Quantum Computing: Transformative Potential and Applications
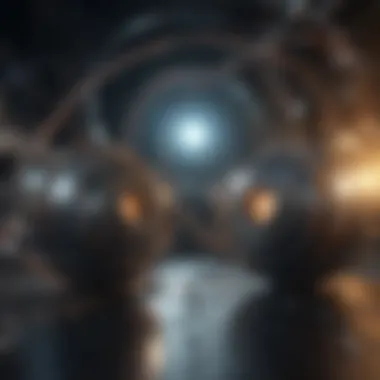
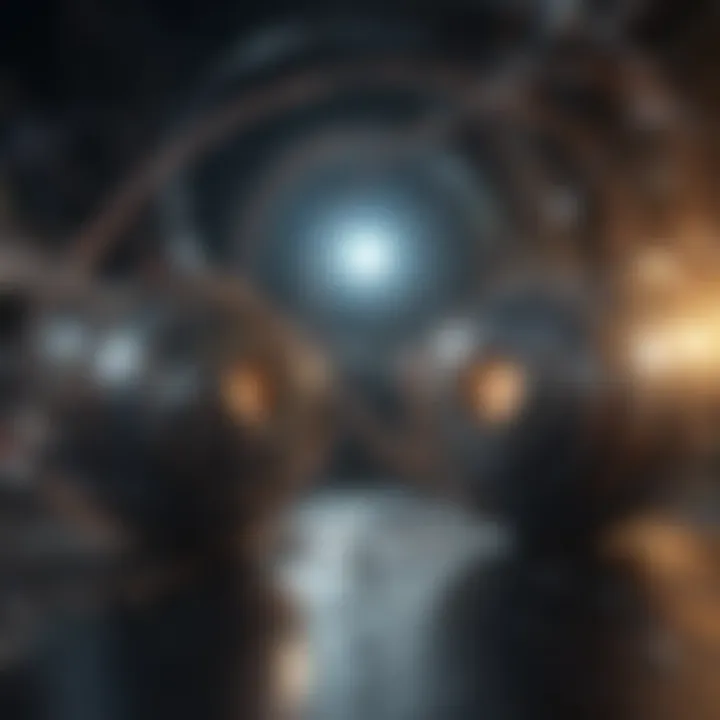
Intro
The rapidly moving world of technology has always sought new avenues of innovation and efficiency. With quantum computing, we're stepping into a realm that promises not just speed but an entirely different approach to problem-solving. At its core, quantum computing harnesses the principles of quantum mechanics to process information in ways that classical computers simply cannot match. This exploration will guide you through the fascinating and complex world of quantum computing, shedding light on how it operates and the impact it could have on various sectors.
Understanding the potential of quantum computers requires a solid grasp of their unique capabilities. They operate using quantum bits, or qubits, which differ markedly from traditional binary bits. While classical bits can only be in one of two states โ 0 or 1 โ a qubit can exist in multiple states at once thanks to a phenomenon known as superposition. This ability enables quantum computers to handle vastly more calculations simultaneously.
Moreover, entanglement, another critical property of quantum mechanics, allows qubits to be linked in ways that transcend classical limitations. When qubits are entangled, the state of one qubit can depend on the state of another, no matter how far apart they are. This interconnectedness facilitates extraordinary computational capabilities.
As we delve deeper, we will uncover the straightforward principles behind these phenomena. From cryptography to optimization problems, quantum computing is poised to disrupt industries in profound ways. The potential benefits are immense, but the hurdles are significant as well. Let's explore some of the key research findings that exemplify the frontier of quantum computing.
Prelude to Quantum Computing
Quantum computing stands at the forefront of a technological revolution, poised to redefine what we understand about computation. Its significance cannot be overstated; we are at a tipping point, where traditional methods falter under the weight of increasingly complex problems. Here, quantum computing steps in as a potential game-changer. It harnesses the oddities of quantum mechanics, promising to tackle challenges that have long eluded conventional computers. This article is an exploration of those unique capabilities - a journey through the strange but fascinating world of qubits and superposition.
