Understanding Cellular Life: Functions and Adaptations
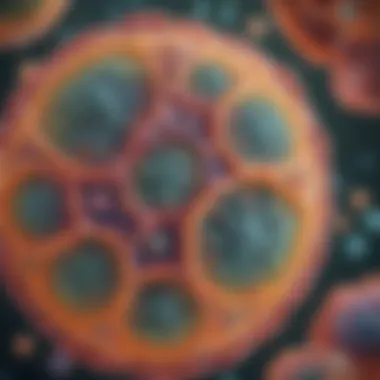
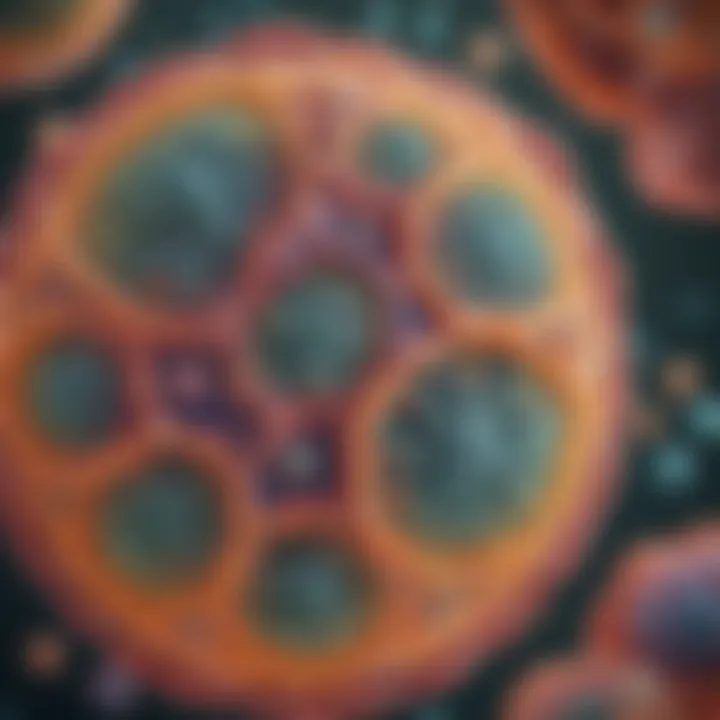
Intro
Cells are often referred to as the building blocks of life; this description is more than just a simple analogy. Each cell functions as a tiny yet sophisticated unit, carrying with it responsibilities crucial for the survival and functioning of all living creatures. In this exploration, we will peel back layers of cellular functionality to unveil the complex interactions and processes that govern life.
From the microscopic organisms thriving in extreme environments to the specialized cells that make up human tissues, the diversity within the cellular world is both remarkable and multifaceted. Understanding the lives of cells not only enriches our comprehension of biology but also enhances our insights into diseases, evolution, and future scientific innovations. This journey promises to illuminate how intimately cells are intertwined with the essence of life itself.
Key Research Findings
Overview of Recent Discoveries
Recent advancements in cell biology have opened new avenues for understanding the intricate workings of cells. Research indicates that cells are much more than static entities; they are dynamic, responding to external stimuli and signaling pathways. One striking finding is the role of stem cells in tissue regeneration, where these unspecialized cells can transform into various cell types based on the body's needs, demonstrating remarkable plasticity.
Additionally, studies on cell signaling pathways have shed light on how cells communicate. For instance, scientists have uncovered the mechanisms behind quorum sensing in bacteria, allowing us to understand how these microorganisms coordinate their behaviors based on population density, affecting everything from virulence to biofilm formation.
"To understand life at its essence is to grasp the language of cells and their ability to adapt, survive, and thrive in the face of adversity."
Significance of Findings in the Field
The implications of these discoveries are profound. Understanding cellular communication not only aids in the development of targeted therapies for diseases like cancer but also paves the way for innovations in biotechnology and genetic engineering. For instance, the emergence of CRISPR technology has stemmed from an increased understanding of cellular DNA repair mechanisms.
Moreover, the study of cellular responses to environmental changes has broad applications in fields such as climate science and agriculture. As researchers explore how cells adapt to stressors, knowledge gained could lead to enhanced crop resilience against climate change, benefiting food security on a global scale.
Breakdown of Complex Concepts
Simplification of Advanced Theories
Cellular biology can sometimes feel like a complex labyrinth, full of intricate pathways and advanced terminology. However, breaking down these concepts can help in grasping the essential ideas. For instance, the concept of the cell cycle can be simplified into phases: Interphase, where the cell prepares for division; and Mitosis, the actual division phase. Each phase has specific checkpoints, ensuring that everything operates smoothly before the cell moves onto the next stage.
