large-scale structure we see today.
"The evidence for dark matter is multi-faceted, ranging from the dynamics of galaxies to cosmic background radiation. These pieces of evidence collectively shape our understanding of the universe's missing ingredients."
Theoretical Frameworks
Theoretical frameworks provide the foundation for understanding dark matter, guiding both new hypotheses and experimental design. The gravity of this subject comes from the necessity to explain the unseen mass that affects structure and behavior of the universe. Alternative frameworks often arise in response to gaps or inconsistencies observed in standard models of physics. This exploration of different frameworks helps to illustrate the versatile approach scientists take to grapple with dark matter's elusive nature.
Modified Newtonian Dynamics (MOND)
Modified Newtonian Dynamics, commonly known as MOND, proposes adjustments to Newton's laws of motion to account for galactic dynamics without invoking dark matter. Developed by Mordehai Milgrom in the early 1980s, MOND suggests that the force of gravity weakens at very low accelerations, which are typically found at the edges of galaxies. This modification aims to explain phenomena such as the flat rotation curves of galaxies, which conventional gravity fails to adequately address.
In MOND, the expected gravitational effects of visible matter do not align with observed motions in many cases. By altering Newton's laws, MOND presents a potential solution to the missing mass problem. It has generated substantial discussion within the astrophysics community. While MOND can effectively fit certain observations, its incompatibility with other domains, such as cosmological observations, limits its acceptance as a complete theory.
Supersymmetry and Dark Matter Candidates
Supersymmetry stands as a compelling theoretical framework that extends the Standard Model of particle physics. This theory posits a relationship between bosons and fermions, predicting the existence of partner particles which could constitute dark matter. The most notable candidates arising from supersymmetry include the lightest supersymmetric particle (LSP), often considered a strong contender for dark matter.
Supersymmetry offers benefits like ensuring the stability of elementary particles and providing solutions to hierarchy problems. However, experimental validation of supersymmetry has proven elusive, with large particle colliders, such as the Large Hadron Collider, failing to detect these predicted particles as of now. Theoretical advances continue to refine supersymmetry models, keeping it a key player in the discourse surrounding dark matter.
WIMPs and Axions
Weakly Interacting Massive Particles, or WIMPs, represent one of the leading candidates for dark matter. These particles are postulated as massive, neutral, and predicted to interact primarily through gravity and the weak nuclear force. WIMPs naturally arise from many theories involving supersymmetry, making them a prominent subject in dark matter research.
On the other hand, axions offer another intriguing possibility. Axions are hypothetical elementary particles proposed as a solution to the strong CP problem in quantum chromodynamics. They are extremely light and weakly interacting, making them difficult to detect yet suitable as dark matter candidates.
Both WIMPs and axions continue to receive extensive attention in experimental searches. Underground detectors and particle colliders are currently set up to hunt for their existence. This ongoing research aims to provide more clarity on dark matter's fundamental properties and its role within the universe.
Theoretical frameworks are essential in guiding the search for dark matter candidates, shaping our understanding of fundamental physics, and enhancing observational strategies.
In summary, exploring various theoretical frameworks, including MOND, supersymmetry, and particle candidates such as WIMPs and axions, enriches the understanding of dark matter and delineates avenues for future investigations.