The importance of understanding quantum computing lies first and foremost in its implications across various domains. Fields such as cryptography, optimization, and material science are just some of those ready for disruption. As we delve into quantum principles and their applications, we will uncover the ways this technology promises to revolutionize industries and scientific practices alike.
Defining Quantum Computing
At its core, quantum computing leverages the principles of quantum mechanics to process information in ways that classical computers simply cannot. Traditional computing uses bits as the building blocks of information, represented either as 0s or 1s. Quantum computing, however, employs quantum bits or qubits. What sets qubits apart is their ability to exist in multiple states simultaneously, a phenomenon known as superposition. This opens the door to vast computational potential.
Moreover, qubits can also be entangled, meaning the state of one qubit is directly tied to another, no matter the distance apart. This interconnectivity offers a new level of parallelism that allows quantum computers to perform multiple calculations at once - think of it as having an incredibly efficient and mighty brainstorming session where every possibility is explored at lightning speed.
In summary, quantum computing doesnโt just enhance our computational capabilities; it fundamentally alters the nature of what computation means, bridging information theory with the peculiarities of quantum physics.
The Evolution of Computing Technology
The journey of computing technology is a tapestry woven with leaps and bounds. Starting from the rudimentary mechanical calculators of the early 17th century, we moved towards the first electronic computers in the mid-20th century, led by machines like ENIAC. Each evolution set the stage for the next, leading us to microprocessors and the personal computing revolution in the 1980s.
However, classical computers have struck a ceiling in performance with exponential increases in processing requirements. For example, consider complex algorithms in fields like data analytics or artificial intelligence which can demand immense resources. Enter quantum computing, not as a replacement, but as a vital complement, able to solve the unsolvable. Instead of following the traditional linear progression of computing, quantum takes a leap into a realm where calculations can defy the speed limitations of classical architectures.
In this sense, exploring quantum computing offers insights not only into what is possible but also into the future trajectory of technology itself. Experts anticipate that as quantum capabilities grow, theyโll foster new solutions previously thought unattainable. Evaluating this evolution helps frame our understanding of its potential impact today and in the future.
Fundamental Principles of Quantum Mechanics
Quantum mechanics underpins the entire structure of quantum computing. Understanding these fundamental principles is paramount as they elucidate how quantum systems operate uniquely compared to classical systems. It is these principles that allow quantum computers to harness the laws of physics at the smallest scales, ultimately leading to groundbreaking advancements in computation.
Notably, two cornerstone concepts of quantum mechanics stand out: superposition and entanglement. These ideas not only shape the functionality of quantum bits, or qubits, but also define the nature of quantum information itself. Moreover, they set the stage for how these systems can solve complex problems far more efficiently than traditional computing methods.
The relevance of these principles cannot be overstated:
- Enabling Capacity: Quantum principles allow computers to process vast amounts of information at once, leveraging the dual states of qubits. This has implications beyond mere speed; it could redefine what tasks are computationally feasible.
- Revolutionizing Fields: Applications deriving from quantum mechanics have the potential to transform sectors such as cryptography, materials science, and optimization problems, offering significant added value over current technologies.
"Quantum mechanics is the key that unlocks the potential of quantum computing, illuminating a world where computation can be exponentially more powerful."
The leap in understanding quantum mechanics will inevitably dictate how effectively quantum computers can be utilized. As researchers and enthusiasts deepen their grasp of these concepts, a more nuanced appreciation of quantum computing will emerge. Each principle stands as a stepping stone toward more complex systems and greater computational capabilities.
Superposition and Entanglement
Superposition refers to the ability of a quantum system to be in multiple states at once. This stands in stark contrast to classical bits, which can only exist in one of two statesโ0 or 1. For instance, when a qubit is in a state of superposition, it can represent both 0 and 1 simultaneously, allowing quantum computers to explore many solutions at once. This feature is akin to a person flipping through multiple channels on television simultaneously rather than tuning into just one.