Visual Aids and Infographics
To further aid understanding, visual aids such as diagrams and infographics can be incredibly useful. They reinforce learning by providing a tangible representation of cellular processes like osmosis, respiration, or signal transduction. For example, illustrating the fluid mosaic model of the cell membrane can help clarify how proteins and lipids interact within the bilayer, leading to a better understanding of membrane dynamics.
Incorporating such resources offers clarity, making the subject matter more accessible, especially for students or those who may not have a strong foundational knowledge of cellular biology.
By delving deeply into the lives of cells, we uncover a world that is foundational to our understanding of life itself. Each discovery adds a thread to the rich tapestry of cellular science, contributing to the narrative of how life functions on Earth.
Prolusion to Cellular Life
Understanding the cellular life is like embarking on a journey into the essence of existence. Every living organism, from the smallest microbe to the largest elephant, is composed of cells, making them the fundamental units of life. The significance of exploring cellular life goes beyond mere curiosity; it lays the groundwork for various scientific fields like genetics, microbiology, and medicine. This article aims to dissect the intricacies of cell functions and their significance in both microscopic and macroscopic perspectives.
Defining Cells and Their Importance
Cells are often referred to as the building blocks of life. They are the smallest units that can replicate independently. Simply put, a cell is a tiny, self-sufficient factory. It performs essential tasks that keep an organism alive; it regulates nutrient uptake, produces energy, and even communicates with other cells. Depending on the organism's needs, cells can either be simple, like those of bacteria, or complex, as seen in plant and animal cells.
The importance of cells cannot be overstated. They provide structure to living beings and facilitate all biological processes. By comprehending how cells operate, we gain insight into growth, repair, and reproduction. Additionally, cellular studies help us understand diseases at a granular level. For example, cancer cells divide uncontrollably, which alters normal bodily functions. Knowing the mechanics of cell behavior allows scientists to create targeted therapies and interventions, thus shaping modern medicine.
"Understanding cells is the first step toward understanding all life forms."
Evolutionary Perspective on Cellular Development
Cells have not always been as they are today; they have undergone substantial transformations over billions of years. This evolutionary journey began with simple prokaryotic cells, which emerged over 3.5 billion years ago. These cells lacked a nucleus and were surrounded by a cell membrane. As environmental conditions changed, so did the cells. Over time, eukaryotic cells appeared, characterized by their complex structures and organelles.
The ability to evolve into more complex forms provided eukaryotic cells a fundamental advantage. They could perform specialized functions, allowing organisms to adapt to varying environments. For instance, plant cells developed chloroplasts, enabling them to harness energy from sunlight via photosynthesis. This adaptation was crucial for life on Earth, as it contributed to the oxygenation of the atmosphere, transforming it into a hospitable environment.
In summary, studying cells from an evolutionary perspective gives us a deeper understanding of biodiversity and the connection between various life forms. It also highlights how cellular adaptations contribute to survival in diverse environments, reinforcing the intricacies of life's evolutionary tapestry.
The Architecture of Cells
Understanding the architecture of cells is crucial in grasping how life operates at a microscopic level. This structural framework shapes functionality and plays a significant role in the intricate processes that sustain life. Cells, being the basic units of life, possess unique architectures that enable them to perform various tasks efficiently. From regulating entry and exit of substances to housing organelles that specialize in different functions, the cell’s architecture is not mere decoration; it's a necessity for survival, growth, and reproduction.
Cell Membrane Dynamics
The cell membrane serves as a vital barrier that separates the interior of the cell from its environment. This dynamic structure is selectively permeable, allowing it to control what enters and exits the cell. Comprised primarily of a phospholipid bilayer, the membrane showcases a mix of lipids and proteins, forming a structure that's both flexible and resilient. It can adapt to changes in the external environment, ensuring that the cell maintains its homeostasis. One key aspect of the membrane dynamics is its ability to facilitate communication with other cells through receptor proteins. This plays a critical role in how cells respond to changes in their surroundings, underscoring the importance of the cell membrane both for protection and communication.
