The Formation of Earth: A Detailed Exploration
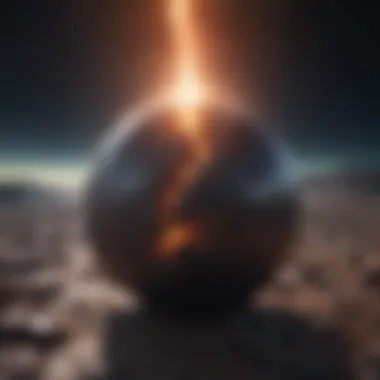
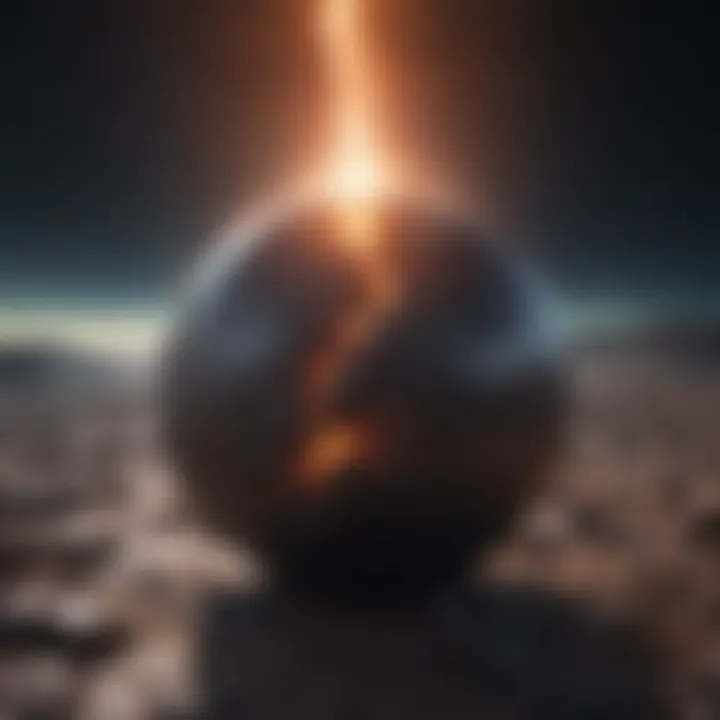
Intro
The formation of Earth is a complex tale woven through billions of years, revealing the intricate processes that have led to the planet we know today. This detailed examination begins with the concept of planetary accretion, where dust and gas in the primordial solar nebula came together, forming larger bodies through gravitational attraction. The journey from a chaotic mass of matter to a stable environment capable of sustaining life involved several critical stages. To appreciate this transformation, it is necessary to analyze various theories and scrutinize geological evidence.
In exploring Earth’s formation, we not only uncover the story of our planet but also gain insights into broader astrophysical processes that govern other celestial bodies in the universe. Understanding these phenomena sheds light on the conditions that may exist on exoplanets and enhances our knowledge of the universe's evolution.
Key Research Findings
Research in recent decades has significantly advanced our knowledge of Earth’s formation. Groundbreaking discoveries in planetary science and geology illuminate various aspects of this process.
- Overview of Recent Discoveries
Recent studies utilizing advanced telescopes and space probes have provided valuable data about the early solar system. They indicate that Earth formed about 4.5 billion years ago from the accretion of material in the solar nebula, closely resembling other terrestrial planets like Mars and Venus. - Significance of Findings in the Field
The insights gained are not just theoretical; they have practical implications for our understanding of planet formation. For instance, knowing the processes that led to Earth’s differentiation informs the search for potentially habitable exoplanets. Furthermore, the role of water and its delivery through impacts during the late heavy bombardment offers important clues about the origin of life.
- The analysis of isotopic ratios in ancient meteorites has provided clues to the conditions present during the formation phase.
- Instruments aboard missions such as the Mars rover Perseverance have opened new avenues for understanding planetary formation by studying Martian geology and comparing it with Earth.
"Understanding the formation of Earth is akin to unwinding a cosmic mystery that reveals the intricate dance of matter and energy over millions of years."
Breakdown of Complex Concepts
The journey of Earth's formation encompasses several intricate processes that can be challenging to grasp. This section aims to simplify these theories for better understanding.
- Simplification of Advanced Theories
- Visual Aids and Infographics
Diagrams illustrating the stages of planetary formation can provide clarity. Although the aim here is not to include images, one may visualize the accretion process and subsequent differentiation through structured flowcharts in their research.
- Planetary Accretion: This is the process where small particles collide and stick together, gradually building up larger bodies.
- Differentiation: Post-formation, Earth underwent a stage where denser materials sank to create a core, while lighter materials formed the crust and mantle.
The formation of Earth is a subject of extensive study with many implications. By synthesizing diverse research findings, this narrative sets a foundational understanding that links Earth's geological history with broader cosmic events, making it a topic of profound significance.