Entanglement, on the other hand, describes a peculiar relationship between qubits where the state of one qubit will instantaneously influence the state of another, no matter the distance between them. This interconnectedness can lead to improved performance in computations since multiple qubits can operate in tandem, enhancing computational efficiency and problem-solving capabilities. This phenomenon can be seen in nature as well, akin to two dancers perfectly in sync, moving together regardless of the distance separating them.
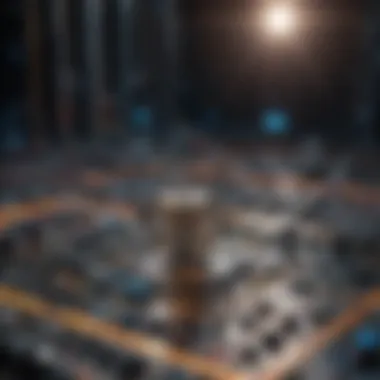
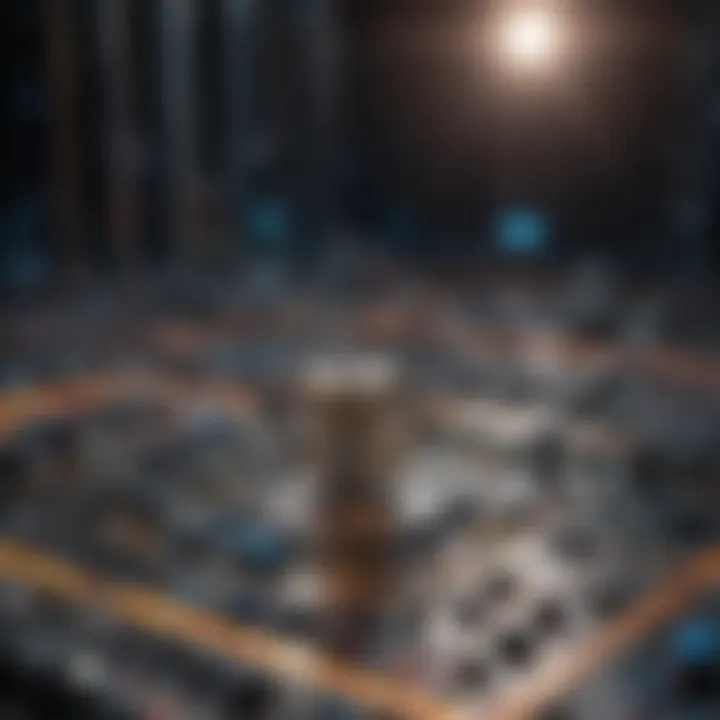
Quantum Bits versus Classical Bits
In classical computing, information is processed using bits, which serve as the basic units represented as either a 0 or 1. These bits work within deterministic frameworks, limiting their computational potential. In contrast, quantum bits, or qubits, leverage the principles of quantum mechanics, allowing them to exist in multiple states and leverage entanglement for complex processing.
Key differences include:
- Representation: A classical bit is binary, while a qubit is typically conceptualized as a sphere, where any point on the surface corresponds to a possible state.
- Processing Power: Due to superposition, qubits can perform multiple calculations at once, exponentially increasing processing power when large numbers of qubits are involved.
- Information Retention: Classical bits can be stable yet limited. Qubits, however, are subject to decoherence; they lose their quantum state quickly due to interactions with their environments, making error correction and fault tolerance crucial for practical applications.
As such, as we delve deeper into the world of quantum computing, these fundamental principles are not mere academic concepts. They are the bedrock that will enable us to envision and construct the quantum systems of the future.
Applications of Quantum Computing
Quantum computing stands at the forefront of technological innovations with its unique applications redefining various sectors. These capabilities extend far beyond what classical computers can achieve, offering solutions for problems previously deemed unsolvable. The exploration of these applications showcases the potential that quantum computing holds, not only to streamline processes but also to revolutionize fundamental practices across different domains.