Organelles and Their Functions
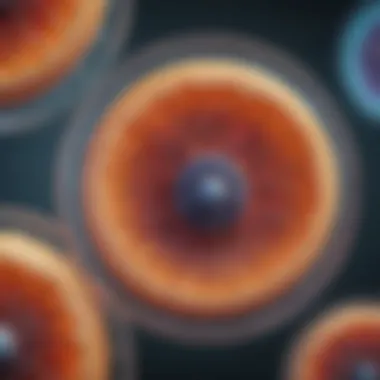
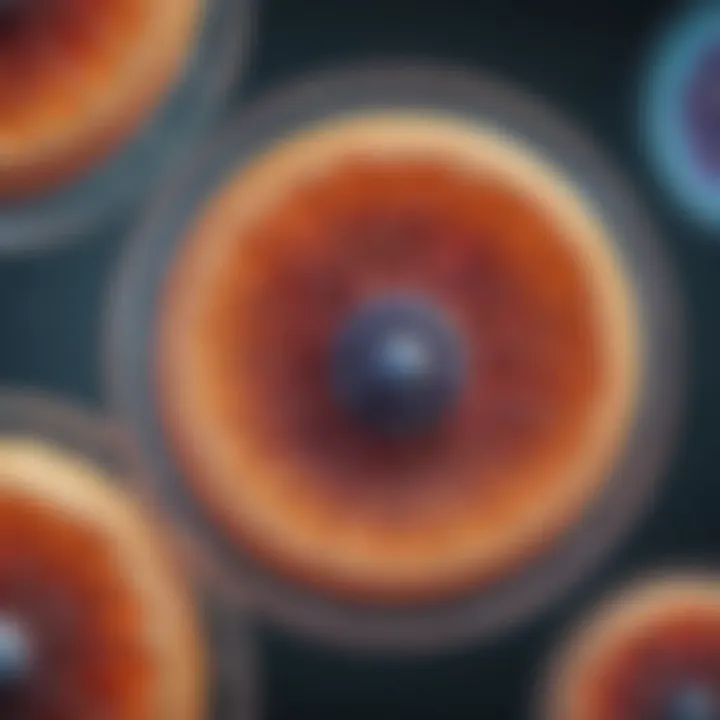
Unlike bricks in a wall, organelles are specialized structures within cells that perform distinct functions essential for cellular operations. Each organelle contributes uniquely to the overall functionality of the cell, ensuring its survival and adaptation in varied environments. Their interrelations create a finely tuned system that demonstrates the importance of cellular structure.
Mitocondria
Mitochondria are often referred to as the powerhouse of the cell for a reason. Their primary role is to generate adenosine triphosphate (ATP), the energy currency needed for numerous cellular processes. What makes mitochondria particularly fascinating is their double membrane structure, which is critical in energy production through aerobic respiration. This unique feature, combined with their ability to adapt based on the energy demands, marks mitochondria as a particularly valuable organelle in cellular architecture. Their collaboration with other organelles is vital; without them, cells would struggle to perform standard functions.
Endoplasmic Reticulum
The endoplasmic reticulum (ER) comes in two forms: rough and smooth, each serving different purposes. The rough ER is studded with ribosomes, making it essential for protein synthesis, while the smooth ER is involved in lipid production and detoxification processes. The characteristics that define the ER—its extensive network of membranes—allow it to serve as a main manufacturing hub in the cell. This versatility presents the ER as a popular choice in this article, given its central role in the production and folding of proteins, as well as in maintaining quality control within the cell. It’s a multifaceted player, critical to both cellular integrity and efficiency.
Golgi Apparatus
The Golgi apparatus functions like the post office of the cell, modifying, sorting, and packaging proteins and lipids for secretions or delivery to other organelles. This organelle’s curved structure creates a unique environment conducive to these modifications. Its role becomes especially important when considering that the cell often needs to send out signals or substances that assist in communication and transport. The intricacies of the Golgi apparatus highlight how essential it is to maintain cellular order and functionality, bolstering the case for its inclusion in our exploration of cellular architecture.
Lysosomes
Lysosomes are the cell's cleanup crew, containing enzymes that break down waste materials and cellular debris. These organelles are crucial for maintaining cellular health, as they prevent the accumulation of unwanted substances. Their ability to digest macromolecules makes them indispensable in recycling processes within the cell. A standout feature is their acidic environment, which is tailor-made for enzymatic activity. This nutritive aspect showcases the advantages of lysosomes in cellular life. However, one must also consider that improper functioning of lysosomes can lead to various diseases, marking their role as a double-edged sword in cellular functionality.
"The harmony of organelles working together showcases the elegance of cellular architecture, where each part has its role to play."
In summary, exploring the architecture of cells demonstrates how specialized structures enable a wide array of functions essential for life. The dynamics of cell membranes and the interrelated functions of organelles underscore their pivotal roles in maintaining the overall health and adaptability of cells.
