Exploring Current Trends in Quantum Computing Research

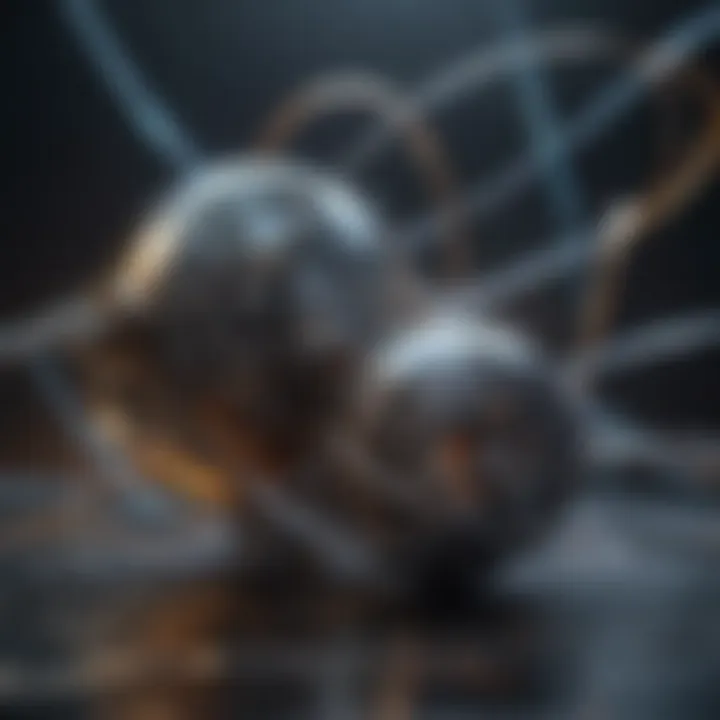
Intro
Quantum computing represents a significant shift in how we understand and manipulate data. Unlike classical computers, which process information in binary, quantum computers utilize the principles of quantum mechanics to perform calculations at unprecedented speeds. This shift is not just theoretical; it's backed by a growing body of research that explores the capabilities and potential applications of quantum systems.
As scholars and practitioners delve into the realm of quantum computing, the significance of research papers becomes increasingly evident. These documents not only present groundbreaking findings but also lay the groundwork for future innovations. In this article, we will unpack key discoveries, simplify complex concepts, and offer insights into the broader implications that these studies hold for technology and society at large.
Prelude to Quantum Computing
In today's rapidly evolving technological landscape, quantum computing stands at the forefront of innovation. This scrutiny of quantum computing is not merely academic; it has tangible implications for fields as diverse as cryptography, materials science, and artificial intelligence. By decoding the principles and advances of quantum computing, practitioners and enthusiasts alike can forecast how this technology might reshape our societal constructs and technological capabilities.
Understanding the Importance of Quantum Computing
Quantum computing is pivotal because it challenges the conventional paradigms of computation. Classical computers rely on binary bits, which signify either a zero or a one. In contrast, quantum bits, or qubits, harness the intrinsic properties of quantum mechanics, enabling them to exist in multiple states simultaneously. This extraordinary potential allows quantum computers to perform computations at speeds and efficiencies unparalleled by classical machines, particularly when addressing complex problems.
Moreover, the exploration of quantum computing paves the way for breakthroughs in handling enormous datasets. Organizations are poised to untangle intricacies in research that would take classical supercomputers eons to solve. Thus, the relevance of quantum computing ripples through various sectors, casting shadows on their operational methodologies.
Key Considerations
Several facets warrant attention:
- Complexity: Understanding the mathematical foundations of quantum mechanics can seem daunting. However, the benefits far outweigh the initial hurdles.
- Investment: The financial and intellectual investment in quantum technology is substantial, yet essential. Innovators are driven by the promise of revolutionizing computation and addressing challenges that heretofore seemed insurmountable.
- Community: The global academic and industrial communities are increasingly fostering collaborations, which bolster the growth of quantum computing research.
The exploration of quantum computing is a passage into an unparalleled world of opportunity and challenge. As we transition to the subsequent sections, we'll delve into defining quantum computing, providing clarity and contextual relevance within the vast landscape of research.