Foreword to Planetary Formation
The study of planetary formation is essential for understanding our own planet, Earth. This process reveals how elements from the cosmos gather and evolve into complex bodies capable of supporting life. It encompasses a multitude of scientific disciplines including astrophysics, geochemistry, and environmental science. An in-depth exploration of planetary formation helps clarify the forces and conditions that shaped Earth, providing a context for contemporary geological and biological phenomena.
Defining Planetary Formation
Planetary formation refers to the series of stages through which dust and gas in space conglomerate to form planets. This begins in a protoplanetary disk, where particles collide, stick together, and gradually build larger and larger bodies. Understanding this process involves recognizing several key stages:
- Nebula Collapse: Initially, a cloud of gas and dust begins to collapse under its own gravity.
- Formation of the Protoplanetary Disk: As the nebula collapses, it spins and flattens into a disk.
- Accretion of Planetary Bodies: Within this disk, small particles collide and stick together to form larger bodies known as planetesimals.
- Formation of Planets: These planetesimals continue to collide and merge, forming protoplanets and eventually full-sized planets like Earth.
Each of these stages reflects a distinct interplay of physical forces and varies based on the specific conditions of the forming planetary system. This foundational knowledge sets the stage for examining the particulars of Earth's formation.
Historical Perspectives on Earth’s Origin
The quest to understand how Earth formed has evolved significantly over centuries. Early theories were primarily philosophical and mythological, lacking empirical validation. With advancements in observational astronomy and geology, however, the narrative shifted toward scientific inquiry.
- Ancient Beliefs: Civilizations such as the Greeks proposed ideas about a primordial earth, influenced heavily by mythology.
- Scientific Revolution: During the 17th century, figures like Galileo began to employ systematic observation, marking a pivotal shift toward scientific reasoning.
- 19th Century Theories: The acceptance of an old Earth altered perspectives. Charles Lyell and Charles Darwin provided frameworks for understanding long processes of geological change, setting the stage for later ideas about planetary formation.
The culmination of these historical perspectives led to more modern theories, including the Nebular Hypothesis, which remains a cornerstone in planetary formation studies today. Understanding these historical contexts enhances grasp of current scientific discussions and fosters deeper insight into future research paths.
The Solar System’s Birth
The birth of our solar system represents a pivotal event in the field of planetary science. Understanding its formation is essential for comprehending the intricate dynamics that led to Earth’s development as a life-supporting planet. The solar system’s birth involved several crucial processes that shaped not only Earth but the entire celestial neighborhood, including planets, moons, and smaller bodies like asteroids and comets.
The Nebular Hypothesis
The Nebular Hypothesis serves as the cornerstone theory explaining how our solar system formed. According to this theory, the solar system emerged from the gravitational collapse of a large molecular cloud. This cloud, composed mostly of hydrogen and helium, began to contract under its own gravity, leading to the formation of a rotating disk.
This disk is significant because it allowed for the concentration of mass in the center to create the Sun, while the peripheral material eventually formed the planets. The process was not immediate; it unfolded over millions of years. As the material in the disk cooled, solid particles began to condense.
Key aspects of the Nebular Hypothesis include:
- Formation of the Sun: At the center of the collapsing cloud, nuclear fusion initiated, giving birth to our Sun.
- Planetary Formation: Out of the disk formed around the Sun, dust and gas coalesced into larger bodies known as planetesimals, which collided, merged, and grew into the planets we recognize today.
- Presence of Planetesimals: The interactions between these small bodies help explain the differences between terrestrial planets like Earth and gas giants like Jupiter.
A comprehensive understanding of the Nebular Hypothesis sheds light on not only the nature of our solar system but also similar systems observed in distant regions of our galaxy. Further study can refine this model, particularly through the application of modern astrophysical techniques.
The Role of Gravity in Accretion
Gravity plays a critical role in the proces of accretion through which planets form. As material clumped together, gravitational forces increased, contributing to the attraction of neighboring particles. This attraction led to a cascade of collisions among planetesimals, gradually building larger bodies.
The gravitational pull also ensures that denser materials sink toward the center of formed bodies. This process significantly affected the internal structure of the planets, including Earth. For instance, metals migrated to the core while lighter materials formed the mantle and crust.
Understanding the role of gravity includes:
- Gravitational Interactions: The influence of gravity results in the clustering of matter and the formation of larger celestial objects.
- Collision Dynamics: With each collision, energy was exchanged, allowing for heating and eventual melting, which is crucial for processes like differentiation.
- Formation of Orbital Patterns: The resulting gravitational interactions established stable orbits for planets, which defines their interactions in the solar system.
The principle of gravity is fundamental to understanding the dynamics of planetary formation, as it dictates how materials come together to form larger bodies.
In summary, the solar system's birth encompasses a framework within which dynamic processes shaped Earth and our other planetary neighbors, emphasizing the significance of gravity and the mechanisms proposed in the Nebular Hypothesis.