Metabolic Activities of the Cell
Understanding the metabolic activities of a cell is like peeking into a bustling city, where every street (or pathway) has its specific function and purpose. Metabolism refers to the chemical reactions that occur within a cell to maintain life, providing energy and assembling the various components needed for growth and maintenance.
The importance of metabolic activities cannot be overstated. Without these processes, cells would simply cease to function, much like a car without fuel. Key benefits of metabolism include energy production, nutrient synthesis, and waste elimination, which are essential for cellular health. Metabolic activities facilitate the conversion of food into usable energy, which is necessary for all bodily functions—from the simplest muscle contractions to complex biochemical pathways.
Role of Enzymes in Cellular Metabolism
Enzymes act as catalysts in metabolic reactions, speeding up processes that would otherwise be too slow to sustain life. They lower activation energy, allowing reactions to occur at body temperature, and are highly specific, meaning that each enzyme only catalyzes a specific reaction or set of reactions.
The efficiency of enzymes means that even minimal quantities can have substantial impacts on metabolic rates. For example, consider the enzyme amylase, which catalyzes the breakdown of starches into sugars in our saliva. Without amylase, the digestion process would be laden with delays. Enzymes are thus essential for sustaining life's tempo, ensuring cells operate as smoothly as a well-oiled machine.
Cellular Respiration Explained
Cellular respiration is the process by which cells harvest energy from nutrients, primarily glucose. This is central to metabolism as it directly affects how cells generate ATP, the energy currency of the cell.
Aerobic Respiration
Aerobic respiration occurs in the presence of oxygen and yields the most energy compared to other forms of respiration. This process generates ATP through a series of steps: glycolysis, the Krebs cycle, and oxidative phosphorylation. The key characteristic of aerobic respiration is its efficiency—up to 36 ATP molecules can be produced from just one glucose molecule. This high yield makes aerobic respiration a beneficial choice for cellular metabolism, especially in energy-demanding tissues like muscles.
Another unique feature is the production of carbon dioxide and water as byproducts, which are relatively non-toxic to cells. This method of energy production showcases the intricate adaptation of cells to use available resources efficiently while minimizing waste. However, the dependency on oxygen can be seen as a potential disadvantage; cells relying solely on aerobic respiration may struggle in environments with limited oxygen availability.
Anaerobic Respiration
In contrast, anaerobic respiration occurs in the absence of oxygen and yields far less energy—typically only 2 ATP molecules per glucose molecule. This process is commonly used by certain microorganisms and in muscle cells during intense exercise when oxygen is scarce.
The key characteristic of anaerobic respiration is its speed. It allows organisms to generate energy quickly, which is vital in emergencies. Lactic acid fermentation, for instance, is utilized by muscles during short bursts of activity, providing an immediate ATP supply during challenging times.
However, the unique feature of anaerobic respiration does have drawbacks. The accumulation of lactic acid can lead to muscle fatigue and discomfort, limiting how long this method is sustainable. In a broader view, relying solely on anaerobic respiration can hinder growth and survival of cells in environments where oxygen is essential.
Photosynthesis: The Cell's Energy Source
Photosynthesis stands as a cornerstone of cellular energy production, particularly for plants and some bacteria. This process transforms sunlight into chemical energy, storing it in glucose form.
Chloroplast Functions
Chloroplasts are the organelles that house the machinery for photosynthesis. A key characteristic of chloroplast functions is their ability to absorb light energy using chlorophyll, converting it into chemical energy. This is particularly beneficial as it promotes the creation of glucose, which can then be utilized in cellular respiration or stored for later use. The unique feature of chloroplasts is their dual role: they not only provide energy to the cell but also produce oxygen as a byproduct, which is essential for aerobic organisms, creating a symbiotic relationship within ecosystems.
On the downside, the efficiency of photosynthesis can fluctuate due to various factors like light intensity, water availability, and temperature changes, impacting overall energy yield.
Light Reaction and Calvin Cycle
The photosynthesis process is primarily divided into two phases: the light-dependent reactions and the Calvin cycle. The light reactions occur in the thylakoid membranes and convert solar energy into ATP and NADPH, crucial energy carriers for the Calvin cycle, which happens in the stroma of chloroplasts.
The key characteristic of these processes is their interconnectedness; light reactions cannot happen without the Calvin cycle, and vice versa. This interplay ensures that energy is harnessed effectively. This structure allows plants to make use of sunlight efficiently, especially in optimal conditions.