Current Research Initiatives
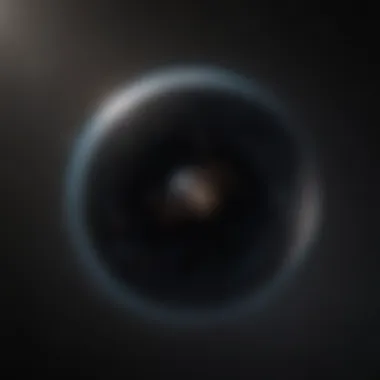
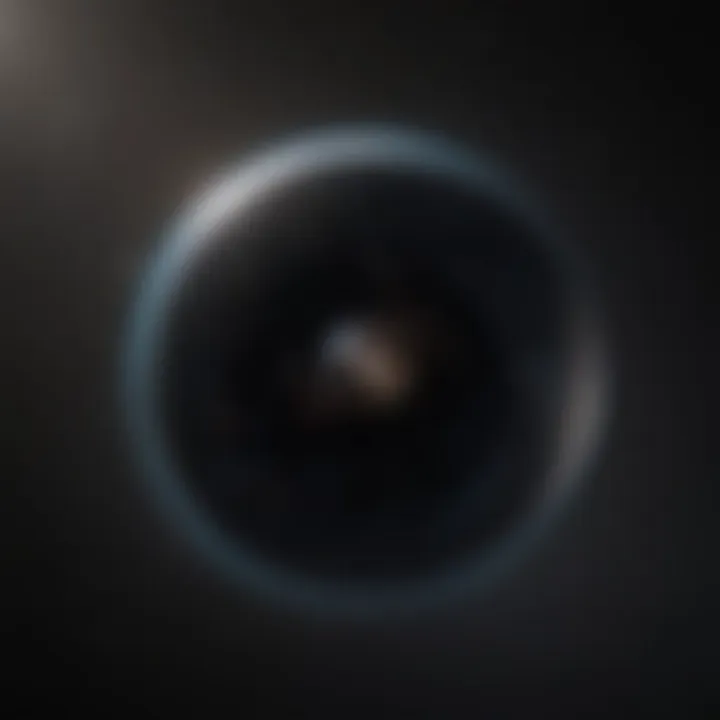
Current research initiatives in dark matter are vital for expanding our understanding of this mysterious cosmic entity. These initiatives aim to uncover the nature of dark matter and its role in the universe's evolution. By employing various scientific methods, researchers hope to gather significant evidence and provide insights that could reshape our knowledge of fundamental physical laws.
Large Hadron Collider Experiments
One of the most significant platforms for dark matter research is the Large Hadron Collider (LHC), located at CERN. The LHC conducts high-energy particle collisions to explore the fundamental components of matter. The experiments at the LHC seek to create conditions similar to those just after the Big Bang.
Theories suggest that certain particles associated with dark matter could be produced in these collisions. For example, Weakly Interacting Massive Particles (WIMPs) are potential dark matter candidates that physicists are eager to detect. The LHC experiments have a dual purpose: to find new particles and to test beyond the Standard Model theories, which may include dark matter.
Observational Studies with Telescopes
Observational studies using advanced telescopes play a critical role in dark matter research. Telescopes such as the Hubble Space Telescope and newer projects like the James Webb Space Telescope provide substantial data regarding the structure and distribution of galaxies. These observations help astronomers analyze the gravitational effects of dark matter.
By studying phenomena such as galaxy rotation curves and gravitational lensing, researchers collect crucial evidence about the existence of dark matter. More importantly, data from these studies can inform scientists about the potential characteristics and distribution of dark matter across the universe.
Underground Detectors
Underground detectors aim to directly observe dark matter particles. These experiments are placed deep underground to shield them from cosmic rays and other background noise that could interfere with detection. The aim is to identify rare interactions between dark matter particles and normal matter.
Examples of these detectors include the XENON1T and LUX-ZEPLIN projects. They utilize various technologies, such as liquid xenon and germanium crystals, to capture any signals of dark matter interactions. The success of these experiments could lead to groundbreaking discoveries regarding the constituents of dark matter.
It is through these varied research initiatives that scientists hope to piece together the puzzle of dark matter, leading to a better understanding of the universe as a whole.
Implications of Dark Matter
The implications of dark matter extend far beyond its existence. Understanding dark matter provides significant insights into the fundamental nature of the universe. It shapes our comprehension of cosmic structures, influences the dynamics of galaxies, and affects the architecture of the cosmos itself. The existence of dark matter has led to new cosmological theories and prompted questions at the intersection of physics and philosophy. Exploring dark matter not only allows for advancements in cosmology but also prompts reconsiderations of particle physics and how we interpret the universe.