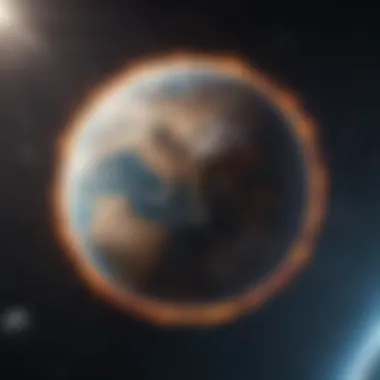
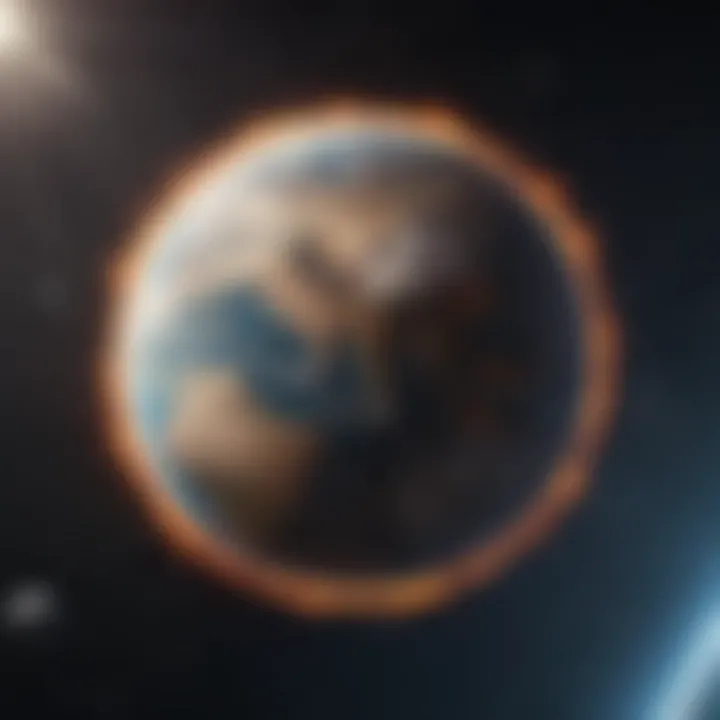
Formation of Protostar and Protoplanetary Disk
The initial stages of stellar and planetary formation are pivotal in understanding how Earth came into existence. The concept of a protostar and a protoplanetary disk play crucial roles in this narrative. These processes not only mark the beginning of the life cycle of stars but also establish the building blocks for planetary systems like ours. The protostar phase is characterized by the gravitational collapse of a region within a molecular cloud, leading to the accumulation of mass and energy. The surrounding protoplanetary disk subsequently forms, which is critical in the accretion of material that eventually coalesces into planets.
The formation of these structures highlights the dynamic and chaotic nature of cosmic phenomena. Studying the protostar and its associated disk offers insights into the fundamental physical processes that shaped early Earth. An understanding of these processes brings clarity to how diverse elements and compounds become available for the formation of terrestrial bodies. Moreover, analyzing these components expands our comprehension of the conditions necessary for life.
Characteristics of the Protoplanetary Disk
The protoplanetary disk, primarily composed of gas and dust, possesses distinct characteristics that are vital for planetary formation. This structure typically appears in a flattened disk shape around a nascent star. Key features of such disks include their temperature gradients and compositional variations. The inner regions of the disk are hotter, favoring the accumulation of metals and silicates, while the cooler outer regions allow for the formation of ices and other volatiles.
Pressure and temperature variations within the disk lead to differing elemental distributions, forming the foundation for diverse planetary compositions.
- Dust Particles: Microscopic particles that collide and stick together.
- Gas: Mainly hydrogen and helium, which dominate the disk's mass.
- Temperature Gradients: The inner disk being hotter than the outer parts creates zones conducive to different chemical processes.
Understanding these characteristics is essential because they dictate the conditions in which protoplanets develop. Gravitational interactions within the disk can result in instabilities, leading to the formation of clumps that eventually aggregate into larger bodies.
The Process of Protostar Formation
The protostar formation involves several stages characterized by the interplay of gravity, pressure, and temperature. It begins when a region of a molecular cloud becomes dense enough for gravity to take over, pulling gas and dust together. As the material collapses, its gravitational energy converts into thermal energy, raising the temperature at the core of the nascent star.
During this phase:
- Collapse Initiation: A molecular cloud starts to condense due to instabilities, leading to localized increases in density.
- Heating of the Core: As the mass accumulates, the center becomes very dense and hot, forming what is recognized as a protostar.
- Protostar Ignition: Eventually, when temperatures are sufficiently high, nuclear fusion begins in the core. This marks the transition from a protostar to a main-sequence star.
This process takes millions of years, but it is during this time that the foundation for planetary formation is being laid out. Tracks of material spinning around the forming star create a disk—setting the stage for accretion and the eventual birth of planets like Earth.
"The formation of a protostar is a critical nexus in the chronology of cosmic evolution, influencing everything from star formation to the genesis of planetary systems."
In summary, the formation of the protostar and the protoplanetary disk are essential phases in the chronology of events leading to Earth's development. They establish the initial conditions and materials necessary for planetary formation, influencing not only the composition of Earth but also the potential for life as we know it.
