The Intricacies of Quantum Vibrations in Science
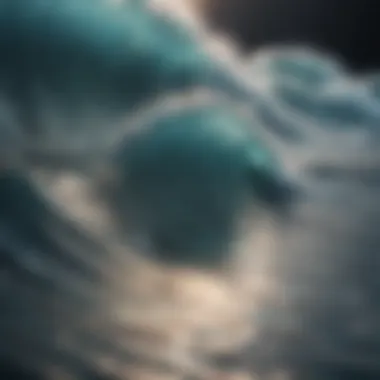
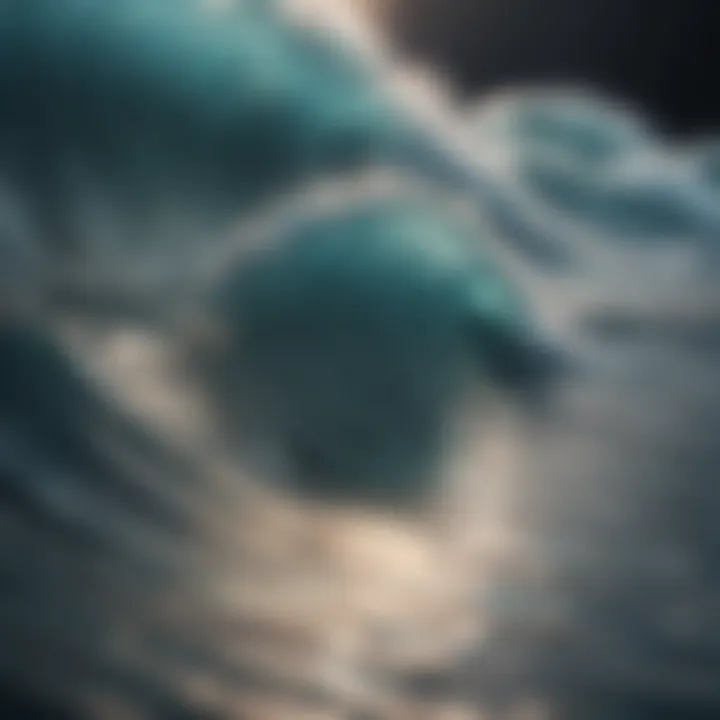
Intro
The universe, with its intricate web of interactions, operates on principles that often elude our everyday experiences. At the microscopic level, a captivating interplay occurs, one that challenges our understanding of both energy and matter. This phenomenon, known as quantum vibrations, serves as a key to deciphering the behaviors of particles and waves that form the foundation of our existence.
In this exploration, we will dive into the nuances of quantum vibrations, shedding light on their significance across various scientific domains. By unraveling these subtle oscillations that exist at the quantum level, we can begin to grasp the unseen forces that govern everything from the materials we use, to biological processes and even the fabric of spacetime itself.
And so, we embark on an intellectual journey into the world of quantum vibrations, unearthing groundbreaking findings, breaking down complex theories, and ultimately synthesizing what this means for our ongoing quest for knowledge.
Key Research Findings
Overview of Recent Discoveries
Recent advancements in the field of quantum physics have shed new light on the nature of quantum vibrations. In 2021, researchers at the Massachusetts Institute of Technology published findings that highlighted how quantum vibrations play a crucial role in heat transfer at the atomic level. This research is pivotal as it lays the groundwork for developing more efficient materials.
Moreover, a 2023 study from the University of California revealed that these vibrations can influence chemical reactions. The simultaneous oscillation of molecules suggests that quantum vibrations may be harnessed to accelerate important reactions critical to fields like catalysis and energy conversion.
Significance of Findings in the Field
The implications of these discoveries extend far beyond theoretical physics. Understanding quantum vibrations can lead to insightful applications, such as:
- Enhanced material design: Creating materials that leverage quantum properties for greater strength or lighter weight.
- Advancements in medical imaging: Utilizing quantum vibrations to improve imaging techniques or therapy applications.
- Energy efficiency: Developing new methods for energy transfer or storage systems that incorporate quantum vibrations to minimize losses.
With the advances mentioned above, researchers are beginning to put together pieces that reflect how quantum vibrations not only embody a dance of energy and matter but also serve as a bridge to understanding interactions within our larger universe.
"Every discovery opens a new door in the labyrinth of knowledge, revealing insights that were once concealed in the shadows of ignorance."
