Exploring Quantum Computers: Foundations and Future
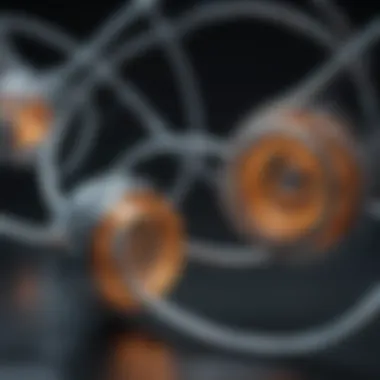
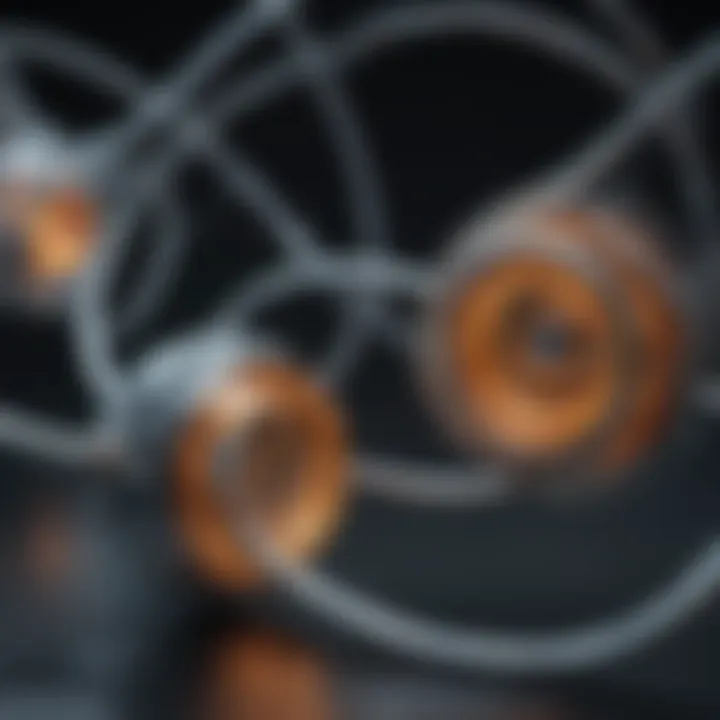
Intro
In the ever-evolving landscape of technology, quantum computing stands out like a lighthouse on a foggy night, guiding researchers, students, and tech enthusiasts alike toward a future teeming with potential. The basic idea revolves around harnessing the peculiar properties of quantum mechanics to process information in ways that classical computers simply can't match. While hinting at the promise of faster computations, the intricacies behind quantum systems are both fascinating and complex, constantly opening up new avenues for exploration.
Key Research Findings
As quantum computing continues to advance, researchers have unearthed several compelling insights that not only propel the field forward but also challenge our understanding of computation as a whole.
Overview of Recent Discoveries
In recent years, significant breakthroughs have occurred, particularly in the areas of quantum algorithms and error correction. For instance:
- Quantum Supremacy: Google declared quantum supremacy in 2019, demonstrating that their quantum processor could perform tasks that would take a classical supercomputer thousands of years.
- Development of Quantum Algorithms: New algorithms like Grover's and Shor's have gained attention for their capability to dramatically enhance problem-solving efficiency in specific scenarios.
- Error Correction: Progress in quantum error correction techniques has been vital. Researchers are developing methods to handle noise and decoherence, ensuring that quantum information can be reliably processed and transmitted.
These advancements signify not only a leap in technology but also a deeper comprehension of the quantum realm.
Significance of Findings in the Field
The implications of these discoveries extend well beyond the walls of academia. They offer:
- Practical Applications: Enhancements in cryptography, material science, and drug discovery are just the tip of the iceberg.
- New Paradigms of Understanding: As researchers push boundaries, they are beginning to seek answers to questions long considered out of reach, such as the quantum behaviour of complex systems.