Cosmological Models
Dark matter is a cornerstone in modern cosmological models. The presence of dark matter helps to explain the formation of galaxies and galaxy clusters. In the prevailing Lambda Cold Dark Matter model (Lambda CDM), dark matter acts as a scaffold for ordinary matter. As the universe expanded after the Big Bang, regions that contained higher densities of dark matter attracted more baryonic matter. Consequently, the gravitational pull of dark matter has been critical in forming the large-scale structures we observe today.
Incorporating dark matter into cosmological models allows scientists to simulate the universe's evolution more accurately. It elucidates phenomena like the cosmic microwave background radiation, observed through satellites such as the Wilkinson Microwave Anisotropy Probe (WMAP). The fluctuations in this radiation align with the gravitational effects of dark matter, reinforcing the validity of dark matter's role in cosmic evolution.
Structure Formation in the Universe
The implications of dark matter on structure formation are profound. It enables the clumping of galaxies and influences their interaction. Without dark matter, the visible mass of the universe would be insufficient to hold galaxies together through gravitational attraction.
The process of structure formation initiated in the early universe continues today. Dark matter, through its interplay with baryonic matter, leads to the emergence of complex structures like galaxy filaments and voids. Observational evidence from the Sloan Digital Sky Survey bolsters these theories, confirming that dark matter findings align with the distribution and dynamics of observed galaxies.
Potential Effects on Particle Physics
Dark matter also has ramifications in the field of particle physics. The search for dark matter candidates has steered researchers toward theories beyond the Standard Model. Candidates like Weakly Interacting Massive Particles (WIMPs) and axions are explored vigorously. Furthermore, a deeper understanding of dark matter could illuminate gaps in our current understanding of fundamental forces and particles.
Experiments conducted at facilities like the Large Hadron Collider aim to detect dark matter directly or indirectly. Discovering a dark matter particle may not only validate its existence but also reshape our understanding of particle interactions at high energies. This can potentially lead to groundbreaking discoveries about the nature of matter and the universe.
Dark Matter and Energy
Dark matter plays a crucial role in understanding the universe, particularly when discussing dark energy. This relationship is essential because it links two of the most significant and enigmatic components of our cosmos. This section explores the interplay between dark matter and dark energy, focusing on their definitions, their implications for cosmology, and the ongoing challenges researchers face.
Relation to Dark Energy
Dark energy is often described as a mysterious force that is driving the accelerated expansion of the universe. It constitutes a substantial portion of the universeโs total energy density. While dark energy is understood as a repulsive force, dark matter serves as the opposite, exerting gravitational attraction. This contrast suggests that comprehending one helps illuminate the nature of the other.
Research indicates that dark matter's gravitational effects influence the structure of galaxies and cosmic formations, while dark energy affects their expansion. It is crucial to understand that these two elements form the backbone of the current cosmological model. To resolve the mysteries surrounding dark energy, scientists investigate the properties of dark matter, examining how their interaction influences cosmic evolution. Recent studies suggest they might not be entirely independent.
"The relationship between dark matter and dark energy is pivotal for solving the fundamental questions about our universe's fate and structure."
Impact on Universe Expansion
The impact of dark matter on the universe's expansion cannot be overstated. Observations suggest that the universe's expansion is accelerating, a phenomenon primarily attributed to dark energy. However, dark matter influences this expansion indirectly through the gravitational forces it exerts on visible matter. This creates local gravitational wells where galaxies can cluster, thus balancing out the effects of expansion driven by dark energy.
Several models have emerged to describe how these interactions occur. They propose that while dark energy drives the large-scale structure of the universe apart, dark matter contributes to the clumping of matter in the universe, affecting the overall cosmic expansion rate.
In summary, dark matter and dark energy are intricately linked. Disentangling their complex relationship presents numerous challenges but is necessary for a rounded understanding of the universe. Cosmic observations, such as the Supernova studies and Cosmic Microwave Background Radiation analyses, often rely on this relationship to extract accurate cosmological parameters.