Breakdown of Complex Concepts
Simplification of Advanced Theories
While the scientific principles surrounding quantum vibrations may seem daunting, breaking them down into simpler concepts makes them more graspable. Quantum vibrations are essentially the tiny oscillations of particles at the quantum level. When we think about particles like electrons, they do not behave like traditional balls moving in a straight line. Instead, they vibrate, similar to how a guitar string resonates when plucked.
This vibration can affect how particles interact with each other. A useful analogy here is a crowded room where everyone is dancing differently. Some people are moving in sync with the music, while others are lost in their own rhythm. Similarly, in quantum vibrations, the interactions can lead to cooperative behaviors among particles that can either enhance or diminish certain processes, depending on their vibrational states.
Visual Aids and Infographics
To further illustrate the concept of quantum vibrations, visual aids can be invaluable. For instance, diagrams depicting vibrational modes of molecules can clarify how energy is distributed among atoms in a compound. Websites like Wikipedia and Britannica provide excellent resources that visualize these complex interactions.
Utilizing these resources allows for a clearer understanding of advanced concepts, making this sophisticated scientific topic accessible to a broader audience—ideal for students and educators aiming to explore the depths of quantum mechanics.
In this unfolding narrative, we will continue exploring the implications of quantum vibrations, weaving through fields that range from theoretical underpinnings to practical applications that could redefine how we interact with the world around us.
Intro to Quantum Vibrations
In the realm of physics and the natural sciences, few subjects evoke both curiosity and complexity quite like quantum vibrations. These delicate oscillations serve as a foundational concept in understanding how energy and matter interact at the most fundamental level. With roots deep in the principles of quantum mechanics, the study of quantum vibrations not only enriches our knowledge of the microscopic world but also influences various innovative applications in technology and beyond. The discourse surrounding this topic provides insights that range from the very essence of atomic interactions to the implications for future technologies like quantum computing and material science.
Recognizing the significance of quantum vibrations is vital for several reasons. First and foremost, they allow researchers to probe the underlying behaviors of particles and the forces that govern them. While everything from your morning coffee to advanced electronic devices is influenced by these phenomena, grasping their subtleties opens doorways to future innovations and applications. Moreover, exploring this topic helps demystify the enigmatic world of quantum physics, making it more accessible to students, educators, and professionals alike.
The close-knit relationship between energy and matter becomes clear when delving into quantum vibrations. These oscillations reflect the dynamic and constantly changing nature of the quantum world. As such, they herald not just a shift in scientific understanding but also serve as a bridge connecting various fields of study. In this article, we will unravel the intricate details of quantum vibrations, exploring their theoretical foundations, experimental insights, practical applications, and the challenges that lie ahead in this fascinating domain.
Definition and Overview
Defining quantum vibrations succinctly is no easy task, as they encompass a broad spectrum of behaviors and phenomena. At their core, quantum vibrations can be understood as the fluctuations of energy states within quantum systems. These fluctuations represent the probabilistic nature of particles at the quantum level, wherein entities like electrons and photons do not exist in fixed states, but rather exhibit a tapestry of potential states, influenced by their surroundings.
When considering energy states, quantum vibrations play a pivotal role. These states are akin to multiple rungs on a ladder, with particles oscillating between them due to energy absorption or emission. This movement isn’t merely random; instead, it’s governed by the rules of quantum mechanics, where wave functions and probabilities dictate behavior. In essence, quantum vibrations are the heartbeat of atomic and subatomic systems, reflecting the fluid nature of existence itself.
Historical Context
The exploration of vibrations at the quantum level is not a newfound curiosity. The journey into this realm has roots that trace back to the early 20th century, around the time quantum mechanics was born. Pioneering scientists like Max Planck and Albert Einstein laid the foundational stones of quantum theory by introducing the idea that energy is quantized—a radical departure from classical physics that helped to explain phenomena such as blackbody radiation and the photoelectric effect.