Key Research Papers and Their Contributions
The realm of quantum computing has witnessed a series of transformative research papers that have not only shaped its theoretical backbone but have also paved the way for practical applications. The significance of these papers lies in their profound impact on advancing our understanding of quantum mechanics and computation. Researchers and practitioners alike dissect these contributions to grasp their implications, whether in algorithm design, error correction, or broader computational frameworks. Understanding these key papers offers a roadmap to the collective knowledge that continues to influence developments in this field.
Foundational Papers in Quantum Theory
The journey into quantum computing necessarily begins with foundational theories. Notable research, such as John von Neumann's work on quantum mechanics and computation, lays the groundwork for how bits of information are redefined within this innovative context. Understanding the principles set forth by pioneers is essential; for instance, David Deutsch's paperβ"Quantum Theory, the Church-Turing Principle and the Universal Quantum Computer"βillustrates the first formulation of a quantum computer that could, in principle, simulate any physical process. This concept shifts the landscape of traditional computing, suggesting a much richer and nuanced perspective on computational capability.
Additionally, the work by Lov Grover on database searching and Peter Shor's algorithm for factoring integers has not only broaden the horizons of theoretical algorithms but also significantly raised expectations for what quantum computers might achieve. Researchers consistently reference these seminal papers, which provide the framework for exploring further innovations in quantum computation, showcasing a model where quantum mechanics does not just complement but enhances computational processes.
Papers Advancing Quantum Algorithms
As exploration in quantum computing proceeds, advancing quantum algorithms becomes paramount. Research papers from experts such as Peter Shor and Lov Grover are critically important as they detail fundamentally new algorithms capable of solving problems exponentially faster than classical approaches. Shorβs algorithm demonstrates factoring large integers, which has profound implications for secure communications and cryptography; this paper has turned heads in cybersecurity circles and beyond. Lov Groverβs algorithm, similarly, revolutionizes search tasks by presenting a quadratic speed-up over classical counterparts.
These algorithms introduce concepts that alter how computations are performed, ultimately enhancing efficiency and enabling solutions to complex problems that were previously deemed intractable. The growing body of work in quantum algorithms emphasizes not just theoretical validation but also practical implications, highlighting areas ripe for exploration and innovation. With every new algorithm developed, the potential applications stretch across sectors, from pharmaceuticals to complex systems optimization.
Recent Breakthroughs in Quantum Error Correction
In the pursuit of making quantum systems viable for widespread application, error correction emerges as a critical focus area. Recent research papers have made strides in addressing the fragile nature of quantum information, particularly the challenge of decoherence. Notable contributions include the surface code presented by Peter Shor and later refined by others, allowing for significant error rate leverage without sacrificing too much inefficiency in the quantum computation process.
As quantum computing platforms evolve, the importance of error correction cannot be overstated. It's the backbone that facilitates the reliable execution of quantum algorithms, enabling longer and more complex operations to be performed. Innovations in this area are often met with leaning looks from the community, eager for robust solutions to long-standing issues. These breakthroughs have thus opened new avenues in quantum hardware and software, steering the research landscape closer to realizing practical, scalable quantum computers.
The advancements in quantum error correction are vital. Without it, the promise of quantum computing canβt be realized.
Understanding Quantum Bits and their Functionality
In order to grasp the nuances of quantum computing, understanding quantum bits, or qubits, serves as a crucial cornerstone. Unlike classical bits that simply sit in a state of either 0 or 1, qubits introduce a new level of complexity and potential. This section delves into the conception of qubits, drawing distinctions between them and classical bits, highlighting their importance, and exploring how they underpin the very fabric of quantum computations.