Cryptography Advancements
Quantum Key Distribution
Quantum Key Distribution (QKD) represents a significant leap forward in secure communications. At its core, QKD ensures that any attempt to eavesdrop on the communication inherently alters the key, alerting the parties involved. This characteristic is fundamental because it utilizes the principles of quantum mechanics to create a framework where any unauthorized access is immediately detected.
The appeal of QKD lies in its capacity to provide unparalleled security in transmitting sensitive information. Unlike traditional methods, which can be compromised over time, quantum keys are practically unbreakable due to their quantum nature. However, implementing QKD can pose challenges, such as the distance limitations and the need for specialized infrastructure to support quantum signals effectively.
"In a world where security is paramount, QKD offers a promising fortress against currents of cyber threats."
Impact on Current Encryption Standards
With advancements in quantum computing, established encryption standards face unprecedented threats. Commonly used encryption methods, such as RSA and AES, rely on the difficulty of specific mathematical problems, which can be efficiently solved by quantum algorithms like Shorโs algorithm. This realization sparks urgency in rethinking encryption protocols to withstand the onslaught of quantum capabilities.
The critical aspect here is not just the obsolescence of current standards, but the necessity to upgrade them to quantum-resistant alternatives. While this transition presents a unique advantage to bolster security, it also poses significant challenges in maintaining the balance between security and accessibility. The adoption of new standards must come quickly, but it must also ensure ease of implementation for users across various platforms.
Optimization Problems
Logistics and Supply Chain
In logistics and supply chain management, optimizing every aspect is crucial for efficiency and cost-effectiveness. Quantum computing can address complex optimization problems that traditional algorithms struggle with. These problems often involve multiple variables and constraints, making them difficult to solve in a reasonable time frame on classical systems.
The key characteristic of using quantum computing in this realm is the ability to explore many potential solutions simultaneously. This uniquely positions businesses to capitalize on efficiencies in routing, inventory management, and resource allocation. Yet, the challenge remains in integrating these quantum solutions into existing infrastructure, which can require a complete overhaul of current systems and practices.
Finance and Portfolio Optimization
Portfolio optimization is another field where quantum computing is set to make waves. Investors constantly strive to maximize returns while minimizing risks, a balance that involves analyzing vast amounts of data. Here, quantum algorithms can unveil improved approaches to finding optimal asset allocations swiftly.
The allure of quantum computing in finance lies in its potential to analyze numerous market conditions instantaneously and provide actionable insights. However, from a practical standpoint, integrating quantum solutions into existing financial models presents challenges. The complexity of financial markets, coupled with the need for real-time data processing, raises questions about viable implementation strategies and the technologyโs ability to deliver tangible results in a fast-paced environment.
Quantum Simulations
Chemical Reactions
When it comes to chemical reactions, quantum simulations can furnish scientists with the ability to model and predict outcomes at an unprecedented level of detail. Traditional computational methods struggle to capture the intricate interactions of particles at a quantum level. Quantum computers, however, can simulate these reactions with higher accuracy, potentially leading to groundbreaking discoveries in pharmaceuticals and materials.
The distinctive feature of using quantum simulations here is their ability to replicate the behavior of molecules and their interactions under various conditions. This characteristic allows for faster and potentially cheaper research and development cycles. Nevertheless, challenges still loom over the need for advanced quantum hardware and algorithms tailored specifically for chemical applications.