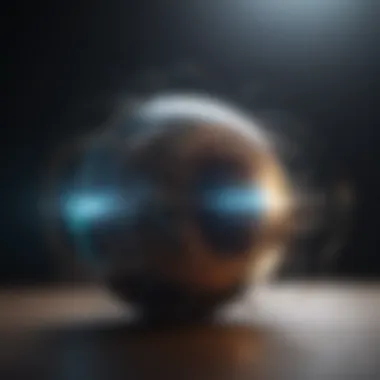
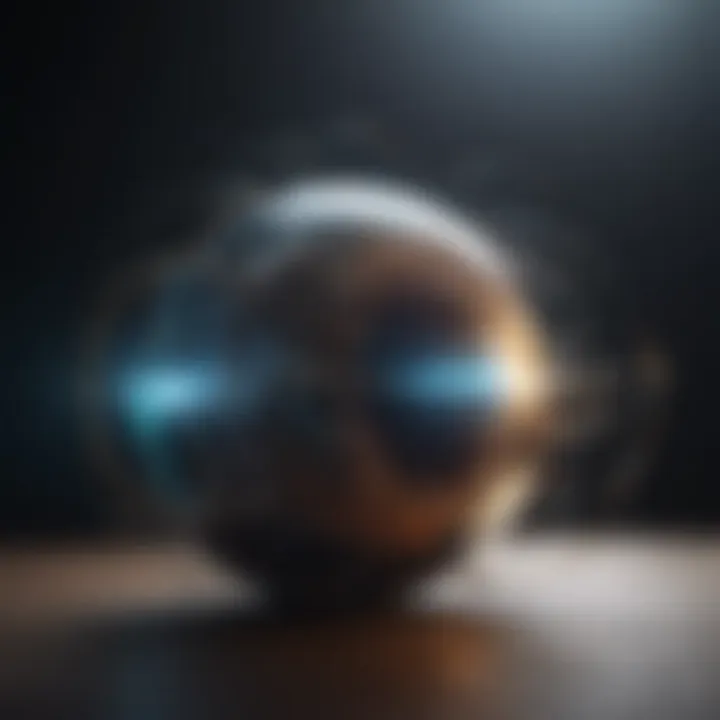
As research progressed, the concept of vibrations became increasingly central to understanding atomic behavior. The work of Niels Bohr provided models for the atomic structure, illuminating how energy levels could be quantized and how these levels relate to vibrational states. The development of quantum field theory further enriched this understanding, enabling physicists to frame particles not as isolated entities but as excitations within fields, subject to the dance of energy and vibrations.
Thus, quantum vibrations encapsulate a rich legacy of scientific inquiry. Each evolution in thought not only deepens our comprehension of the microscopic world but also sparks new questions, propelling research into uncharted territories. Today, as we stand at the confluence of quantum mechanics and modern technology, the impact of these vibrations reverberates through various disciplines, hinting at potential discoveries waiting to be unearthed.
Theoretical Foundations
The study of quantum vibrations lies at the heart of modern physics, unlocking a window into the very nature of matter and energy to unravel the intricate ballet between them. Understanding the theoretical foundations equips readers with a solid framework to comprehend phenomena that seem counterintuitive at first glance. This section serves as a cornerstone of the article, shedding light on basic concepts that lay the groundwork for exploring more complex relationships in subsequent sections. By diving into the principles that govern quantum mechanics, readers can better appreciate how these vibrations manifest in various applications, influencing technology and our understanding of the natural world.
Quantum Mechanics Basics
To grasp quantum vibrations, one must first understand the principles of quantum mechanics. Quantum mechanics, fundamentally, is the study of particles at atomic scales and how they behave. This realm, unlike classical physics, operates under different rules, where particles do not occupy fixed positions and the act of observation often alters their state.
A couple of key aspects include:
- Superposition: Particles can exist in multiple states simultaneously until observed. Imagine flipping a coin; until it lands, it's both heads and tails, similar to how a quantum particle can occupy several states.
- Entanglement: This unique phenomenon occurs when particles become interlinked, such that the state of one instantaneously affects another, regardless of distance. It’s as if two dancers are perfectly in sync, no matter how far apart they are.
These principles provide a framework for the fluctuations in energy that contribute to quantum vibrations, influencing everything from atomic interactions to macroscopic systems.
Wave-Particle Duality
One of the most intriguing concepts in quantum physics is wave-particle duality. This principle suggests that particles such as electrons, which we usually picture as tiny balls, can also exhibit wave-like behavior. It challenges our classical intuition but offers deep insights into the nature of reality.
For instance, when electrons are fired at a double-slit barrier, they produce an interference pattern—characteristic of waves—when not observed. Yet, when we measure them as they pass through the slits, they behave like particles, landing in distinct spots. This duality demonstrates that energy and matter are not always definable in one way, kind of like viewing the same illustration differently depending on the angle you look from.