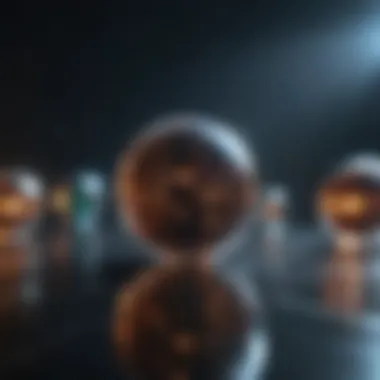
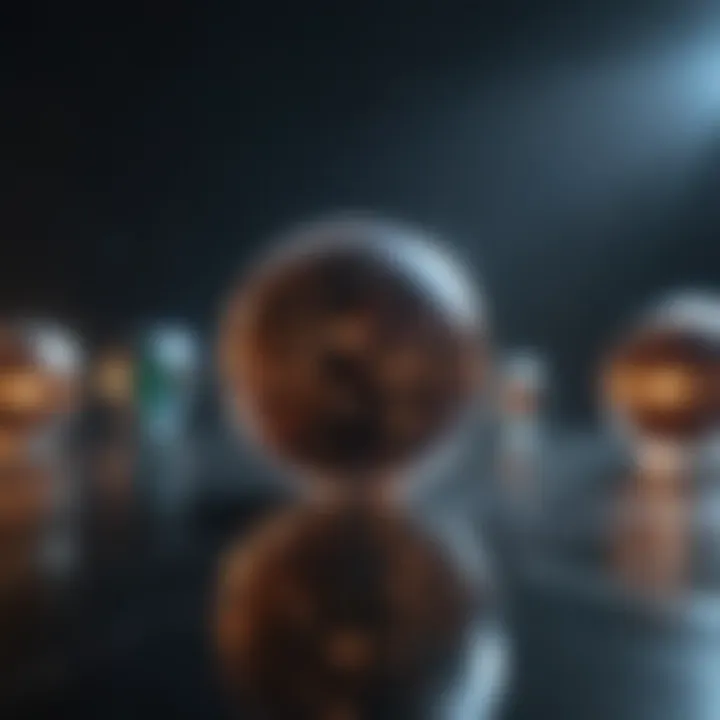
The Concept of Qubits
A qubit functions as the basic unit of quantum information. It straddles both the classical domains of computing with intriguing characteristics that defy conventional logic. Central to the definition of a qubit is its ability to exist in a superposition of states. This means that a qubit can represent 0, 1, or any quantum superposition of both simultaneously. This property not only enhances computational capacity but also underpins quantum parallelismβthe notion that quantum computers can process vast amounts of data tirelessly.
When we think of qubits, we think of the dual phenomenon of superposition and entanglement. Entangled qubits exhibit a phenomenon where the state of one qubit cannot be described independently of the state of another, no matter how far apart they are. This interlinked behavior facilitates a level of interconnected calculations that forge pathways toward solutions that classical computers could only dream of. It's like having a team of experts who are not just individually intelligent, but whose brains are wired togetherβresulting in a collective intelligence that's exponentially greater.
Comparisons with Classical Bits
Now hereβs where things start to color outside the lines. Classical bits are the old-school heroes of computing, represented clearly as either a 0 or a 1. All traditional computing relies on bits arranged in a series of on/off switches. In contrast, qubits represent a radical departure from this binary system.
- Complexity: While a classical bit encompasses a single state, qubits can weave multiple states into the fabric of computations. For example, two classical bits can represent four possible states (00, 01, 10, 11), while two qubits can cover an entire superposition of states, which is actually four in a single unit of computation. This leads to an exponential increase in the amount of information stored and processed.
- Processing Power: The superposition enables a quantum computer to explore numerous solutions concurrently, making it vastly more powerful for certain problems, such as factoring large numbers or simulating quantum systems, relative to classical counterparts.
- Error Rates: Despite their enormous potential, qubits come with the challenge of decoherence. Classical bits are stable and can maintain their state indefinitely. Qubits, vulnerable to environmental interferences, lose their quantum properties relatively swiftly, making error correction an essential consideration in quantum computing.
- Implementation: Classical bits can be realized through physical mechanisms like transistors, while qubits can be implemented through varied means, including trapped ions, superconducting circuits, and photon polarization. Each method brings its own set of advantages and challenges.
"As we navigate the complex narrative of quantum bit functionality, an understanding becomes apparent: the stakes are high, and the realm of possibilities is virtually limitless."