Accretion Phase of Earth’s Formation
The accretion phase of Earth’s formation is a vital segment of planetary evolution, acting as a bridge between the initial formation of solid material in the solar nebula and the creation of a fully developed Earth. This process marks the period during which small particles gradually coalesce into larger bodies, ultimately forming the planet we inhabit today. Understanding this phase helps in appreciating the scale and complexity involved in planetary construction, as well as the conditions that made the eventual emergence of life possible.
During the accretion phase, countless tiny particles, known as planetesimals, came together due to gravitational attraction. This collective process transformed small objects into larger entities, resulting in a hot, molten state due to the immense energy generated by impacts and collisions. As time passed, these growing masses began to differentiate based on density and composition, setting the groundwork for Earth's lithosphere, atmosphere, and hydrosphere.
Accretion not only contributed to the mass of the young Earth but also introduced a variety of materials—ranging from metals to volatile compounds—that are essential for life. The distribution and types of these materials influenced subsequent geological and biological processes. Therefore, acknowledging the significance of the accretion phase is crucial for understanding the origin of Earth in the larger context of cosmic and geological phenomena.
Collisional Growth of Planetesimals
The collisional growth of planetesimals is a core aspect of the accretion phase. Planetesimals, which formed from dust and gas in the protoplanetary disk, began to collide and stick together, leading to the creation of larger bodies called protoplanets. This growth was not a gentle process; rather, it was characterized by frequent and significant impacts that produced considerable heat and pressure.
These collisions were critical for several reasons:
- Energy Generation: The kinetic energy from these impacts transformed into thermal energy, significantly increasing temperatures within the forming planetesimals.
- Material Accumulation: Larger bodies could attract and accumulate more material, promoting growth exponentially compared to smaller ones.
- Diversity of Composition: Different types of planetesimals contributed varying minerals and elements, enriching the eventual formation of Earth’s crust.
For example, studies suggest that the cores of some planetesimals may have had heavier metals such as iron and nickel, while their outer layers contained silicates and volatiles. The diverse origins of these colliding bodies played a role in shaping the chemical and physical properties of early Earth. This collisional process continues today in the form of meteor impacts, which sometimes scatter remnants of this ancient process across our planet.
Formation of Earth’s Early Structure
The formation of Earth’s early structure involves the early differentiation of the planet as it cooled down. As planetesimals merged, the resulting body began to develop distinct layers due to variations in density and temperature. Heavy materials, such as iron, migrated toward the center, while lighter silica-based compounds formed the crust. This layering is a fundamental characteristic of terrestrial planets.
Key components of early Earth structure formation include:
- Core Formation: As the planet continued to grow and heat up, the densest materials sank to form the core. This core would eventually become the source of Earth’s magnetic field.
- Mantle Development: Surrounding the core, the mantle formed from materials that were less dense, allowing for convection processes that would later influence plate tectonics.
- Formation of the Crust: As temperatures decreased, a solid crust began to form. This crust, however, was still unstable and subject to frequent volcanic activity, which played a role in shaping the landscape and the atmosphere.
In summary, the early structure of Earth was not just a result of physical processes but also a precursor for future geological activity. The alignment of materials, their distribution, and the dynamics at play would have profound implications for Earth as it developed further.
"The accretion phase is not merely the construction of a planet; it is an intricate dance of physics, chemistry, and time that shapes the very characteristics of worlds."
Understanding these formative processes provides crucial insights into the conditions required for life and the long-term evolution of our planet.
Differentiation and Early Geological Activity
The process of differentiation and the subsequent geological activity that followed were critical to the early development of Earth. They set the stage for the planet’s structure, composition, and capacity for supporting life. Differentiation refers to the process whereby materials in a planet separate into layers due to variations in density. This physical transformation influenced the thermal evolution of Earth and the emergence of a more stable environment.
Understanding Planetary Differentiation
Planetary differentiation occurs when a mixture of materials within a planetary body, such as rock and metal, undergoes a change in position based on density. Heavier materials tend to sink, while lighter materials rise. This led to the formation of the core, mantle, and crust of Earth. The core, largely composed of iron and nickel, formed at the center, while the mantle and crust developed above it.
This stratification allowed for distinct geochemical environments. Each layer has unique properties that affect everything from tectonics to volcanic activity. Furthermore, differentiation is crucial for the development of a magnetic field, generated by movements in the liquid outer core. This magnetic field protects Earth from harmful solar radiation, which is essential for maintaining an atmosphere conducive to life.
Impact of Radioactive Decay
Radioactive decay played a significant role in both differentiation and the geological processes that shaped Earth. As radioactive isotopes, like uranium and thorium, decay, they release heat. This heat contributes to the geothermal gradient, facilitating layering as lighter materials migrate toward the surface. The decay of these isotopes also affects the thermal history of the planet, assisting in driving mantle convection, a vital mechanism for plate tectonics.
Additionally, the heat generated by radioactive decay is not just about differentiation. It also leads to volcanic activity and the recycling of materials within the Earth's crust. Volcanic eruptions can introduce new elements to the surface, enriching the crust with essential nutrients, which ultimately benefits the development of an atmosphere and potential life forms.