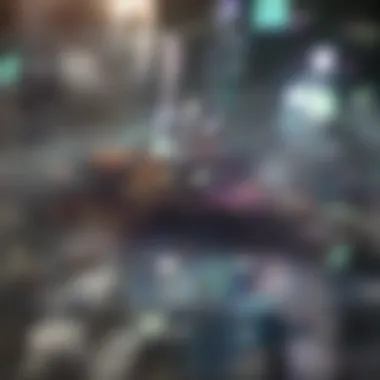
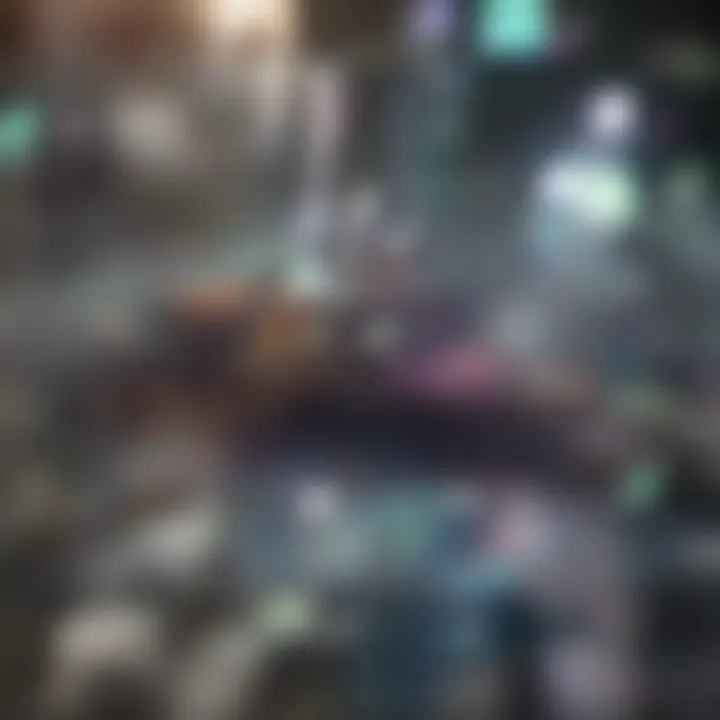
Understanding dark matterโs role is vital for predicting the universe's long-term fates, such as the Big Freeze or Big Crunch scenarios. Research into these areas remains vital for scientists hoping to achieve a fuller understanding of matter-energy dynamics in cosmology.
The Future of Dark Matter Research
The exploration of dark matter is still in its early stages. As scientists continue to delve into this enigmatic substance, researchers find themselves grappling with both exciting opportunities and significant challenges. Developing a deeper understanding of dark matter is crucial. It ties directly to our grasp of the cosmos and the fundamental laws of physics. In coming years, the methods and technologies used to study dark matter are likely to evolve, leading to new discoveries.
Upcoming Experiments and Observations
Various upcoming experiments are set to enhance our knowledge of dark matter. These initiatives aim to detect dark matter particles directly. A notable experiment is the LUX-ZEPLIN (LZ) at the Sanford Underground Research Facility. This facility aims to capture interactions of dark matter with ordinary matter using ultra-sensitive detectors. Another project is the Pico experiment, focusing on finding light dark matter. Both projects have innovative methods to help researchers make sense of dark matter.
In addition to underground labs, observatories are also essential to dark matter research. The James Webb Space Telescope is expected to unveil new insights into galactic structures influenced by dark matter. It may provide data about the formation of galaxies during the early universe.
Researchers are excited about how these new observations can inform us more about dark matter.
Theoretical Advances on the Horizon
The theoretical framework surrounding dark matter may also see significant advancements. Researchers struggle to explain the nature of dark matter particles fully. However, theories such as supersymmetry and string theory may yield new insights. These frameworks suggest the possibility of new particles that could be a part of the dark matter puzzle, enhancing overall understanding of the universe's structure.
Additionally, the development of more rigorous mathematical models is critical. Some scientists are exploring modified gravity theories as alternatives to dark matter explanations. This includes concepts such as f(R) gravity. Refining these theories may guide experimental efforts toward validating or disproving them.
"The future of dark matter research hinges on both experimental data and theoretical interpretations, each informing the other in a dynamic interplay."