In wrapping up this section, itβs evident that qubits stand as a linchpin in the architecture of quantum computing. Their unique characteristics set the stage for advances that classical computing simply cannot match. This conceptual understanding will be critical as we analyze deeper into methodologies, applications, and challenges awaiting in the evolving landscape of quantum research.
Methodologies in Quantum Computing Research
Understanding the methodologies in quantum computing research is vital in capturing the essence of how breakthroughs are achieved in this profound field. Researchers employ various approaches that help bridge theoretical concepts with practical applications. This section highlights the pivotal methodologies that shape the discovery and innovation landscape in quantum computing.
Simulations in Quantum Research
Simulations play a crucial role in quantum computing research, acting as a sandbox for testing hypotheses without the need for physical experimentation. They allow theoreticians and practitioners to model quantum systems that might be impractical or impossible to observe directly. For instance, researchers can simulate quantum algorithms to analyze their efficacy or visualize quantum entanglement scenarios, which are often outside the grasp of classical computation.
Some benefits of using simulations include:
- Cost Efficiency: Running virtual experiments is often less resource-intensive than building hardware.
- Flexibility: Simulations can accommodate various parameters, allowing rapid iteration and experimentation.
- Scalability: While physical systems may struggle with scaling up, simulations can simulate larger quantum systems without significant increases in complexity.
However, simulations aren't without their challenges. Limitations arise from the assumptions integrated into models, which might not always accurately reflect reality. Furthermore, as quantum systems increase in size, computational complexity may outpace classical computing capabilities, drifting closer to the limits of available hardware.
"Simulations act as the testing ground for quantum theories, shaping how we translate abstract ideas into concrete advancements."
Experimental Approaches
On the flip side, experimental methodologies fuel progress in the quantum realm by providing empirical foundations upon which theories are built. Experimentation is critical for validating the predicted behaviors of qubits, algorithms, and quantum circuits. Noteworthy techniques include:
- Ion Trapping: In this method, charged particles are confined using electromagnetic fields, allowing precise control over their quantum state. This approach has led to significant advancements in quantum gates, showcasing real-time manipulation and measurement of information.
- Superconducting Qubits: Many research groups have turned to superconducting circuits due to their potential to operate at higher speeds than other qubit implementations. This experimental setup brings us closer to scalable quantum computation, as seen in Googleβs 2019 announcement regarding their quantum supremacy achievement.
Conducting experiments in quantum computing often involves intricate setups that require a deep understanding of quantum mechanics to interpret results accurately. Furthermore, researchers must also grapple with decoherenceβwhere quantum states lose their special properties due to interactions with the environmentβcomplicating the extraction of useful data from experimental results.
Researchers are constantly refining these experimental methodologies to overcome obstacles. The collaboration between simulation and experimentation paves the way for a more cohesive understanding of quantum phenomena while steering the course for future innovations in the field.
Challenges in Quantum Computing
Quantum computing holds substantial potential, yet it faces several noteworthy obstacles that could significantly impact its development and implementation. These challenges are not trivial; they embody fundamental aspects that researchers must navigate to enable practical quantum systems. This section examines the primary difficulties that the quantum computing community currently grapples with, emphasizing the importance of addressing these issues for future advancements.
Scalability Issues
Scalability remains a critical hurdle in quantum computing. Traditional computing systems can be scaled relatively easily, simply by adding more classical bits. In contrast, quantum systems require increasing numbers of qubits, each operating in accordance with the peculiar principles of quantum mechanics. The primary challenge arises from the nature of qubits themselves.
- Interconnectivity: Each qubit requires precise control and communication with others. As the number of qubits increases, maintaining quality interactions becomes increasingly complex. It can lead to a situation where errors cluster, undermining the systemβs performance.
- Resource Intensive: Making a quantum computer larger doesn't just mean throwing on more qubits; it also means managing the qubits' cryogenic temperatures, shielding against noise, and providing robust error correction mechanismsβall tasks that can escalate resource requirements rapidly.
- Physical Limitations: Engineers are also hampered by material science challenges. Many current quantum hardware implementations, such as superconducting qubits or trapped ions, face intrinsic physical limitations that prevent seamless scalability.