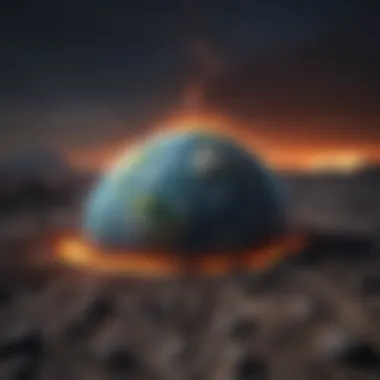
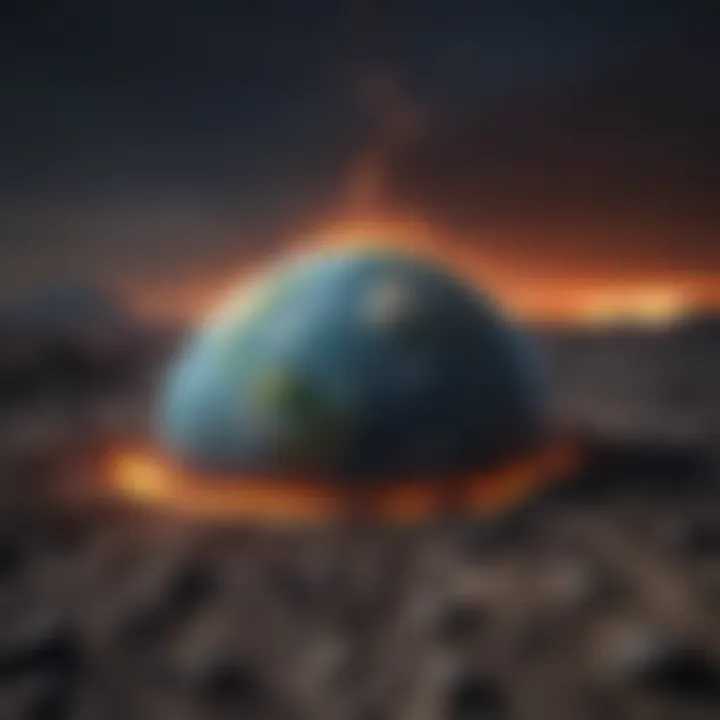
"Differentiation and the heat from radioactive decay are the twin forces that shaped the geological landscape of early Earth, creating conditions under which life could eventually arise."
The interplay between these processes is complex yet essential for understanding Earth's early geological history. The implications extend well beyond the planet's formation, influencing its subsequent evolution and ability to host diverse forms of life.
Through studying differentiation and its consequences, we gain insights into not only Earth’s past but also its present and future. The processes are a reminder that the very ingredients for life were forged in the chaotic and transformative early epochs of our planet.
The Formation of the Moon
Understanding the formation of the Moon is crucial for comprehending Earth's history and evolution. The Moon plays a significant role in stabilizing Earth's axial tilt, which influences climate and seasonal variations. Its presence has not only impacted geological processes but has also shaped biological evolution on Earth. The formation of the Moon is tied closely to the events that characterized the early Solar System, providing insight into the processes involved in planetary formation.
The Giant Impact Hypothesis
The Giant Impact Hypothesis is the leading theory explaining the origin of the Moon. According to this hypothesis, approximately 4.5 billion years ago, a Mars-sized body named Theia collided with the young Earth. This cataclysmic event resulted in a significant amount of debris being ejected into space. This debris eventually coalesced to form the Moon.
This hypothesis aligns with various lines of evidence:
- Isotopic Similarities: Lunar rocks retrieved during the Apollo missions share isotopic characteristics with Earth, indicating a common origin.
- Model Simulations: Numerous computer simulations support the plausibility of such an impact and show that it could result in the observed size and composition of the Moon.
- Angular Momentum: The current Earth-Moon system exhibits a level of angular momentum that is consistent with the impact scenario.
The Giant Impact Hypothesis provides a comprehensive framework to understand how major celestial events shape planetary bodies, particularly in the context of our Solar System.
Effects of the Moon on Earth’s Development
The Moon has profoundly influenced the development of Earth in several significant ways:
- Tidal Forces: The gravitational pull of the Moon contributes to tides in Earth's oceans. This tidal action has played a crucial role in shaping coastal ecosystems and influencing climate systems.
- Axial Stability: The Moon's presence stabilizes Earth's axial tilt. This stability leads to predictable climatic patterns over geological time scales. It reduces the randomness of climate variations, supporting a more hospitable environment for life.
- Biological Effects: The rhythmic nature of the tides may have influenced early biological evolution, creating an environment where life could flourish in coastal areas.
Establishment of the Atmosphere and Hydrosphere
The establishment of Earth's atmosphere and hydrosphere is a key topic in understanding how our planet transitioned from a lifeless mass to a vibrant environment capable of supporting life. This phase in Earth's formation is not just about the presence of gases and oceans; it relates to the intricate processes that shaped these elements and their interaction with geological activity, biological evolution, and environmental conditions.