In summary, research on dark matter is entering an exciting phase. The blend of novel experiments and theoretical approaches offers a promising avenue for discovery. As researchers continue their work, we may come closer to unraveling the mysteries of dark matter and its role in the cosmos.
Challenges in Dark Matter Studies
Understanding dark matter presents a series of complex challenges that impede our capacity to fully grasp its nature and significance. These obstacles not only complicate ongoing research but also necessitate innovative approaches in detection and theoretical interpretation. By examining these challenges, we can appreciate the multifaceted nature of dark matter and its crucial role in cosmological studies.
Detection Challenges
One of the primary issues researchers face is detecting dark matter directly. Since dark matter does not emit, absorb, or reflect light, traditional astronomical methods become ineffective. The existing theories suggest that dark matter interacts weakly with normal matter, making it invisible and elusive.
Several types of detectors are being developed to search for dark matter particles. These include:
- Cryogenic detectors that aim to measure the heat generated from potential dark matter interactions.
- Liquid noble gas detectors, like those using liquid argon, designed to capture the rare interactions of dark matter particles with atomic nuclei.
- Geiger counters in underground laboratories to minimize cosmic ray interference.
Despite these advancements, all detection methods face a challenge called the signal-to-noise ratio. Dark matter interactions are incredibly rare, and distinguishing them from background noise is very difficult. This makes confirmation of any signal almost a matter of good fortune.
Philosophical Implications of Unseen Matter
The existence of dark matter also brings up profound philosophical questions. If we consider that a significant portion of the universe consists of something that we cannot directly observe, it challenges our conceptual framework of reality.
In academia, the implications prompt discussions around several core ideas:
- Nature of knowledge: How do we define something that is fundamentally unobservable? Traditional empirical science often relies on direct observation, and dark matter poses a challenge to that.
- Existential questions: What does it mean for a significant fraction of the cosmos to be 'unknown'? The acknowledgment of unseen entities implies that our understanding is still deeply incomplete.
- Bias in interpretation: The void that dark matter fills also raises issues about anthropocentric bias in science. Are we interpreting cosmology through a human-centric lens when our tools and understanding are inherently limited?
By confronting these philosophical implications, scientists and thinkers can explore more profound meanings behind dark matter. This examination enriches the dialogue in the scientific community about the fundamental structure of reality itself.
"Dark matter may inform not only our understanding of the cosmos but also reveal the limitations of our knowledge systems."
The End
The conclusion serves as a critical reflection on the significance of understanding dark matter in the cosmos. It synthesizes the information gathered throughout the article while underlining the importance of continued exploration of this enigmatic substance. Despite the apparent invisibility of dark matter, its influence permeates many facets of modern astrophysics and cosmology.
Summary of Key Points
Several key points emerge from the analysis of dark matter.
- It is a mysterious entity that constitutes about 27% of the universe's total mass and energy, yet eludes direct detection.
- Historical developments in the understanding of dark matter highlight the evolution from early theories to contemporary models supported by substantial observational evidence.
- Various research initiatives, including experiments at the Large Hadron Collider and observations with powerful telescopes, are underway to shed light on the nature of dark matter.
- The implications of dark matter extend into cosmological models, structure formation, and potential interactions with particle physics, thereby influencing our understanding of the universe significantly.
This summary emphasizes the depth of knowledge gained and provides a coherent overview of the topic.
The Importance of Continued Research
The quest to unravel the secrets of dark matter is of paramount importance for several reasons.
- Fundamental understanding: Dark matter plays a vital role in the universe's structure and evolution. Continued research will deepen our comprehension of how galactic and cosmic systems operate.
- Technological advancements: Research initiatives drive the development of new technologies and methodologies, potentially leading to breakthroughs in other scientific fields.
- Philosophical insights: Understanding dark matter challenges our fundamental perceptions of reality and the universe. It raises significant questions about existence and what constitutes matter.
Overall, ongoing research efforts in understanding dark matter are not merely academic pursuits but foundational endeavors that could reshape our grasp of the universe.