To summarize, the scalability issue in quantum computing encapsulates a myriad of factors that intertwine physical limitations, complex interaction requirements, and substantial resource demands. If these challenges are not met, quantum cognition may remain a mere theoretical exercise, rather than a revolution in computational capabilities.
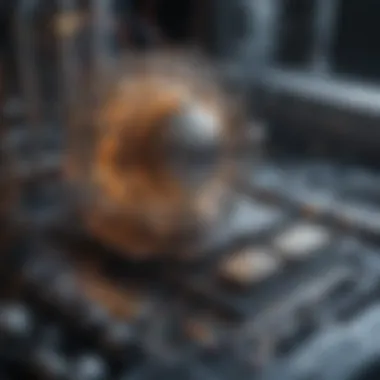
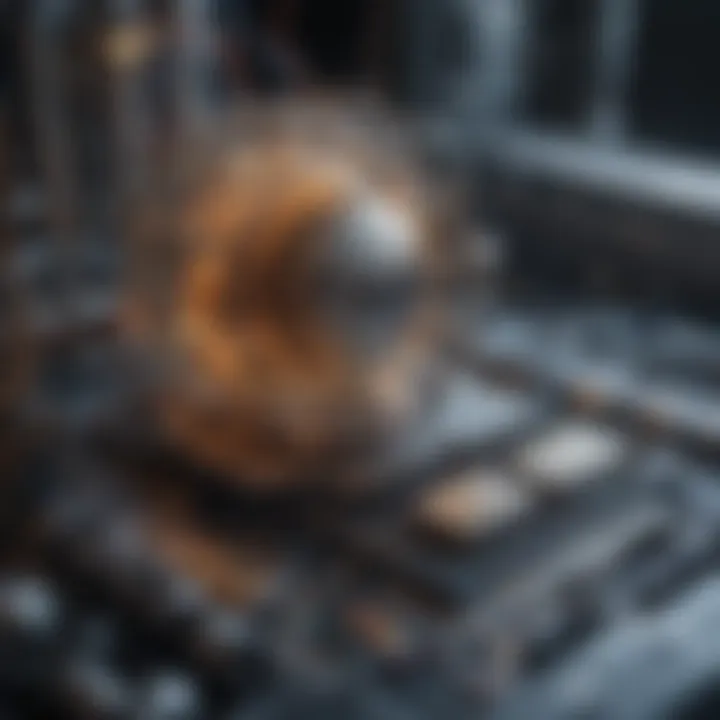
Maintaining Quantum Coherence
Another daunting challenge in quantum computing is maintaining quantum coherence. Coherence refers to the ability of qubits to maintain their quantum state long enough to perform computations. Unfortunately, qubits are notoriously sensitive to their surroundings; any disturbance can lead to decoherence, which effectively collapses their quantum state.
- Environmental Interaction: Qubits constantly interact with their environmentβbe it electromagnetic radiation or thermal noise. These interactions can introduce errors, as state transitions could mimic computational operations, throwing a wrench into calculations.
- Time-Sensitive Operations: The window of time available for coherent operations is often minuscule. Researchers must design quantum algorithms to exploit these brief moments of stability, which often leaves little room for error.
- Error Correction Challenges: While theoretical error correction methods exist, they often require a significant overhead in additional qubits to protect against errors. This overhead can complicate system designs even further, with coherence still taking precedence.
"The leap from classical to quantum is not merely an upgrade; itβs navigating uncharted waters where coherence and scalability are the twin currents guiding our journey."
The exploration of these challenges illuminates the developmental landscape that quantum researchers must traverse to unlock the full potential of quantum technologies. Without overcoming these issues, the promise of quantum computing remains insubstantial.
Applications of Quantum Computing
In the rapidly evolving field of quantum computing, the applications of this technology form a crucial part of its narrative. These applications not only demonstrate the potential of quantum algorithms but also pave the way for transformative changes across various sectors. From security to data analysis, understanding these real-world implementations illuminates the vast landscape of possibilities that quantum computing offers.