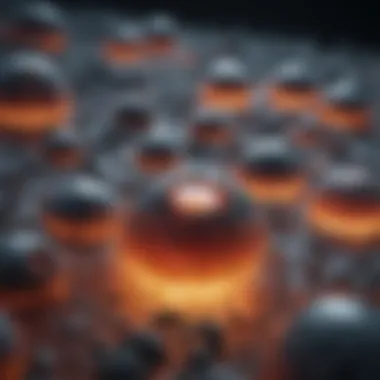
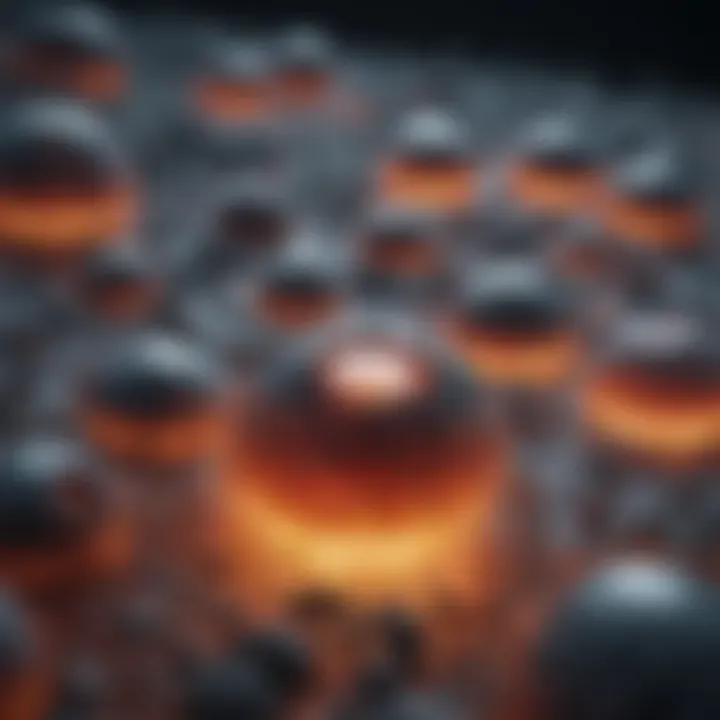
However, inefficiencies can arise when light intensity is too low or too high, causing stress on the plant and limiting overall productivity. This variable performance highlights the importance of environmental factors in photosynthesis, thus impacting not only individual cells but entire ecosystems.
Cell Communication Mechanisms
Cell communication mechanisms are pivotal to the functioning of both single-celled organisms and complex multicellular life forms. The way cells interact with one another guides essential processes such as growth, immune responses, and even behavior. These communication pathways enable the coordination of various activities, making it possible for cells to adapt to local and global changes in their environment. Without such systems, cells would operate in isolation, hindering the myriad processes that sustain life.
Signaling Pathways and Their Importance
Signaling pathways are like the intricate webs of conversation that connect the two ends of a telephone call. When a cell receives a signal, whether it's a hormone, a nutrient, or even a stress factor, it needs a means to interpret that message to react appropriately. These pathways can be characterized in stages: reception, transduction, and response.
- Reception involves the interaction between signaling molecules and receptor proteins on the cell’s surface.
- Transduction refers to the conversion of signals from one form to another within the cell, often through cascades of protein modifications.
- Response is the endgame, where changes in cellular activity take place, such as gene expression changes, cell division, or apoptosis.
Understanding signaling pathways deepens our insight into how cells communicate under normal conditions and during disease states, revealing potential targets for new treatments.
Receptor Proteins and Signal Transduction
Receptor proteins serve as the gatekeepers of cellular communication, strategically positioned on cell membranes to detect specific signals. Think of these receptors as the locks awaiting the right keys to open them. When a signaling molecule, often termed a ligand, binds to a receptor, it triggers a cascade of processes inside the cell. This mechanism of signal transduction can lead to varied outcomes, from activating specific genes to altering metabolic pathways.
- Types of receptors include G-protein coupled receptors (GPCRs) and receptor tyrosine kinases (RTKs), each having unique roles in signaling cascades.
- G-protein coupled receptors are well-known for mediating responses to hormones and neurotransmitters, influencing physiological functions like heart rate and mood.
- Receptor tyrosine kinases play a crucial role in catalyzing cellular growth, survival, and differentiation, particularly in evolving tissues.
"In cellular biology, the simplicity and sophistication intertwined in receptor function reveal nature's genius; a minimalistic design serves complex and diverse functions."
Cellular Reproduction
Cellular reproduction serves as the bedrock of life's continuity, ensuring that organisms grow, develop, and maintain their health. Without this intricate process, life, as we know it, would come to a grinding halt. It's a fascinating area that not only sheds light on how cells propagate but also underpins complex biological systems.