By comprehensively digesting these advancements, professionals across various domains can appreciate the transformative effects this technology might have in the coming years.
Breakdown of Complex Concepts
This technology can appear daunting, but understanding the fundamentals behind it can pave the way for fruitful discussions and innovative applications.
Simplification of Advanced Theories
Quantum mechanics fundamentally challenges classical logic—where ordinary bits are either 0 or 1, quantum bits (qubits) can exist in multiple states simultaneously due to superposition. This notion of existence in multiple states is what enables quantum computers to solve certain problems much quicker than traditional machines. Moreover, the phenomenon of entanglement allows qubits that are entangled to affect each other's states instantly, no matter the distance separating them.
Visual Aids and Infographics
To better grasp these concepts, various infographics detail:
- The structure of quantum computers and how qubits are organized.
- The differences in processing information between quantum and classical computers.
Engaging with these visuals can demystify complex theories and elevate our understanding of quantum mechanics.
"Quantum computing is not just the next chapter; it is a whole new book in the field of computing science."
As we navigate through this intriguing world of quantum computing, it becomes imperative to keep our minds open and critical, for the future is indeed intertwined with the threads of quantum technology.
Preamble to Quantum Computing
In today's rapidly evolving technological landscape, the significance of quantum computing stands tall, drawing attention from various fields such as science, industry, and even regular life. As we leaped deeper into the 21st century, the quests for faster computing power led us to explore realms beyond classical systems. Understanding the fundamentals of quantum computing is no longer an academic exercise; it's pivotal for anyone eyeing the future of technology, from budding students to seasoned professionals.
The core of quantum computing lies in its ability to handle complex problems that classical computers struggle with. Instead of traditional bits that hold a value of either 0 or 1, quantum computers utilize quantum bits or qubits, which can exist in multiple states simultaneously due to the principle of superposition. This unique characteristic means quantum machines have the potential to solve intricate calculations at lightning speed, making them indispensable, especially in challenging domains like cryptography and materials science.
The advantages of diving into quantum computing aren't just confined to technical prowess. They extend to ethical considerations, the need for careful regulation, and an evolution in how we perceive computational problems. In the face of potential worldwide issues—like climate change and disease modeling—quantum computing offers the means to simulate and analyze vast data sets in ways we haven't achieved yet.
Key Points about the Intro to Quantum Computing:
- Conceptual Leap: Quantum computing represents a shift in computing paradigms, emphasizing the need for new practices.
- Real-World Relevance: Its capabilities can significantly impact healthcare, finance, and environmental studies, showcasing the need for knowledge across sectors.
- Future Employment: A sound understanding of quantum computing opens doors to numerous career opportunities as the demand for skilled professionals in this area grows.
"Quantum computing is not just a niche field; it is becoming the cornerstone for future technological innovations."
Equipped with a foundational understanding of what quantum computing encompasses, we delve into its definition and historical backdrop. This exploration provides a clearer context of where we stand today and what possibilities lie ahead.
Fundamental Principles of Quantum Mechanics
Understanding quantum mechanics provides the bedrock upon which quantum computing stands. It’s not just a theoretical construct but rather, a framework that reshapes how we perceive computation, using nature’s own rules to solve complex problems. The principles of quantum mechanics—superposition, entanglement, and quantum interference—are not merely academic; they have profound implications for technology and practical applications. Each of these key concepts revolutionizes our approach to information processing, allowing quantum computers to tackle challenges current systems cannot.
Superposition
Superposition is an extraordinary principle that asserts a quantum system can exist in multiple states simultaneously. For instance, unlike a classical bit, which can only be a 0 or 1, a qubit can be both at the same time. Picture a spinning coin—while it's in motion, it’s neither heads nor tails, but instead a blend of both outcomes. This duality enhances the computing capacity exponentially. It’s as if the coin, while still spinning, is able to calculate untold possibilities, enabling quantum computers to perform operations at unprecedented speeds. Thus, when combined with other qubits in superposition, the potential outcomes multiply like rabbits during spring.