Quantum Cryptography
One of the most compelling applications of quantum technology is quantum cryptography, particularly quantum key distribution (QKD). At its core, QKD leverages the principles of quantum mechanics to facilitate secure communication. Unlike classical techniques, QKD ensures that any eavesdropping attempts reveal themselves, creating a level of security unmatched by traditional encryption methods.
- Benefits of Quantum Cryptography:
- Unbreakable security protocols: Based on quantum physics, it significantly raises the stakes for information breach attempts.
- Practical implementations: Countries like China have already deployed satellites utilizing QKD, demonstrating real-world applicability.
- Future-proof: As computational power increases, quantum cryptography remains resilient against advances in conventional hacking techniques.
As organizations increasingly face cybersecurity threats, the drive to adopt quantum cryptography becomes imperative. The capacity to safeguard sensitive data against future challenges makes this area not just relevant but vital.
Quantum Machine Learning
The intersection of quantum computing and machine learning is another fertile ground for research. Quantum machine learning (QML) extends classical algorithms to quantum systems, potentially achieving significant speedups over their classical counterparts. Here, the concepts of superposition and entanglement play pivotal roles, allowing for handling larger datasets and deriving insights that classical computers struggle with.
- Key considerations for Quantum Machine Learning:
- Speed: QML algorithms like quantum support vector machines can run faster than classical ones.
- Enhanced data handling: Quantum states allow encoding more information than classical bits, improving analysis efficiency.
- Novel algorithms: Approaches such as quantum-inspired algorithms are being developed, merging traditional machine learning with quantum techniques.
Students and researchers in data science should keep a close eye on developments in QML as they could redefine standards for tackling complex problems ranging from image recognition to natural language processing.
Optimizing Complex Systems
Quantum computing is particularly well-suited for optimization problems, where the goal is to find the best solution from a huge set of possibilities. Industries such as logistics, finance, and pharmaceuticals are already exploring quantum optimization techniques to streamline operations and enhance decision-making processes.
- Specific applications in complex systems include:
- Supply chain optimization: Quantum techniques can handle logistical challenges in more efficient ways than classical methods.
- Financial modeling: Simulation of various financial scenarios can be achieved with higher precision and fewer resources.
- Drug discovery: The intricate relationships between molecular structures can be efficiently modeled and analyzed, speeding up the discovery process.
"The real trick to using quantum computing effectively is knowing which problems to apply it to."
The potential of quantum computing to optimize complex systems highlights its transformative role in contemporary technology. As research progresses, it will clear hurdles that have long hindered advancements in various sectors. The ramifications of these applications are immense, making them essential to the ongoing dialogues in both technology and societal contexts.
The Future of Quantum Computing Research
The field of quantum computing stands at a fascinating crossroads, where the potential for groundbreaking advancements aligns with the intricacies of theoretical and applied research. Understanding the future of quantum computing research is crucial for grasping how it might reshape technology, daily life, and problem-solving approaches in various sectors. Research in this area holds promise not just for theoretical understanding, but for practical applications that could revolutionize industries like cryptography, materials science, and artificial intelligence.
The driving forces behind the future of this domain are multiple. For one, as more institutions commit significant resources, both financially and intellectually, the advancements are likely to become more rapid and inspiring. Indeed, emerging trends hint at exciting possibilities that could transform current technologies into something unrecognizable, paving the way for innovations we can only dream of today.