The atmosphere, primarily composed of nitrogen and oxygen, acts as a shield against solar radiation and helps regulate temperature. Without an atmosphere, the planet would experience extreme temperature fluctuations, making it inhospitable for any form of life. Similarly, the hydrosphere, which includes all water bodies, plays a crucial role in weather patterns and as a medium for biochemical reactions vital for life. The development of both systems was influenced by various geological and celestial factors.
Understanding how the atmosphere and hydrosphere came to be helps to trace the Earth’s history and evolution. Key components in this phase involve processes such as volcanic outgassing and the contributions from celestial bodies like comets. By delving into these factors, one can appreciate the complex interplay that led to the formation of conditions necessary for life.
Volcanic Outgassing and Atmospheric Composition
Volcanic outgassing is a significant process that contributed to the formation of Earth’s early atmosphere. As the young Earth cooled, volcanic activity released gases such as water vapor, carbon dioxide, sulfur dioxide, and nitrogen into the atmosphere. Initially, the composition was very different from today's, heavily favoring greenhouse gases which created a thick and hazy atmosphere.
Over time, the presence of water vapor played a transformative role. As the planet cooled further, much of this vapor condensed to form oceans. The vast expanses of water acted as a sink for carbon dioxide, reducing its concentration in the atmosphere. This process eventually allowed for the development of conditions suitable for life.
"Volcanic outgassing is a pivotal driver behind not just atmospheric composition, but also climate development on early Earth."
Key points to note about volcanic outgassing include:
- Gaseous Releases: Primarily consisted of steam, carbon dioxide, and nitrogen.
- Oceans Formation: Water vapor leading to heavy rainfall, resulting in the formation of bodies of water.
- Climate Regulation: Early greenhouse effects maintaining temperatures conducive for liquid water.
The Role of Comets and Asteroids
Comets and asteroids played a crucial role in supplementing Earth’s atmosphere and hydrosphere. These bodies, containing ice and other volatile substances, impacted Earth during its formative years. Upon collision, they released substantial amounts of water and other organic compounds. This transfer influenced both the chemical makeup of the atmosphere and the availability of water on the surface.
Research suggests that a significant volume of Earth's water may have originated from icy comets and water-rich asteroids. In addition to contributing to the hydrosphere, these celestial bodies also brought various minerals and organic compounds which could have set the stage for the emergence of life.
Noteworthy aspects include:
- Water Delivery: Comets potentially providing additional water resources, essential for ocean formation.
- Organic Compounds: Introduction of complex molecules which could serve as precursors for the development of life.
- Atmospheric Impact: Effects of massive collisions influencing atmospheric conditions and climate patterns.
In summary, the establishment of the atmosphere and hydrosphere is woven into the fabric of Earth's formative events. The blend of volcanic activity and contributions from outer space laid the groundwork for a planet ready to evolve into a cradle for life.
The Emergence of Life on Earth
The emergence of life on Earth represents a pivotal milestone in the narrative of our planet. Understanding how life began is essential not just for comprehending our own existence but also for grasping the broader context of life's development across the universe. Numerous conditions had to converge to create a suitable environment for life to emerge. Examining these requirements and the various theories surrounding the origin of life sheds light on the delicate balance that supports ecosystems today.
Conditions for Life: A Narrow Path
Life as we know it demands a specific set of conditions.
- Liquid Water: Water is vital as it serves as a solvent for biochemical reactions. Without it, essential processes cannot occur. Earth’s unique distance from the Sun ensures that temperatures allow water to exist in liquid form.
- Stable Temperature Range: For life to thrive, the planet must maintain a temperature range that permits chemical reactions without extreme conditions. Earth’s atmosphere and magnetic field play critical roles in regulating temperature.
- Chemical Nutrients: Elements like carbon, nitrogen, phosphorus, and sulfur are crucial for building the molecules necessary for life. These elements must be present in adequate quantities.
- Energy Sources: Life also requires energy, either from sunlight or chemical sources. This energy fuels metabolic activities fundamental to survival.
The combination of these factors allows certain environments, such as deep-sea vents and primordial ponds, to potentially host early forms of life.
Theories on the Origin of Life
Several theories have been proposed regarding how life began on Earth. Each theory offers different perspectives, showcasing the complexity of this subject.
- Primordial Soup Theory: This idea suggests that life began in a warm pond or a similar environment rich in organic compounds. The right conditions would facilitate chemical reactions, eventually leading to the formation of simple life forms.
- Hydrothermal Vent Hypothesis: Some scientists propose that life may have originated near hydrothermal vents, where hot water laden with minerals feeds on chemical reactions. This environment may have provided the necessary energy and raw materials for early life.
- Panspermia: This theory posits that life, or its precursors, may have come from outer space, delivered to Earth by meteorites or comets. If true, it expands the question of life’s origin beyond our planet.