Entanglement
Entanglement, on the other hand, captures a truly mind-bending aspect of quantum mechanics. When qubits become entangled, the state of one qubit instantly influences the state of another, regardless of the distance separating them. This principle is akin to a pair of gloves; if you find one glove in the drawer, you instinctively know the other will fit the opposite hand, even if it's in a box across town. This interconnectedness allows incredibly fast and complex computations to occur, since entangled qubits can share information at astonishing speeds. This leads quantum computing into an entirely different realm of efficiency compared to classical systems.
"Entangled qubits can instantly influence each other, embodying a level of interconnectedness that classical bits can't approach."
Quantum Interference
Finally, we arrive at quantum interference, the phenomenon that enables quantum algorithms to amplify the right answers while canceling the wrong ones. Imagine waves in an ocean, where some waves combine to create towering heights while others drown each other out. Similarly, quantum interference manipulates probabilities, enabling certain solutions to crystalize while obliterating others. Through clever design of quantum circuits, algorithms harness interference to optimize solutions further, trimming down the chaff from the wheat, leading to more precise outcomes. This capability is what allows quantum computers to tackle complex problems—like simulating molecular interactions or optimizing logistical challenges—in ways that are simply unmanageable using classical methods.
In summary, the fundamental principles of quantum mechanics are not just theoretical musings; they serve as the driving forces behind quantum computing. With superposition allowing simultaneous processing, entanglement creating connected networks of information, and quantum interference enhancing accuracy and efficiency, these principles lay down a robust foundation for what could be a transformative technology in the years to come.
Quantum Bits: Qubits
Quantum bits, or qubits, serve as the foundational building blocks of quantum computing. Unlike classical bits, which exist in a binary state of either 0 or 1, qubits can exist in a state of superposition, holding both values simultaneously. This unique trait enables quantum computers to process a vast amount of information in parallel, setting them apart from the limitations of classical computing. The importance of qubits lies in their ability to harness the strange and puzzling properties of quantum mechanics, allowing for complex calculations that classical bits simply cannot handle. The richer information capacity translates to exponentially faster processing speeds for specific tasks, making qubits the heart of quantum algorithms and applications.
Classical Bits Versus Qubits
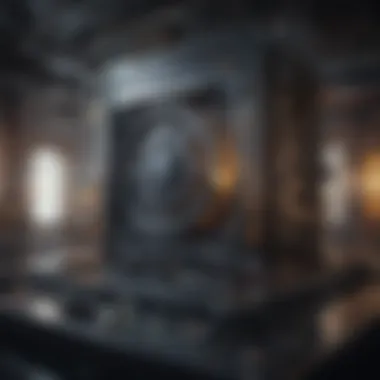
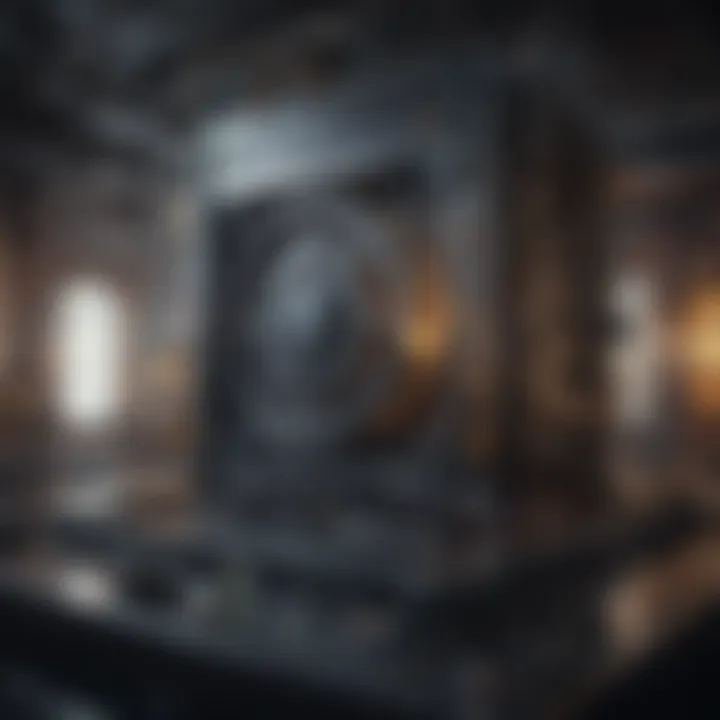
The distinction between classical bits and qubits is a cornerstone of understanding quantum computing. Classical bits function in a clearly defined manner—they sit firmly in either the 0 or 1 category. In contrast, qubits are like a coin spinning in the air, representing a blend of both states until measured. This property can be compared to a person being both awake and asleep until someone checks. Furthermore, when two or more qubits become entangled, they maintain a correlation regardless of the distance separating them. This interconnectedness can lead to substantial increases in computational power, making qubits a critical element in advancing quantum technology.