Emerging Directions
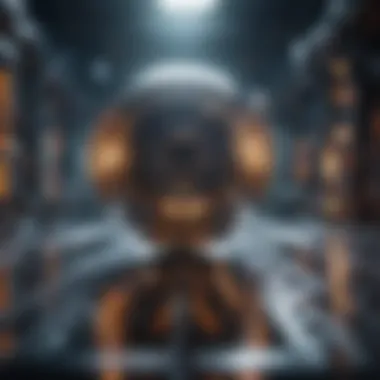
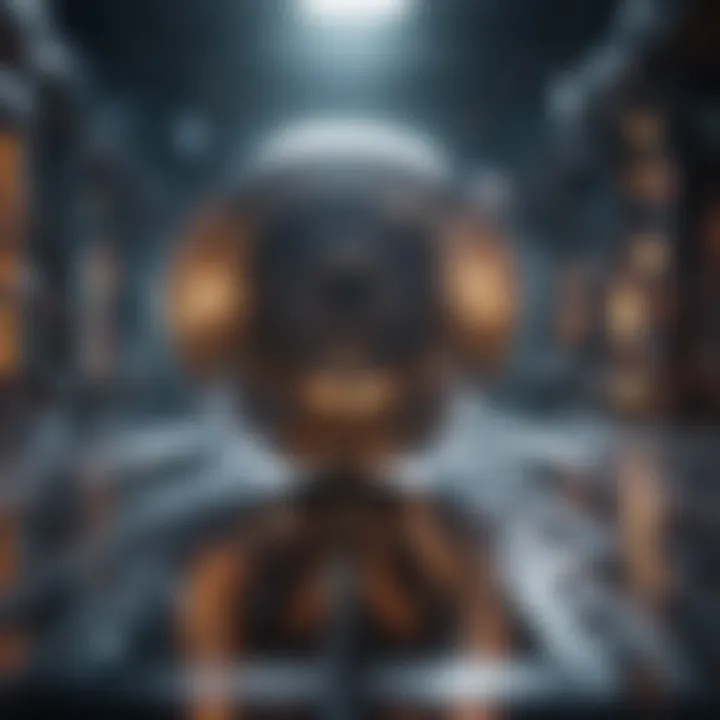
Several emerging directions stand out when contemplating the future of quantum computing research. One vital area is the exploration of quantum networking. This involves creating interconnected quantum systems capable of sharing information in ways classical networks cannot, potentially revolutionizing communication security and efficiency. Going beyond that, researchers are working on developing quantum supremacyβthe point at which quantum computers perform computations beyond the reach of classical computers. Achieving this milestone would not only mark a significant leap in computational power but also validate the theories that underlie quantum mechanics.
Another notable direction includes the integration of quantum algorithms tailored for specific problems, like optimization issues and complex simulations. By focusing these algorithms on real-world applications, researchers are not just broadening the theoretical frameworks but also ensuring tangible impacts on various fields. This blend of theoretical exploration and practical application speaks volumes about the future trajectory of quantum research.
Moreover, the rise of hybrid computing models epitomizes future potential. Combining quantum and classical systems could lead to enhanced problem-solving capabilities, optimally leveraging the strengths of each. As such technologies advance, an interdisciplinary approach will become crucial.
Interdisciplinary Approaches
Interdisciplinary approaches are essential for the future of quantum computing research. The convergence of fields such as computer science, physics, and even philosophy will shed light on various challenges and opportunities in quantum research. Diverse teams can help tackle complex questions that a single discipline may not easily resolve. For instance, merging insights from mathematics and quantum physics can lead to novel algorithm designs that surpass existing methods.
Contributions from materials science are also significant. The development of better superconductors, for instance, can lead to more efficient qubits, thus enhancing the performance of quantum computers. Understanding the physical limits of materials used in quantum computing setups requires significant collaboration between physicists and materials scientists.
On another front, the ethical implications of quantum technologies must not be overlooked. Discussions among ethicists, technologists, and policymakers can guide responsible advancements, ensuring that the benefits of quantum computing can be enjoyed widely without detrimental societal implications.
βThe future of quantum computing isn't just about computational power; itβs about interdisciplinary collaboration that will shape our understanding of both technology and society.β
All in all, considering these emerging directions and interdisciplinary approaches is critical. They promise not just growth within quantum computing itself but have the potential to trigger changes across various industries. Whether we are tackling complex calculations or addressing real-world challenges like climate modeling and drug discovery, the future trajectory of quantum computing research holds a treasure trove of possibilities.
Quantifying the Impact of Research Papers
In the realm of quantum computing, quantifying the impact of research papers serves as a cornerstone for understanding how theories and applications evolve. The significance of this topic stretches beyond mere numbers or citations. It reveals the depth of innovation, the direction of future explorations, and the practical implications of research. Essentially, it gauges how these contributions mold the landscape of technology and society at large.