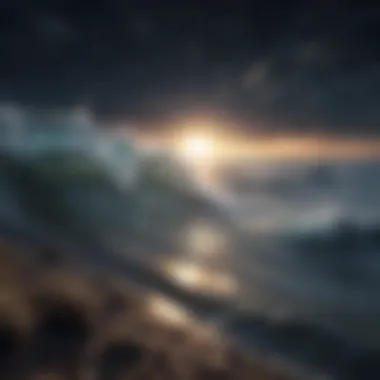
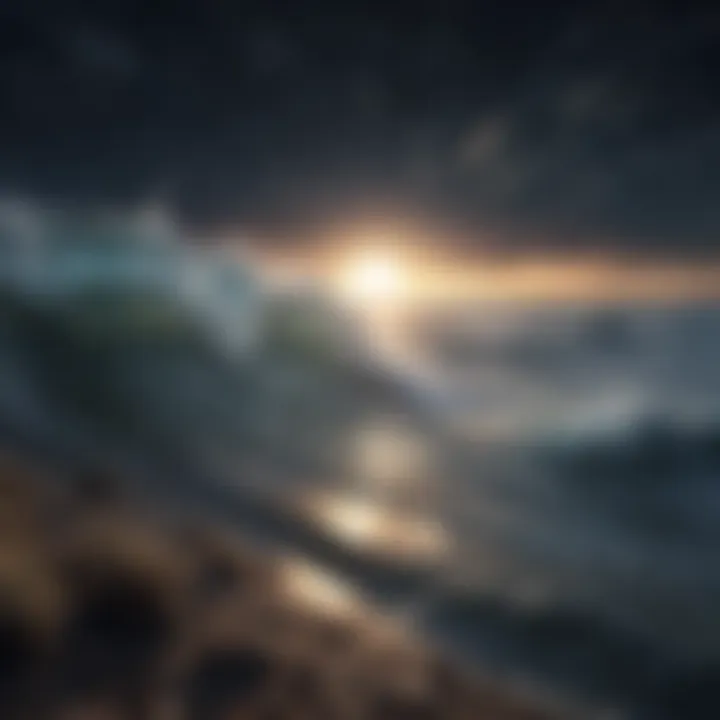
Despite the various hypotheses, no single theory has gained unanimous acceptance. Ongoing research continues to investigate these possibilities further.
These discussions encapsulate the significance of life's emergence in understanding Earth’s history. By studying the origins of life, we do not only learn about our past but also inform the search for extraterrestrial life.
The quest for discovering life's foundations enhances our grasp of fundamental biological processes and reactions, while unraveling the mysteries of our planet's past.
Geological Time Scale and Earth’s Evolution
Understanding the geological time scale is critical when examining Earth's history. This framework provides a way to organize the vast amount of geological and biological history into manageable units. Not only does it help geologists, but it also aids researchers and educators in understanding the progression of life on Earth and the significant events that shaped our planet.
The geological time scale is divided into several hierarchical categories: eons, eras, periods, epochs, and ages. Each level signifies a specific time frame during which notable geological, climatic, and biological changes occurred. One of the key benefits of this scale is that it allows scientists to date and correlate rock layers, making it possible to study the evolutionary processes over millions or even billions of years. This chronological context is invaluable for understanding how different life forms emerged and adapted over time, reflecting both environmental change and biological evolution.
One important consideration when studying the geological time scale is the concept of uniformitarianism, which posits that the same natural laws and processes we observe today have been at work throughout Earth's history. This idea serves as a backdrop for much of geological research, linking the past with the present.
"The geological time scale serves as a time-machine that transports us back through the ages, allowing us to witness Earth’s transformative journey."
Understanding the Geological Time Scale
The geological time scale arose from the need to understand Earth’s complex history. It encompasses four primary eons:
- Hadean
- Archean
- Proterozoic
- Phanerozoic
Each eon is further divided into eras, where significant geological and biological events occurred. For example:
- Phanerozoic Eon: This eon is marked by the emergence of widespread and diverse life forms, starting 541 million years ago and continuing to the present day. It is divided into three eras: Paleozoic, Mesozoic, and Cenozoic.
- Archean Eon: This is the period when the first simple life forms started to appear, dating back around 4 billion years ago.
Through meticulous research, scientists have dated rocks and fossils, allowing for a more complete timeline of Earth’s history. By utilizing techniques such as radiometric dating, they provide a framework that enhances our understanding of geological events, climatic shifts, and the evolution of life throughout different epochs.
The Major Eras of Earth's History
The major eras of Earth’s history, as defined by the geological time scale, include the following:
- Paleozoic Era: Lasting from approximately 541 to 252 million years ago, this era marks the Cambrian explosion, a time of rapid diversification of life. Various life forms, including fish, amphibians, and reptiles, evolved during this period.
- Mesozoic Era: Known as the age of dinosaurs, this era occurred around 252 to 66 million years ago. It is characterized by the dominance of dinosaurs and is subdivided into three periods: Triassic, Jurassic, and Cretaceous.
- Cenozoic Era: Following the mass extinction event that eliminated the dinosaurs, the Cenozoic, also known as the age of mammals, has played a crucial role in shaping the modern diversity of species. This era started 66 million years ago and continues to the present day. It is further divided into the Paleogene, Neogene, and Quaternary periods.
Understanding these eras provides insight into how life has adapted to changing environments, including mass extinctions and climatic shifts. Each era plays a role in the narrative of Earth’s evolution, reflecting both geological transformations and the resilience of life.