Material Science
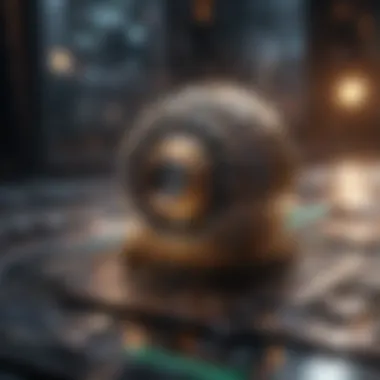
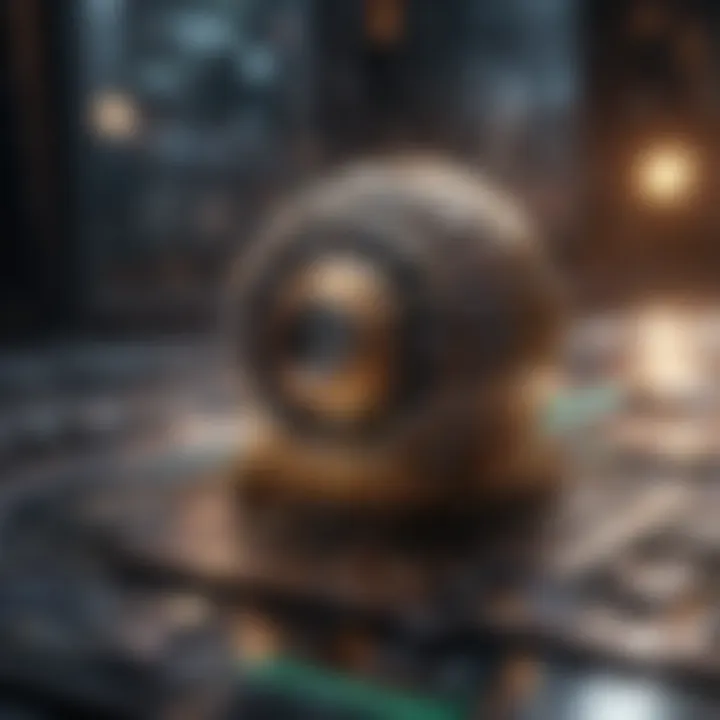
In material science, quantum computing enables researchers to design and understand new materials with desirable properties. The ability to simulate molecular structures and predict their behavior plays an invaluable role in innovation from superconductors to nanomaterials. Projects leveraging quantum simulations in material science are paving the way for advancements that can lead to more efficient and sustainable materials.
The unique benefit of this application is the depth of insight gained into atomic arrangements and electronic properties, allowing scientists to tailor materials to specific applications. But just like other fields, the reliance on cutting-edge quantum technology presents hurdles that researchers must overcome, such as hardware limitations and the need for interdisciplinary collaboration to translate quantum findings into practical applications.
Exploring quantum computing's various applications showcases the technology's transformative potential across industries. From enhancing security frameworks in cryptography to optimizing supply chains and reimagining chemistry and material science, quantum computers are steering us into an era of unprecedented possibilities.
Current Research and Developments
The strides made in the realm of quantum computing are not just fascinating; they're pivotal to its emergence as a groundbreaking technology. The significance of current research and developments extends far beyond just theoretical discussions. What we see today is the fruit of immense collaborations between academics, industries, and research institutions across the globeโall working toward a shared goal: unlocking the potential of quantum devices.
These advancements help shape the future of various fields ranging from cybersecurity to pharmaceuticals, and itโs essential to grasp their impact. By comprehending the paramount roles that companies and academic institutions play, we can gather critical insights that might drive the next wave of innovation.
Itโs not just about the engineering feats but also the societal repercussions that these developments entail. Researchers are not merely hunting for the next big advancement; they are addressing significant issues like the potential disarray in privacy laws and shifts in job markets as quantum technologies thrive.
Leading Quantum Computing Companies
In the race toward quantum supremacy, several key players have emerged, each contributing uniquely to the ecosystem of quantum computing. Companies like IBM, Google, and D-Wave are at the forefront, pushing the boundaries of what quantum machines can achieve. For instance, IBM has developed the Qiskit framework, enabling users to experiment with quantum algorithms on their quantum processors; itโs a step that democratizes access to quantum technology.
- IBM - Their Quantum Experience platform allows users to run experiments on actual quantum processors.
- Google - Known for achieving quantum supremacy in 2019 with its Sycamore processor, highlighting the practical possibilities of quantum technology.
- D-Wave - Focuses on quantum annealing, specializing in optimization problems that classical computers struggle with.
- Rigetti Computing - A rising star that builds quantum processors and provides cloud access to quantum computing through Forest.
These companies exemplify the fusion of innovation and collaboration. They often share their findings and challenges, creating an environment where growth is collective rather than isolated. This cooperative spirit bridges the gap between idea and implementation, encouraging a flow of knowledge that is vital for the fieldโs advancement.