In summary, wave-particle duality serves as a gateway to understanding quantum vibrations. When energy levels shift and particles interact, they oscillate between these two states, defining their vibrational characteristics. This is not just an abstract theory; it lays the groundwork for applications in fields such as quantum computing, where harnessing these oscillations can lead to breakthroughs in processing power and information transfer.
"Quantum theory cannot be reconciled with classical physics, because it reveals that the universe doesn’t always dance to the same tune we expect it to." - Unknown
Ultimately, acknowledging the theoretical foundations of quantum mechanics and the concept of wave-particle duality sets a solid stage for further exploration of quantum vibrations. It intertwines a theoretical framework with experimental realities, guiding us toward innovative fields in sciences and technology.
Nature of Vibrations at the Quantum Level
Exploring the nuances of vibrations at the quantum level reveals a fascinating layer of complexity and significance in modern physics. This section serves as a crucial pivot, connecting the abstract theoretical underpinnings of quantum mechanics to tangible applications in various fields. In essence, understanding quantum vibrations sheds light on the subtle yet powerful interactions of energy and matter. It creates a pathway through which one can appreciate the intricate relationships that govern our universe.
Energy States and Quantum Harmonics
At the heart of quantum vibrations lies the concept of energy states. These states can be thought of as the various ways in which a quantum system can exist while retaining distinct energy levels. It's similar to a musical scale; just as a note can vary in pitch, a quantum particle oscillates between energy levels. This phenomenon is not just a theoretical fancy; it is central to how matter behaves at microscopic scales.
For instance, consider an electron in an atom. It occupies certain energy states defined by quantum mechanics. When energy is added, such as from an incoming photon, the electron may leap to a higher state, exhibiting vibrational characteristics through its transition. Conversely, when the electron drops back to its original state, it emits energy, often in the form of light. This emission is a critical aspect of quantum harmonics, where specific wavelengths relate to energy differences between states.
Energy levels in quantum systems can be likened to the rungs on a ladder; an electron must ascend or descend distinct steps to change its state.
Additionally, the concept of quantum harmonics allows us to understand phenomena that might seem baffling at first. Take, for example, lasers. They function on the principle of stimulated emission, where photons coax electrons into consistent vibrational modes, generating coherent light. Therefore, examining energy states and harmonics not only enhances our grasp of atomic behavior but also paves the way for revolutionary technological advancements.
Role of Quantum Fluctuations
Within the quantum realm, fluctuations are not just random; they serve fundamental roles that influence how particles interact. Quantum fluctuations refer to the temporary changes in energy that occur in empty space due to the Heisenberg uncertainty principle. Picture a calm lake suddenly rippling due to unseen factors; similarly, the quantum vacuum is never truly ‘empty’ but is alive with transient energy bursts.
These fluctuations can lead to various important phenomena. For instance, the Casimir effect illustrates how two uncharged plates placed in a vacuum can exhibit an attractive force solely due to fluctuations in the quantum field. Such examples highlight a dimension of reality where things aren't as straightforward as they appear; the dance of energy and matter is ongoing and deeply intricate.
Moreover, when examining the role of quantum fluctuations, it becomes evident how they contribute to the creation of virtual particles. These fleeting entities exist only for short periods, coming into existence and annihilating almost instantaneously. Although they might seem trivial, their impact on physical processes is profound, influencing everything from the stability of vacuum states to the properties of materials at a microscopic level.
In summary, understanding quantum vibrations at the fundamental level is not merely an academic exercise. It allows scientists, researchers, and enthusiasts alike to glean insights into the building blocks of matter and energy, fostering advancements in technology and enriching our grasp of the universe.
Experimental Insights
Understanding quantum vibrations is no simple task, yet the insights gleaned from experimental approaches provide a clearer view of how these phenomena operate in the realm of energy and matter. Experimental insights not only validate theoretical frameworks but also push the boundaries of what is known in quantum mechanics and related fields. As researchers continuously face the challenges of measuring such tiny and elusive phenomena, advancements in experimental techniques have become pivotal for progress. This section emphasizes why grasping these experimental insights is critical in uncovering the mysteries of quantum vibrations and their far-reaching implications.