Types of Qubits
Different physical implementations of qubits bring along unique strengths and weaknesses. Understanding these types of qubits is essential for grasping how quantum computers operate under the hood. The three notable types are superconducting qubits, trapped ion qubits, and topological qubits, each offering distinct methodologies and operational frameworks.
Superconducting Qubits
Superconducting qubits are noteworthy for their advantage in speed and scalability. These qubits are typically formed from circuits made of superconducting materials cooled to extremely low temperatures. One of the key characteristics of superconducting qubits is their ability to be manipulated with microwave signals, making them extremely fast and efficient. A compelling feature of superconducting qubits is their relatively simple integration with existing semiconductor technologies, facilitating advancements in quantum hardware development. However, challenges such as decoherence—a phenomenon where quantum information is lost—still loom large for sustaining their operation over longer time frames.
Trapped Ion Qubits
Trapped ion qubits use ions that are cooled and confined electromagnetically. They offer an impressive level of precision when it comes to entangling and measuring qubit states. The notable characteristic here is their long coherence times, which can sustain quantum states without interference for a longer duration than many other types of qubits. This makes them a popular choice in research settings. However, the complexity in scaling up trapped ion systems can become a barrier, as creating and manipulating multiple ions in an arrangement simultaneously is technically demanding.
Topological Qubits
Topological qubits represent a more recent leap in qubit technology, drawing on the principles of topological states in matter. The key characteristic of topological qubits lies in their potential to encode information in a way that is more robust to environmental disturbances. This inherent stability is particularly appealing for the long-term preservation of quantum information. Their unique feature includes braiding operations that protect qubit states from noise, which could potentially mitigate some of the issues faced by other qubit types. However, the conception and realization of topological qubits is still in a nascent stage, leaving questions about their overall practicality in widespread applications.
"Understanding the intricacies of qubits is essential for grasping the revolutionary potential of quantum computing. Each type of qubit brings unique capabilities to the table, shaping the future landscape of technology."
As the field of quantum computing continues to grow and evolve, the exploration of these varying types of qubits will likely play a pivotal role in determining the course of technology and practical applications.
Quantum Gates and Circuits
Quantum gates and circuits lie at the heart of quantum computing, functioning analogously to classical logic gates but with a vastly increased complexity and capability. They not only manipulate quantum bits, or qubits, but also enable quantum algorithms to perform tasks that classical computers struggle with. Understanding the intricacies of quantum gates, as well as the architecture of quantum circuits, is essential for anyone interested in the future of computational technology.
The significance of quantum gates and circuits can't be overstated. They serve as the building blocks for quantum algorithms, allowing for operations that capitalize on quantum mechanics' peculiarities such as superposition and entanglement. Unlike traditional gates, which only manage binary states of 0s and 1s, quantum gates facilitate operations on qubits, which can be in multiple states simultaneously. This characteristic dramatically increases the computational power and efficiency of quantum systems.