Academic Contributions to Quantum Algorithms
Academia plays an irreplaceable role in propelling quantum computing forward. Universities and research institutions worldwide are exploring complex quantum algorithms, fueling the body of knowledge that supports practical applications. This academic groundwork is where theoretical models crystallize into operational algorithms.
Various institutions are not just theorizing; they're putting theory to the test, pushing the limits of whatโs known about quantum mechanics. For example, research teams from institutions like MIT and Stanford have delved into optimization algorithms, finite-element simulations, and even quantum version of machine learning techniques.
"The ultimate goal is to solve problems that are currently intractable for classical computers."
Moreover, the contribution of publications and collaborative research networks significantly influences the progression of this field. Open-source initiatives thrive in universities, allowing researchers across the globe to access quantum software tools and datasets, leading to novel findings that might one day pave the way for real-world applications.
In summary, the landscape of current research and developments in quantum computing is dynamic and multifaceted. The synergy between corporations and academic institutions enriches the discourse surrounding quantum technology, creating pathways for innovation that may redefine various aspects of our lives. As we observe these interactions, we gain a clearer view of the challenges and opportunities that lie ahead in this revolutionary domain.
Challenges in Quantum Computing
The journey into the realm of quantum computing is not all smooth sailing. Amid the excitement and potential, several challenges loom large, demanding attention and resolution. Addressing these challenges is critcal not just for scientists and tech enthusiasts but also for various stakeholders of the society, as they hold implications that can reshape our future in profound ways. Tackling these hurdles means navigating through the intricate web of technical limitations and ethical considerations. Let's delve into the specifics.
Technical Hurdles
Error Rates in Quantum Operations
One of the most pressing technical hurdles in the world of quantum computing is the error rates in quantum operations. Unlike classical computers, where errors can often be corrected with redundancy, quantum computers operate on quantum bits, or qubits. These qubits are delicate and influenced by external factors, which can lead to errors in calculation. The key characteristic of qubit error rates is that they are significantly higher compared to traditional bits. This is a vital consideration because low error rates are essential for reliable computations.
The challenge of error management is further compounded by the phenomenon known as decoherence, where quantum information is lost to the environment. This unique feature underscores the importance of developing robust error correction algorithms tailored for quantum contexts. While attempts have been made to enhance these error rates, the underlying physics presents a tricky puzzle. In essence, high error rates can hinder the reliability of quantum computations, thereby affecting their practical utility.
Scalability Issues
Scaling quantum computers presents another significant challenge in the field. As one seeks to build more powerful quantum machines, the issue of scalability comes into sharp focus. The key characteristic here is that adding more qubits isnโt as straightforward as stacking bricks. Ensuring that additional qubits function harmoniously with existing ones increases the complexity of both hardware and software. This intricate dance of qubits brings along its own issues, as more qubits result in heightened error rates and technical demands.
Moreover, designing scalable quantum architecture that can support increased qubit counts while maintaining operational efficiency remains a daunting task. The unique feature of scalability issues lies in the delicate balance that must be maintained: increasing power and potential without escalating complications. Currently, these scalability challenges pose a significant barrier to realizing the full potential of quantum computing.