Techniques for Measuring Quantum Vibrations
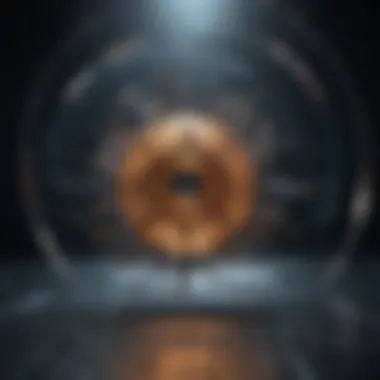
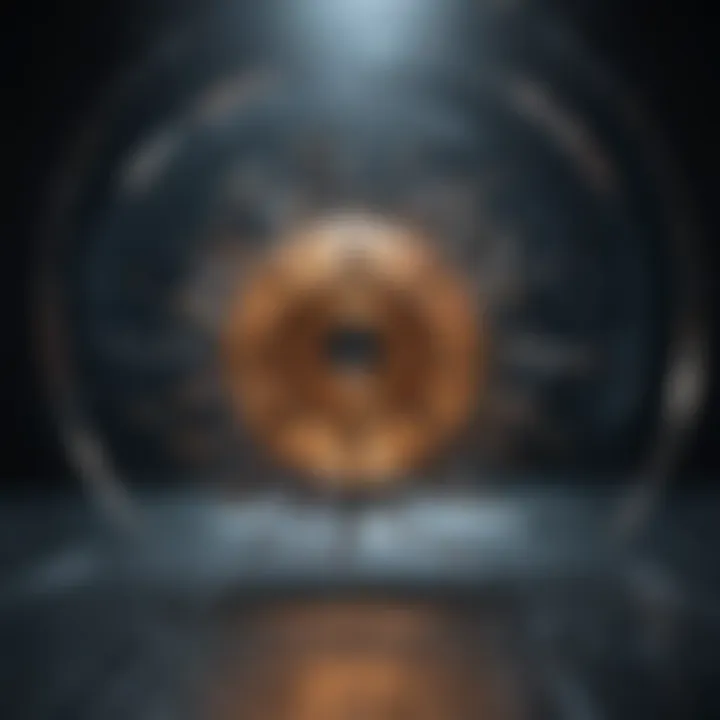
Several innovative techniques have emerged for measuring quantum vibrations, enabling scientists to capture the nuances of this unseen world. Here are some key methods:
- Optical Tweezers: This method uses focused laser beams to manipulate microscopic particles. When applied to quantum systems, optical tweezers can isolate particles, allowing researchers to study the effects of vibrations imparted on them.
- Quantum Resonance: Employing techniques from nuclear magnetic resonance (NMR), this approach measures energy transitions of particles at quantum levels. By understanding resonance patterns, scientists can deduce information about vibration states.
- Cryogenics: Low-temperature environments help reduce thermal noise and enhance sensitivity in detecting quantum vibrations. Operating at these temperatures allows for clearer observations of quantum states.
- Interferometry: With this high-precision technique, scientists can observe phase shifts in light waves caused by quantum vibrations. Interference patterns provide crucial data for analyzing vibrational states of particles.
These techniques reveal a fusion of creativity and technical advancement, each addressing the issue of precision. Coupled with sophisticated instrumentation, these methods contribute to a deeper understanding of quantum vibrations.
Recent Experimental Findings
Recent research has unveiled astounding discoveries in the field of quantum vibrations, highlighting their complexity and implications for various domains. Some noteworthy findings include:
- Entangled States of Vibration: Experiments have shown that particles can be entangled through vibrational states, suggesting quantum correlations that transcend traditional physical boundaries. This phenomenon opens doors to potential applications in quantum computing and secure communication.
- Quantum Dots and Vibrational Coupling: Researchers have explored how quantum dots exhibit unique vibrational properties, leading to applications in nanotechnology and materials science. The coupling of vibrations with electronic states highlights the potential for energy harvesting at the quantum level.
- Biological Applications: Studies reveal that quantum vibrations play a role in biological processes, from photosynthesis in plants to biochemical reactions in cells. This intertwining of quantum mechanics with biology underscores the importance of further research in this area.
Incorporating these findings into wider scientific knowledge illustrates the interconnectedness of disciplines, paving the way for interdisciplinary research. As experimental techniques continue to evolve, the potential for groundbreaking discoveries in quantum vibrations remains vast.