The importance of understanding cellular reproduction cannot be overstated. For instance, it plays a critical role in growth and development during an organism's lifecycle. Think about it: from a single fertilized egg, all the diverse cell types in our body arise through well-orchestrated cell division. Additionally, cellular reproduction is foundational for healing and repairing tissues. When you sustain an injury, it's the rapid division of cells that facilitates recovery, demonstrating the process's practicality in everyday life. Furthermore, it helps in the maintenance of genetic stability across generations, which is crucial for evolutionary fitness.
Mitosis and Meiosis: A Detailed Overview
Mitosis and meiosis, while both forms of cellular reproduction, serve different but equally essential purposes. Mitosis is the mechanism through which somatic cells divide, producing two identical daughter cells, each holding a complete set of chromosomes. This is essential for growth, repair, and replacing worn-out cells. On the flip side, meiosis is a specialized type of cell division that occurs solely in reproductive cells, leading to the creation of gametes such as sperm and eggs. This results in cells that have half the number of chromosomes, a necessity for sexual reproduction to ensure that offspring have the correct chromosome number.
The Role of Genetic Material
DNA Replication
DNA replication is a pivotal event in cellular reproduction. It refers to the process by which a cell duplicates its genetic material, allowing daughter cells to inherit a complete set of genes. The significance lies in its precision; any errors can lead to mutations that might affect the organism's viability. One key characteristic of DNA replication is its semi-conservative nature. Each new DNA molecule contains one original strand and one newly synthesized strand. This unique feature ensures high fidelity in genetic transmission. A notable advantage is that it provides a mechanism for repair - when errors occur, the cell's repair machinery can detect and fix them, maintaining genomic integrity throughout generations.
- Advantages of DNA replication:
- Ensures genetic continuity
- Allows for repair of damaged DNA
- Plays a crucial role in evolution by permitting variability through mutation
Despite its benefits, there are downsides too, particularly when replication goes awry due to external factors like radiation or chemicals, leading to potentially harmful mutations.
Cell Cycle Regulation
Cell cycle regulation orchestrates the timing and progression of cellular reproduction. It involves a series of checkpoints that ensure the integrity and readiness of the cell to divide. A critical point in this regulation is the G1 checkpoint, where the cell assesses DNA damage and nutrient availability before entering the synthesis phase. One of its strong points is that it helps prevent uncontrolled cell division, which could lead to cancer.
- Key characteristics of cell cycle regulation:
- Checkpoints manage cell cycle progression
- Involves various proteins and enzymes
- Critical for normal cell function and development
However, if these regulatory mechanisms fail, it can lead to severe consequences, such as uncontrolled cell growth and the formation of tumors. The balance they maintain is vital for health, making cellular reproduction not only a fascinating topic but also a crucial one for medical science.
"Cellular reproduction underpins all biological processes, making it a cornerstone of life itself."
Understanding these processes isn’t just for the sake of knowledge; the implications they have for medicine, genetics, and evolutionary biology are profound. With ongoing research, we can learn more about how to harness these processes in innovative ways, potentially leading to breakthroughs in healthcare and biotechnology.
Adapting to Environmental Changes
Cells are in a constant dance with their surroundings, changing and responding in myriad ways. This ability to adapt is not just a survival tactic; it’s the cornerstone of life itself. Whether it’s a simple microorganism in a pool of water or complex human tissues, how cells manage these changes significantly influences functionality and health. Understanding how cells adapt to their environments explains not only the survival of the fittest at a cellular level but also the broader implications for disease resistance, aging, and overall vitality.
Homeostasis: Maintaining Balance
Homeostasis, as the term suggests, involves the dynamic equilibrium that cells strive to maintain amidst external fluctuations. Picture a tightrope walker—cells balance various factors like temperature, pH levels, and ion concentrations to ensure their internal environment remains stable. This balancing act is crucial. For example, human blood cells adapt to shifts in oxygen levels; at high altitudes, they produce more hemoglobin to enhance oxygen uptake.