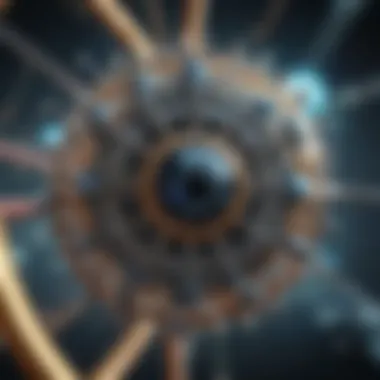
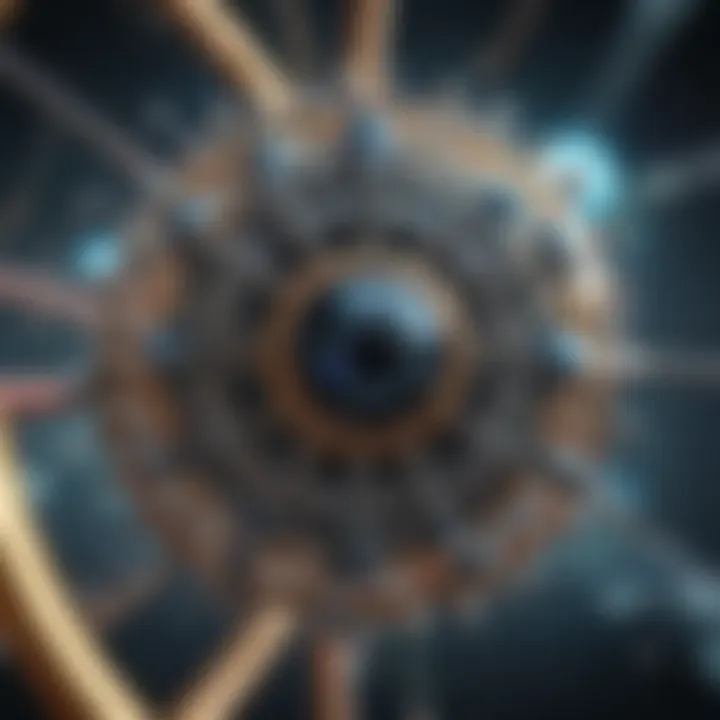
Ethical Considerations
Implications for Privacy
Jumping from the technical problems, we now arrive at the ethical side of quantum computing. The implications for privacy are profound. Quantum technologies have the potential to break current cryptographic systems, which many rely upon for securing sensitive data. The key characteristic of quantum computingโs impact on privacy is its ability to facilitate rapid decryption of classical data through quantum algorithms. This has raised substantial concern among privacy advocates and technologists alike.
The unique feature surrounding these implications is the dual nature of quantum capability. While it threatens existing privacy measures, it also enables development of new, more secure quantum encryption methods. These advancements could alter our current understanding of data security. Therefore, while the challenge is formidable, it also invites innovation and the need for re-evaluating our practices.
Impact on Employment
The impact of quantum computing on employment represents a critical intersection of technology and society. The rapid advancement of this field brings the potential for significant job displacement across various sectors. The key characteristic of this challenge is that the proficiency demanded by quantum technologies often exceeds the current skill sets available in the workforce. As industries adapt and evolve, there may be an increasing demand for a skilled workforce trained in quantum theories and practical applications.
The unique feature of this situation is that it not only poses risks of job loss but also presents opportunities for new job creation in emergent sectors focused on quantum technologies. The balance of these potential outcomes creates a dynamic landscape ripe for further scrutiny. As we forge ahead, it becomes imperative to consider targeted educational initiatives and programs that can equip the workforce with necessary skills amidst this technological shift.
The challenges in quantum computing are not merely obstacles; they are also catalysts for innovation and dialogue that could redefine the landscape of technology and society.
Future Prospects of Quantum Computing
Future prospects of quantum computing offer a window into how this technology might reshape the very landscape of computation, reshuffling our understanding of data processing and problem-solving. The potential breakthroughs on the horizon โ coupled with the interaction between quantum systems and classical computing โ set the stage for a computing paradigm that could redefine efficiency, speed, and even security across many sectors.
Potential Breakthroughs
The world of quantum computing brims with anticipation regarding its potential breakthroughs. Not only is there the promise of speed that could far exceed what classical computers can manage, but there are also specific areas where quantum capabilities might negatively impact current limitations.
- Drug Discovery: One of the most exciting potentials lies within the realm of pharmaceuticals. Quantum computers have the ability to model complex molecular structures far more efficiently. With this capacity, future breakthroughs in medicinal treatments for diseases that currently resist treatment could be realized.
- Artificial Intelligence: The integration of quantum computing in AI could facilitate far swifter data analysis, enabling intelligent systems to process and learn from vast datasets almost instantaneously. Imagine algorithms running in a fraction of the time they currently do, unlocking new possibilities in fields like machine learning.