Applications of Quantum Vibrations
The exploration of quantum vibrations opens up a myriad of possibilities across various disciplines. Their applications could revolutionize technology, enhance scientific understanding, and even offer insight into biological processes. Grasping these applications is crucial for both academic and practical purposes, underscoring the profound implications quantum vibrations have in real-world scenarios.
Quantum Computing and Information
Quantum computing stands as one of the most profound applications of quantum vibrations. This technology hinges on the principles of quantum mechanics, particularly the behavior and interaction of qubits. Unlike classical bits, which are either 0 or 1, qubits can exist in multiple states simultaneously. This property, derived from quantum vibrations, allows quantum computers to process information at astonishing speeds, outperforming classical counterparts for specific tasks.
- Speed and Efficiency: Using quantum vibrations, quantum computers can handle complex calculations much more efficiently. This can be especially vital for areas like cryptography, optimization problems, and molecular modeling.
- Error Correction: Quantum vibrations play a pivotal role in error correction algorithms, enabling systems to maintain their integrity during computations even in the presence of noise.
In short, the core of quantum computing springs from these vibrations, potentially transforming industries, from banking to health sciences, by making processes much faster and secure.
Material Science Innovations
In the realm of material science, the application of quantum vibrations is a game changer. Understanding how materials behave at the quantum level can result in the development of novel materials with unprecedented properties. This is especially relevant in the creation of superconductors and advanced nanomaterials.
- Superconductivity: Quantum vibrations govern the mechanisms that lead to superconductivity, where materials can conduct electricity without resistance. This has significant implications for energy efficiency in electrical systems.
- Nanotechnology: By harnessing quantum vibrations, scientists can manipulate materials at the atomic level, leading to innovations in nanotechnology. This could result in lighter, stronger materials or more effective drug delivery systems in medicine.
These advancements in material science can lead to improved performance in electronics, construction, and medicine, illustrating how deeply quantum vibrations can impact our daily lives.
Biological Implications
The exploration of quantum vibrations does not stop at physics and engineering; it extends into biology as well. There's growing evidence that these vibrations might play a role in various biological processes, which could redefine our understanding of life on a molecular level.
- Photosynthesis: One compelling area of research is how quantum vibrations may contribute to the efficiency of photosynthesis. Plants, through their complex structure, could be utilizing quantum effects to optimize light energy conversion.
- Enzyme Activity: Quantum vibrations might also influence enzyme activity and molecular recognition. This points toward a potential paradigm shift in how we understand biochemical reactions, possibly leading to new methods of drug design or targeted therapies.
The biological implications of quantum vibrations are not merely theoretical; they hold potential for tangible advancements in health, energy production, and our understanding of fundamental life processes.
"Understanding quantum vibrations is like looking through a keyhole into the universe's intricate interplay of energy and matter; the more we explore, the more we realize how interconnected everything is."
Challenges and Limitations
When exploring the intricate web of quantum vibrations, it’s crucial to understand the challenges and limitations inherent in this field. These obstacles are not merely academic; they influence how scientists and engineers approach quantum systems, and thereby, shape the innovations that stem from them. Acknowledging these complexities is essential to fully grasp the ongoing research and potential breakthroughs in quantum mechanics and its related fields.
The Complexity of Quantum Systems
At the heart of the discussion lies the sheer complexity of quantum systems themselves. Unlike classical systems, where variables can often be isolated and measured independently, quantum systems exist in a realm of interdependence. Picture a tightly woven tapestry; pull one thread and you affect the entire fabric. This interconnectedness means that changes in one part of a quantum system can have unpredictable effects on other segments.
For instance, consider particles that are entangled. This unique phenomenon allows two particles to be correlated in such a way that the state of one immediately influences the state of the other, no matter the distance between them. Such complex relationships complicate the predictability of quantum behaviors and challenge traditional modeling methods. Moreover, when researchers try to observe these energetic vibrations, introduces the observer effect — the act of measuring influences the system being measured. This creates an intricate dance of influencing factors where clarity is hard to come by.
"In quantum mechanics, measurement is not merely a passive observation, but an active engagement that alters the very entities one seeks to understand."
This complexity leads to a range of difficulties in deriving conclusive results. For example, theoretical predictions often merge with statistical interpretations, making it tough to ascertain whether observed behaviors are genuine outcomes of quantum vibrations or merely artifacts of measurement processes.