In summary, the geological time scale is essential for a comprehensive understanding of Earth's history. It serves as a framework that connects the past to the present, allowing scholars to explore the intricate processes that have shaped our planet through time.
Contemporary Research on Earth’s Formation
The study of Earth's formation continues to be a dynamic and multi-faceted area of research. This section emphasizes the significance of contemporary investigations and the methods employed in understanding planetary formation. Researchers are not only looking at Earth's early days but also connecting these findings to broader astrophysical contexts. Understanding how Earth formed provides insights into the complexities of planetary formation across the universe. This knowledge aids in deciphering how different planets evolve and potentially support life.
Advancements in Geological Methods
Recent advancements in geological methods have revolutionized our approach to understanding Earth’s formation. Until now, traditional techniques focused on the analysis of rock samples and fossil records. Modern technology, however, has introduced more precise tools such as laser ablation mass spectrometry and field portable X-ray fluorescence systems. These innovations allow scientists to measure isotopic compositions with enhanced accuracy, shedding light on the conditions during Earth's formation.
The continuous sampling of ancient rocks through direct coring provides facts essential for piecing together stratigraphic records. Moreover, methods such as radiometric dating enable the determination of ages of rock samples with high reliability. This precision is vital for constructing timelines of geological events, providing clarity on how quickly Earth's structure developed, how it differentiated, and the timescale of significant processes impacting its evolution.
"The integration of advanced isotopic analysis with traditional geological methods enables a more holistic understanding of Earth’s formative processes."
Astrobiological Implications
The findings from contemporary research into Earth’s formation have profound implications for astrobiology. By understanding the unique conditions that allowed Earth to become a cradle for life, researchers can better assess the habitability of exoplanets. The factors that contributed to the emergence of life on Earth, such as the presence of water and a stable climate, are central to evaluating potential life-supporting environments elsewhere in the universe.
Ongoing studies highlight the importance of looking for similar geological features on other planets. For instance, identifying volcanic activity, tectonic plate movements, and atmospheric composition is crucial in exoplanet investigations. The quest to find planets in the so-called "Goldilocks Zone"—where conditions may be just right for life—has become more targeted, thanks to insights gained from Earth's own evolution.
Ending and Reflections
The conclusion of this article synthesizes the intricate journey of Earth's formation. Understanding this process is not only crucial for geologists and astronomers but for anyone interested in the origin and evolution of our planet. The formation of Earth represents a convergence of numerous physical processes, astronomical events, and chemical evolutions. Each key insight detailed throughout this article provides a deeper appreciation for the cosmos.
The reflections outlined here point to several essential elements. One significant consideration is the role of interdisciplinary approaches in enhancing our understanding of Earth's formation. The integration of geology, astronomy, and biology has shed light on how our planet evolved over billions of years. These fields work together to provide a more comprehensive picture of how the Earth's environment and life-supporting capabilities came to be.
Another benefit of examining Earth's formation lies in its implications for understanding the conditions required for life elsewhere in the universe. By studying the unique circumstances that fostered life on Earth, scientists can better identify exoplanets that may share similar attributes.
"Earth is the only known planet that supports life, making its formation story vital for astrobiology and planetary science."
In summary, the reflections on the formation of Earth highlight the importance of continuing research. A systematic investigation into our planet's history enables humanity to grasp not only where we come from but also how we might navigate the challenges of the future.
Summary of Key Insights
The article presented several pivotal insights:
- The Nebular Hypothesis: This theory illustrates that our solar system formed from a massive cloud of gas and dust. It set the stage for planetary accretion.
- The Role of Gravity: Gravity played a critical role in the accretion of materials, cementing the structure of what would become Earth.
- Differentiation: Early geological activities, driven by heat from radioactive decay, allowed Earth to develop its layered structure, with a core, mantle, and crust.
- Formation of the Moon: The Giant Impact Hypothesis offers a plausible explanation for the Moon's formation. Its presence has influenced Earth’s axial tilt and stabilizes its climate.
- Emergence of Life: Understanding the conditions that allowed life to flourish on Earth informs our search for extraterrestrial life.
The cumulative insights emphasize Earth's unique and delicate balance, shaped by complex processes over time.
Future Directions in Earth Sciences
Looking ahead, several future directions in Earth sciences appear promising:
- Technological Advancements: Improved remote sensing technologies will enhance our ability to study distant celestial bodies and their formation, giving insight into planetary systems beyond our own.
- Astrobiology: Continued exploration of extreme environments on Earth can provide clues about life on other planets. This field will rely heavily on interdisciplinary research.
- Geological Research: Ongoing investigations in areas like plate tectonics and mineral resources remain vital. The formation processes on Earth must be understood to predict future geological conditions.
- Climate Studies: Studying the relationship between Earth's formation and its climate can offer insights into long-term sustainability. Examining past climates could provide guidance on addressing current climate change challenges.
Future research will build upon the groundwork laid by past findings, striving to create a more interconnected understanding of planetary formation and evolution. Emphasizing collaboration and emphasizing new technologies will facilitate breakthroughs that can enrich the field of Earth sciences.