- Material Science: As researchers continue to push the envelope in material discovery, quantum computers could simulate the properties of new materials at an atomic level, leading to lighter, stronger, or more conductive materials. This has implications for everything from electronics to construction.
- Climate Modeling: Tackling climate change requires complex modeling that current computers struggle with due to their limitations. Quantum computing can provide new models that factor in a multitude of variables efficiently, leading to better predictions and ultimately informed policymaking.
In essence, these breakthroughs signal a shift from theoretical possibilities to practical, hands-on advancements. Itโs expected that within a decade, we might see pivotal transformations in how we approach technology and its integration into society.
Integration with Classical Computing
Despite the glossy promises of quantum computing's potential, it's critical to understand that it won't replace classical computing entirely. Rather, both systems will find ways to work in tandem, complementing one anotherโs capabilities. Thus, integration becomes an essential topic in future discussions.
- Hybrid Systems: As researchers explore ways to harness the strengths of both computing paradigms, hybrid systems are emerging. For instance, quantum processors might address specific complex tasks while classical systems handle general processing. The classic and quantum worlds can communicate, leveraging the best of both.
- Software Development: The development of quantum software must align closely with classical systems. New programming languages and paradigms are being invented that facilitate this interactionโlike Q# from Microsoft. Ultimately, tools that allow programmers to seamlessly integrate quantum capabilities into existing software infrastructures will prove invaluable.
- Business Applications: Companies like IBM and Google are already trialing ways to integrate quantum computing into their existing business models. This includes developing applications for supply chain optimization, financial modeling, and more, where classical computing handles routine operations while quantum solutions deal with complex problems.
The End and Implications
The journey through the world of quantum computing reveals not just its capabilities, but its potential to reshape entire industries and redefine our understanding of computation. The significance of understanding the implications of this technology cannot be overstated. As we press on into an era where quantum computers become more commonplace, we must consider both the advantages they offer and the challenges they bring.
Key benefits seen from quantum computing include accelerated problem-solving abilities, particularly in areas traditionally plagued by computational bottlenecks. Whether in optimizing complex supply chains or modeling intricate chemical reactions, the ability of quantum systems to handle vast amounts of data at unprecedented speeds is nothing short of revolutionary.
However, this also comes with considerations that society must grapple with. The evolution of quantum capabilities may lead to vast changes in job landscapes, as roles that can be automated by quantum algorithms replace traditional positions. Similarly, in the realm of cryptography, while quantum computers promise enhanced security methods, they also threaten established encryption techniques, prompting a reevaluation of how we safeguard our data.
"The key to the future of computing lies not just in the machines, but in our approach to using them responsibly and ethically."
In effect, understanding these implications becomes paramount for students, educators, researchers, and professionals alike.
Summary of Key Insights
Throughout this examination, several pivotal insights have emerged:
- Unique Capabilities: Quantum computers utilize principles like superposition and entanglement which allow them to process information in ways classical computers cannot.
- Transformative Applications: Fields such as cryptography, finance, supply chain management, and material science stand to benefit immensely from advancements in quantum computing technologies.
- Evolving Landscape: The research landscape is rapidly changing, with enterprises and academic institutions pushing boundaries in quantum algorithm development and quantum hardware improvements.
In summary, it's clear that as we look to the future, understanding the nuances and potential impacts of quantum computing is essential for effectively navigating its integration into our world.
Call for Continued Research
The exploration of quantum computing must not end here. Continuous research is critical to unlocking its full potential. Here are some points to consider as we move forward:
- Investment in Education: Preparing the next generation of scientists and engineers to work on quantum technologies will be crucial. Educational institutions need to introduce specialized programs that cater to these emerging fields.
- Public-Private Partnerships: Collaboration between governments, academia, and private sectors can drive innovation, ensuring that these technologies are developed in alignment with societal needs.
- Ethical Framework Development: As with any major technological advance, the establishment of ethical standards and guidelines is necessary. This must include considerations around privacy, security, and job displacement.