Overcoming Measurement Challenges
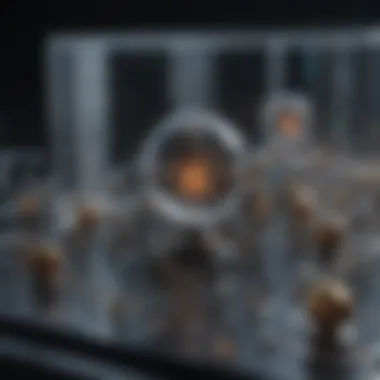
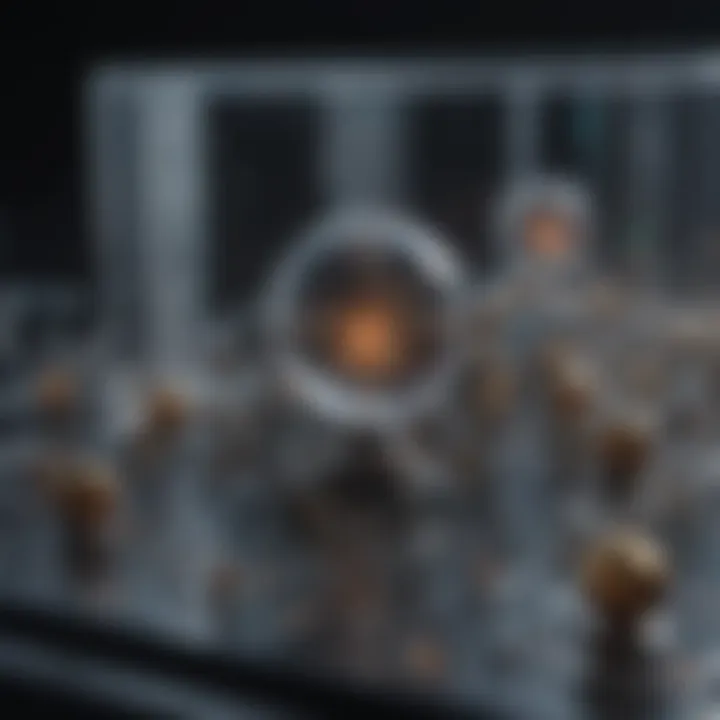
Measurement in quantum systems presents a unique puzzle that requires innovative thinking and advanced technology. Conventional measuring techniques might not apply or may fall short when applied to quantum vibrations. Traditional devices designed to observe macroscopic phenomena often fail to capture the subtleties of quantum behavior, leading to a gap in data and understanding.
One of the more promising technologies in this regard is the development of quantum sensors. These sophisticated instruments are engineered to detect minute changes in quantum states and phenomena. For example, superconducting quantum interference devices (SQUIDs) are used to measure magnetic fields at extremely low levels of sensitivity.
However, the introduction of such technology has its own challenges. High-level sensitivity increases the risk of being affected by environmental noise, thus muddling the data collected. Researchers are actively working on ways to mitigate these effects, such as isolating equipment from external disturbances or employing advanced software algorithms to filter noise.
Future Directions
The exploration of quantum vibrations is a rapidly growing field that promises to reshape our understanding of both energy and matter. As we position ourselves at the cusp of significant scientific breakthroughs, discussing the future directions in this domain reveals not only the potential benefits but also the obstacles that lie ahead. This section will detail the theoretical advancements we can anticipate, alongside the technological applications that could revolutionize various sectors.
Theoretical Advancements
Delving deeper into quantum vibrations means building on established principles and venturing into new theoretical territories. Future advancements could lead to refined models that explain vibrational phenomena with even greater precision. One area of interest involves the implications of quantum entanglement in enhancing our understanding of these vibrations.
Potential paths for theoretical study might include:
- Unified Quantum Theories: Efforts to reconcile general relativity with quantum mechanics may yield a better understanding of vibrations at cosmic scales.
- Enhanced Simulation Models: By leveraging advanced computational techniques, theories regarding quantum behavior can be simulated with unprecedented accuracy, improving predictive capabilities.
- Interdisciplinary Collaboration: Increasingly, physics intersects with fields like biology and materials science. Such collaborations could lead to novel dimensions in our understanding of vibrations.
By pursuing these directions, researchers will uncover not just new theories but also validate existing ones, crafting a richer understanding of the quantum realm.