Understanding Quantum Gates
Quantum gates perform specific operations on qubits. Each gate can be visualized as a transformation that alters the state of the qubit in a well-defined way. For instance, the Hadamard gate takes a qubit in state |0⟩ and transforms it into a superposition of
This gate exemplifies how quantum gates differ from classical gates by producing probabilities rather than definitive outcomes.
There are several fundamental quantum gates, each with unique functionalities:
- Pauli-X Gate: Flips the state of the qubit, much like a classical NOT gate.
- CNOT Gate: A control gate that flips the state of a target qubit based on the state of a control qubit.
- Phase Gate: Alters the phase of a qubit's state, impacting the interference patterns in computations.
This variety is not just for show; the choice of gate can significantly influence the performance and outcome of quantum algorithms. When using quantum gates, every meticulous adjustment counts, as outcomes hinge on the subtleties of quantum mechanics.
Architecture of Quantum Circuits
Building a quantum circuit involves arranging quantum gates into a logical sequence to execute complex computations. Picture it as a maze, where each gate acts as a junction leading you to multiple potential pathways. The challenge lies in qubit coherence and maintaining entanglement through the circuit, ensuring that the necessary quantum state is achieved at each stage.
Consider the following elements that contribute to an effective quantum circuit architecture:
- Qubit Connectivity: The ability to connect multiple qubits is crucial. If qubits can't interact effectively, the circuit's performance will suffer.
- Depth of the Circuit: This refers to the number of gates in sequence. A circuit that is too deep can introduce errors due to decoherence.
- Modularity: Designing circuits in a modular fashion can simplify complex computations, allowing for easier troubleshooting and adjustment.
Creating quantum circuits isn’t just about throwing gates together. It requires thoughtful design, anticipating potential choke points in computation.
As quantum computing progresses, understanding gates and circuits will prove instrumental in pushing the limits of what these machines can achieve. The drive for more efficient algorithms hinges on efficient gate architectures and the interplay between them.
Algorithms in Quantum Computing
Algorithms play a pivotal role in the realm of quantum computing. They harness the power of quantum bits, or qubits, to deliver solutions that classical algorithms struggle with. Understanding these algorithms is critical because they can dramatically alter the landscape of computing and problem-solving as we know it. What's more, they can address problems over a vast array of fields, from cryptography to optimization, making them a cornerstone of future technological advancements.
Quantum algorithms utilize the peculiar properties of quantum mechanics, allowing them to perform complex computations in a fraction of the time taken by their classical counterparts. As such, they can lead to significant speedups in processing that could reshape industries. Furthermore, they provide a framework for researchers to explore solutions that were previously thought unattainable.
Key aspects to consider when discussing quantum algorithms include their efficiency and adaptability. Unlike classical algorithms that might be rigid and tailored to specific problems, quantum algorithms can evolve and be modified, translating across various sectors. This flexibility offers tremendous potential for innovations in industries ranging from pharmaceuticals to finance.
Shor’s Algorithm
Shor's Algorithm stands out as one of the most influential algorithms in quantum computing. Developed by mathematician Peter Shor in 1994, this algorithm addresses the factorization of large integers efficiently. The significance of Shor's Algorithm lies in its implication for current encryption methods. Classical computing methods, which rely on the complexity of factorization, could be quickly rendered obsolete by Shor's prowess. The threat it poses to traditional RSA encryption requires a shift towards post-quantum cryptography as higher security measures are needed.
The algorithm functions by reducing the problem of factorizing an integer into manageable components, leveraging quantum techniques such as the quantum Fourier transform. In essence, while it might take classical computers an impractical amount of time to factor large integers, Shor’s method can handle it seamlessly, leading to potential vulnerabilities in current cryptographic systems.
Key Procedures of Shor’s Algorithm:
- Modular Exponentiation: This forms the backbone of Shor's, enabling calculations needed for effective factorization.
- Finding Periods: The identification of the period of a function is crucial and is conducted with quantum efficiency.
- Classical Post-Processing: Following the quantum computations, traditional methods are employed to derive the factors from the quantum output.