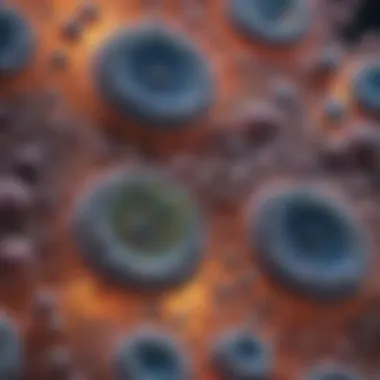
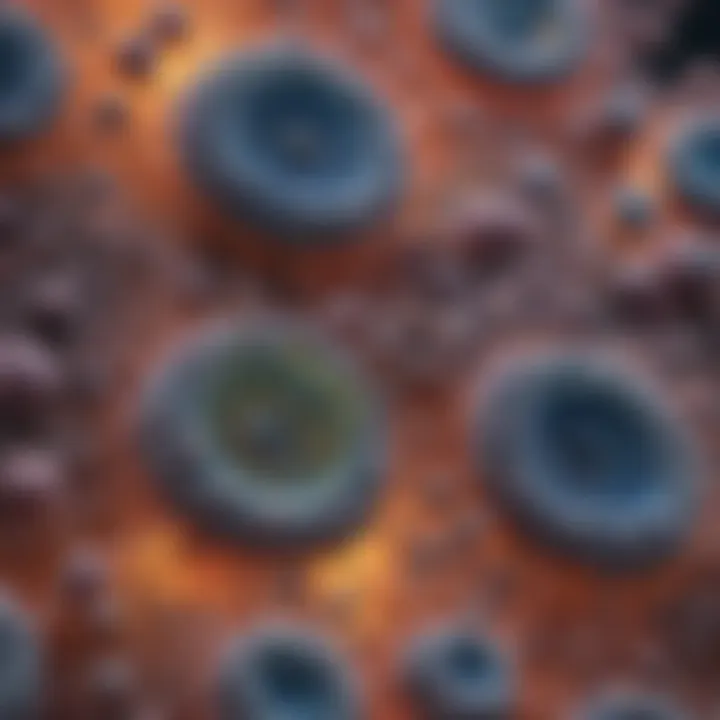
The cell membrane plays a vital role in this process by regulating what enters and exits. If conditions shift towards acidity, cells might increase the output of proteins that help neutralize acids. Yet, homeostasis isn’t static. It requires constant adjustments, employing feedback mechanisms which can be as simple as altering a few channels in the cell membrane.
- Key Elements of Homeostasis:
- Feedback mechanisms: Positive and negative feedback loops help in regulation.
- Temperature regulation: Adjusting metabolic rates to counteract environmental changes.
- Ion Homeostasis: Ensuring proper concentrations of ions like sodium and potassium.
"Cells communicate like a bustling market—each interaction a transaction that maintains homeostasis."
Cellular Response to Stress Factors
When stress occurs, whether from toxins, temperature extremes, or pathogens, cells activate stress response pathways. Think of a fire alarm blaring amidst a smoke-filled room; cells have similar alarm systems. Cellular stress responses can lead to immediate changes in function, offering a chance to recover or adapt. These pathways often involve stress proteins, commonly known as heat shock proteins, which assist in refolding damaged proteins or degrading them when they’re beyond repair.
The versatility of response allows cells to thrive under various conditions. A good case in point is plants responding to drought. They can close their stomata to reduce water loss, a process that requires signaling molecules to orchestrate a broad cellular response.
- Common stress factors include:
- Oxidative stress: Encountering excess free radicals that can damage cellular components.
- Heat shock: Encountering higher temperatures that can denature proteins.
- Pathogen attacks: Triggering immune responses at the cellular level.
In sum, the ability to adapt to environmental changes not only ensures cell survival but also intricately weaves together the complex tapestry of life. By understanding homeostasis and cellular responses to stressors, we gain insight into broader biological functions, disease mechanisms, and the implications for longevity and health.
The Interconnectedness of Cells
In the tapestry of life, cells are like threads—tightly woven, each playing a pivotal role in the greater fabric of living organisms. Understanding the interconnectedness of cells not only enlightens us about the biological processes at play but also reveals the complexity and elegance of life itself. This section dives into how cells collaborate and interact, forming an intricate network essential for the functionality of tissues and organs.
Tissues and Organs: Cells Working Together
Cells do not exist in isolation; their survival hinges on cooperation. Consider the heart—a remarkable organ composed of cardiac muscle cells, connective tissues, and specialized cells all working in harmony. Each cell type brings its unique capabilities to the table, facilitating the essential task of pumping blood throughout the body.
Key Points about Tissues and Organs:
- Cellular Cohesion: Cells adhere to each other through junctions, maintaining structural integrity.
- Extracellular Matrix: A scaffold that provides support, allowing cells to communicate and function efficiently.
- Signaling: Cells send and receive signals, telling one another when to grow, divide, or even die, ensuring appropriate responses to physiological needs.
"The whole is greater than the sum of its parts."