Potential Technological Applications
The leap from theory to application remains a vital element in the discussion regarding quantum vibrations. Future technological advancements will likely harness quantum principles to develop solutions that are currently beyond our reach. Here’s a look into some promising areas:
- Quantum Computing: As quantum computers evolve, they will rely heavily on the principles of vibrations for better efficiency. New algorithms may emerge, fine-tuning operations that exploit these quantum states for superior processing power.
- Energy Harvesting: By understanding the dynamics of quantum vibrations, scientists may find ways to harness energy more efficiently, tapping into quantum energy fluctuations to power devices.
- Medical Technologies: Techniques utilizing quantum vibrations could transform medical imaging systems, enhancing capacity to detect diseases at their earliest stages.
In a world that's constantly looking for efficiency and innovation, the role of quantum vibrations can not be overstated. These potential applications stand to offer both economic and social benefits, paving the way for advancements that could redefine how we engage with technology.
"The future of quantum vibrations is not just about understanding them; it's about applying that understanding to create real-world solutions."
In summary, as we reflect on the future directions of quantum vibrations, it is clear that this journey is filled with promise. It’s a dynamic space in which theory and practice intertwine, shaping a new landscape of possibility that aligns closely with our ever-evolving technological society.
Closure
The concept of quantum vibrations permeates our understanding of the physical world, serving as a cornerstone in fields ranging from quantum mechanics to innovative technologies. By sewing together the fabric of energy and matter, quantum vibrations make a profound impact not only on theoretical understanding but also on practical applications. Their significance extends to the very core of scientific inquiry, making it essential to grasp their implications fully.
Recap of Key Insights
In summary, this article has discussed several pivotal ideas surrounding quantum vibrations:
- Fundamental Principles: Quantum vibrations are rooted in quantum mechanics; they illustrate the duality of particles and waves.
- Energy States and Harmonies: At the quantum level, energies exist in quantized states, and vibrations of particles can be observed as harmonics, shedding light on atomic and molecular behavior.
- Experimental Techniques: Advances in technology have facilitated the measurement of quantum vibrations, providing clearer insights into their characteristics and implications.
- Widespread Applications: These vibrations influence various disciplines, from developing quantum computers to enhancing material science and unraveling biological processes.
As we connect the dots, it becomes evident that our comprehension of quantum vibrations places us at the forefront of modern science, encouraging innovative thinking and exploration.
Implications for Future Research
The realm of quantum vibrations invites continued inquiry and exploration. Some of the areas that warrant further investigation include:
- Exploring Quantum Entanglement: Understanding how quantum vibrations contribute to entanglement could unlock new possibilities for quantum computing and secure communications.
- Biological Resonance: Research on how quantum vibrations could influence biological processes may lead to breakthroughs in healthcare, diagnostics, and disease treatment.
- Material Innovation: New materials could emerge from a deeper understanding of vibrations at quantum levels, bringing forth more sustainable and resilient technologies.
"The mysteries of quantum vibrations stand not just as puzzles to solve but as keys to unlock the next generation of scientific and technological advancements."
As researchers, educators, and professionals delve deeper into these inquiries, the potential for groundbreaking discoveries expands. Thus, continuing studies on quantum vibrations will not only enhance scientific knowledge but could also redefine technological frontiers, bridging the gap between theoretical understanding and practical advances.
Key Benefits of Including References
- Establishing Authority: Concrete sources validate arguments and theories, lending authority to the content.
- Encouraging Further Exploration: Providing a clear list encourages readers to dive deeper into the subject matter. Not all nuances can be captured within a single article; references act as stepping stones to broader understanding.
- Connecting Disciplines: Quantum vibrations intersect multiple fields — from physics to biology. Citing sources from varied disciplines showcases the interdisciplinary integration of this topic.
Considerations When Using References
- Relevance: The importance of selecting sources that are closely aligned with the topic at hand cannot be overstated. Older materials can offer historical context but may not reflect current advancements.
- Diversity of Sources: Incorporating both primary research articles and reviews ensures a well-rounded perspective and enriches the academic tapestry.
- Accessibility: For readers eager to delve further, references should ideally point to resources that are accessible, whether these are open-access journals or widely available texts.
"In science, the most fruitful discussions often hinge on the insights of others, thus creating a dialogue across time and space."
Emphasizing the importance of references not only reinforces the article's authority but illuminates the intellectual lineage that has contributed to our contemporary grasp of quantum vibrations. It forms a tapestry of knowledge that not only enriches our present understanding but also scaffolds the future inquiries that await in this enigmatic domain.