“If we can harness the capabilities of Shor's Algorithm, we might be able to crack codes that stand as pillars of our digital security.”
Grover’s Algorithm
Grover's Algorithm offers a different taste of what quantum computing can accomplish. Proposed by Lov Grover in 1996, it focuses on searching unsorted databases with remarkable efficiency. The essence of Grover's lies in its quadratic speedup over classical search algorithms. Where classical algorithms may require evaluating on the order of N items, Grover’s can locate a target item in roughly √N steps.
This efficiency is achieved through the use of quantum superposition and interference, effectively allowing multiple possibilities to be explored simultaneously. Grover's Algorithm can reshape data retrieval practices across diverse domains. For example, in cybersecurity, with a need for rapid search capabilities to spot vulnerabilities or in artificial intelligence for faster pattern recognition in large datasets.
Main Features of Grover's Algorithm:
- Superposition: Allowing multiple states to be considered at once, thereby accelerating the search process.
- Amplitude Amplification: A critical step in enhancing the probability of measuring the desired result, making it a powerful tool even when the target is minuscule in size.
In summary, both Shor’s and Grover’s algorithms illustrate how quantum computing isn't just a theoretical concept but a foundational pillar ushering in a new era of computing possibilities. The journey in quantum algorithms is merely beginning, with prospects of discovering even more transformative calculations ahead.
Comparing Classical and Quantum Computing

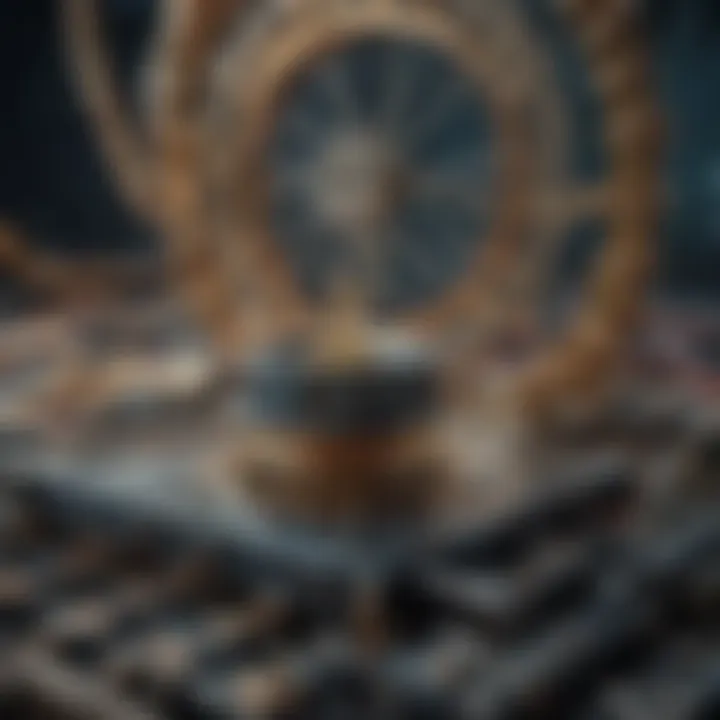
In the ever-evolving landscape of technology, understanding the differences between classical and quantum computing is not just an academic exercise; it holds profound relevance for researchers, practitioners, and educators. This comparison is crucial for grasping the potential of quantum systems to outperform classical methods in specific applications. The advent of quantum computing brings with it promises of breakthroughs in areas such as cryptography, complex problem solving, and simulations—domains where traditional computers often hit a wall. Today, we’ll dive into two primary areas of focus: processing speed and problem-solving capabilities, both of which starkly underline the possibilities and limitations of classical versus quantum paradigms.
Processing Speed
One undeniable aspect where quantum computing shines is in processing speed. At its core, classical computing relies on binary bits, processing information as either 0s or 1s. While effective for a myriad of tasks, this fundamentally limits the computing power available for complex operations. In contrast, quantum computers harness the power of qubits which can exist in a state of 0, 1, or both simultaneously (thanks to superposition). This significant difference opens the door to breathtaking gains in performance for certain computational tasks. For instance, consider a search problem; a traditional approach may require inspecting each item one by one, which is a laborious process. On the other hand, a quantum approach could evaluate many possibilities at once, slashing the time necessary for solutions.