This phrase resonates deeply in the context of cells forming tissues and organs. An organ’s functionality transcends individual cells, manifesting a complex balance of activities; like an orchestra, every section must play in sync for the melody of life to unfold.
Here’s a closer look at different types of tissues:
- Epithelial Tissue: Forms protective barriers and is involved in absorption and secretion.
- Connective Tissue: Provides support and insulation, linking various body parts together.
- Muscle Tissue: Essential for movement, it includes skeletal, cardiac, and smooth muscles.
- Nervous Tissue: Allows communication throughout the body, processing and transmitting signals.
Cellular Specialization and Its Significance
Cellular specialization is the artist’s brushstrokes that create a masterpiece of biological function. As cells differentiate into numerous types, each specializes in particular tasks, ensuring efficiency and adaptability. Think of red blood cells, for example; they are uniquely designed to carry oxygen, equipped with hemoglobin and having no nucleus to maximize space.
- Enhanced Efficiency: Specialized cells have adaptations that enable them to perform specific roles effectively, exemplifying the division of labor in biological systems.
- Plasticity and Responses: Some cells can change functions based on environmental cues—a phenomenon crucial for healing and repair.
- Interdependence: Specialized cells often depend on one another; for instance, neurons rely on glial cells for support and maintenance.
In summary, the interconnectedness of cells through specialization, collaboration, and communication is foundational to life. Their ability to function as a cohesive unit enables organisms not only to thrive but also to adapt to ever-changing environments. This intricate network underscores the importance of cellular biology in understanding not just health and disease, but the very essence of life itself.
Ending: The Ongoing Study of Cell Lives
The journey through the intricate and multifaceted world of cellular life reveals not just the science behind life itself, but also the endless questions that continue to arise as we deepen our understanding. As we reflect on the nuances discussed in this article, it’s crucial to consider the importance of ongoing research in cellular functionality. It is this research that not only enhances our comprehension but also serves as the backbone for advancements in various scientific fields.
One significant element to highlight is that cellular studies are not static; they evolve. Modern technologies like CRISPR and advanced microscopy have opened new avenues for exploration. For instance, CRISPR-Cas9 technology has propelled genetic engineering into the limelight, allowing scientists to edit genes within organisms more precisely than ever before. This prompts new questions about ethical boundaries, potential applications in medicine, and the fundamental nature of life itself.
Furthermore, the understanding of cellular processes can lead to tangible benefits in medicine. Comprehending how cells interact with their environment aids in developing targeted therapies for diseases like cancer. Imagine a scenario where treatments are tailor-made to target the unique cellular environments of individual tumors. Such developments underscore the pressing need for continual investment in cellular research.
Here are a few reasons why ongoing study is essential:
- Innovation in Therapeutics: Advancements in targeted therapies and personalized medicine heavily rely on understanding cellular function.
- Environmental Impact: By studying how cells respond to various stressors, researchers can better address challenges related to climate change, pollution, and habitat destruction.
- Improve Agriculture: Cellular biology informs genetic modifications in crops, enhancing yields and resistance to pests while addressing food security issues.
In this landscape, collaboration across disciplines becomes increasingly important. Biologists, chemists, and physicists must work hand in hand to uncover mysteries that could lead to groundbreaking discoveries. The relevance of the ongoing study of cell lives profoundly connects with our day-to-day existence, proving that even as we encounter challenges, understanding can carve pathways towards solutions.
“Knowledge is a treasure, but practice is the key to it.”
Future Perspectives in Cellular Research
Future perspectives in cellular research suggest a wealth of opportunities waiting to unfold. Scientists are keen on tapping into the molecular underpinnings of diseases which could have significant implications for drug development. As artificial intelligence and machine learning algorithms become more sophisticated, they will likely be employed to predict cellular behavior, allowing for faster experimental iterations and breakthroughs.
Research is also trending toward studying cell-to-cell communication and how this intricate dance influences not only individual cell behavior but the health of entire tissues. Advancements in imaging techniques can help visualize these interactions in real-time, contributing to more robust predictive models for disease progression.
The Relevance of Cellular Understanding in Modern Science
The relevance of cellular understanding in modern science cannot be overstated. From health care innovations to environmental sustainability, the insights derived from cellular biology furnish foundations for numerous scientific advancements. Fields such as biotechnology benefit immensely from a profound comprehension of cell mechanisms, paving the way for revolutionary products and procedures that enhance human existence.
Ultimately, as we delve deeper into cellular life, we uncover secrets that not only answer age-old questions but also cultivate a new awareness of life's complexities. Each discovery opens doors to new inquiries, strengthening the case for supporting and investing in cellular research as a vital frontier in science.