The essence of quantifying research impact lies in its ability to offer tangible metrics that foster insights and drive decisions. This process is multifaceted: it encompasses citation analysis, which tracks how often a paper is referenced in new studies, and an examination of influence on policy and industry. Both these aspects shed light on the scholarly ecosystem, ensuring that pioneering ideas receive the recognition they deserve.
Citation Analysis
Citation analysis acts like a barometer for measuring the prominence of a research paper within the academic community. It's not just about counting references; it's about evaluating the quality of those citations. High citation counts often suggest that a paper has provided foundational insights or novel approaches, making it a reference point for subsequent studies.
However, a closer look at citation networks can reveal additional layers of meaning. For instance, if a paper is frequently cited in interdisciplinary works, it may indicate its relevance beyond its initial field, suggesting a broader impact on areas such as artificial intelligence or computational theory.
- Key Factors in Citation Analysis:
- H-index: An authorβs h-index can reflect productivity and impact, indicating how often their work is cited.
- Citation Velocity: The rate at which a paper is cited over time can signal a surge of interest or a paradigmatic shift in research focus.
- Context Matters: Understanding the context in which a study has been cited is crucial. Was it to support a hypothesis, or was it critiqued by others? This distinction provides valuable insights.
Using citation analysis allows researchers to pinpoint influential papers, guiding future research directions effectively.
Influence on Policy and Industry
The impact of quantum computing research papers isn't resigned solely to academia; it spills into the realms of policy-making and industry practices. When groundbreaking ideas emerge, they can inspire the creation of frameworks that govern technological advancements. For example, a study elucidating quantum encryption could lead to new regulations around data security practices in various sectors.
- Policy Influence:
- Industry Influence:
- Informing Legislation: As researchers publish papers on quantum computing's potential societal implications, policymakers may craft legislation that fosters innovation while minimizing risks.
- Funding Directions: Research findings can influence government and private sector funding priorities, directing resources toward promising areas of quantum research.
- Guiding Company Strategies: When prominent research highlights effective quantum algorithms, companies might pivot their development strategies, aligning their products with these new insights.
- Encouraging Collaboration: The dissemination of impactful research findings can foster partnerships between institutions and businesses, enabling them to leverage each otherβs strengths.
The integration of research papers into the policy and business landscapes illustrates a symbiotic relationship. It underlines how quantum computing research does not exist in a vacuum but actively participates in shaping future technological advancements.
Epilogue
As we draw the curtain on this exploration into quantum computing research papers, itβs clear that the relevance of this topic cannot be overstated. Quantum computing stands at the crossroads of theoretical investigation and practical applications, presenting a unique landscape that continues to evolve.
Summarizing the Key Takeaways
In looking back through the myriad contributions of significant research papers, several key points emerge:
- Interconnectedness of Knowledge: The journey through quantum research is not isolated. Each paper builds upon the foundation laid by its predecessors, creating a rich tapestry of intertwined ideas and innovations. Understanding this connection allows students and professionals alike to appreciate both the historical context and future trajectory of the field.
- Diversity of Methodologies: The methodologies in quantum computing are as varied as they are fascinating. From theoretical models to simulations and physical implementations, the approaches to research are diverse. This variety not only fuels creativity but also fosters resilience in tackling challenges faced in quantum systems.
- Real-World Applications: Many of the discussed research papers emphasize practical applications. Fields such as cryptography and machine learning stand to benefit immensely. This highlights the enormous potential of quantum computing to revolutionize industries and influence societal structures.
- Navigating Challenges: It's imperative to acknowledge the barriers present in quantum computing. Scalability and maintaining coherence are prevalent issues that require innovative solutionsβthese challenges often remain unsolved, spurring further research.
- The Future: With the pace of advancements in quantum computing, staying abreast of emerging trends and interdisciplinary collaborations is essential. Good researchers should continuously engage with the field, building skills while anticipating future developments.
It's worth reflecting that the implications of quantum computing extend beyond technicalities; they resonate with philosophical questions about computation, information, and even humanity itself. In sum, the journey through quantum research papers reveals a landscape rich with insight, challenges, and opportunities that not only shape the realm of computing but also possess the potential to influence wider societal paradigms.