"Quantum computers can potentially solve certain problems in seconds that would take classical computers thousands of years."
To better understand this, let's break it down:
- Algorithms: Quantum algorithms like Grover’s showcase impressive speed improvements for searching unsorted databases, utilizing qubits’ unique properties to reduce the time complexity.
- Parallelism: Quantum systems inherently leverage parallelism, unlike their classical counterparts which follow a sequential processing model.
- Scalability: As quantum technology develops, the potential for scaling up processing speed without linearly increasing power consumption is astonishingly appealing.
Problem-Solving Capabilities
When it comes to problem-solving, the abilities of quantum computers can not be overstated. Traditional computers excel at performing calculations and processing data, but they sometimes struggle with more abstract forms of reasoning—especially in cases involving vast datasets or complex systems. Quantum computing presents a paradigm shift, particularly in addressing problems in areas such as logistics, pharmaceuticals, and even AI.
For instance, consider the drug discovery process. Classical methods typically simulate the interactions of molecules one at a time. Conversely, quantum systems can analyze multiple interactions at once, potentially leading to discoveries that classical methods might miss altogether. This ability to perform complex simulations is essential in fields that require a deep understanding of intricate variables.
Some key considerations in this domain include:
- Complexity Handling: Quantum algorithms are designed to tackle problems with exponential complexity, efficiently managing relationships in large datasets.
- Optimization Problems: Quantum solutions provide means to optimize multi-variable problems much more expediently than classical computers often can.
- Machine Learning: In artificial intelligence, quantum computing models can provide enhancements that are capable of outperforming classical algorithms in training processes.
By taking a closer look at processing speed and problem-solving capabilities, it's clear that quantum systems bring fresh perspectives to computational problems that have long stymied classical approaches. As researchers continue to unravel the intricacies of quantum mechanics and its application in computing, the implications for the modern world could be transformative.
Current Quantum Technologies
The realm of quantum computing is rapidly evolving, pushing the boundaries of what we once thought was possible. It’s not just a matter of theory anymore; significant strides in hardware and software are transforming academic inquiries into practical applications, making this topic vital for anyone invested in the future of technology. Understanding current quantum technologies can provide insight into both the present landscape and future potential, revealing how they might alter industries from medicine to financial services.
Hardware Developments
Hardware plays a crucial role in quantum computing, as it's the foundation upon which all functionalities rest. The primary focus here is on building reliable and scalable quantum systems that can execute complex computations far beyond the capacity of classical computers.
There are several noteworthy advancements in hardware developments, including:
- Superconducting Circuits: Companies like IBM and Google are employing superconducting circuits that utilize Josephson junctions. These junctions allow for incredibly low resistance, meaning they can operate at near zero energy loss. This helps in achieving high-speed computations and is crucial for the coherence times needed for effective quantum processing.
- Trapped-Ion Systems: Using lasers to manipulate charged particles, or ions, trapped in electromagnetic fields is another path being explored. Research institutions, like the University of Colorado, are investigating this technology. The beauty lies in the precise control these ions offer, enabling them to act as qubits and expand computational capabilities.
- Photonic Technologies: Light particles, or photons, are still another avenue being investigated for their ability to easily transmit data over distances. Companies such as Xanadu are on the cutting edge of this area, developing quantum chips with unique optical properties.
Hardware innovations are essential not simply because they amplify computational power but also because they lay the groundwork for future systems that integrate quantum AI and quantum networking.
Advancements in quantum technologies are often different from traditional computing. Recognition of these differences is vital to understanding both their weaknesses and advantages. For example, maintaining qubit coherence is constantly at odds with environmental interactions, known as decoherence. Research continues to focus on reducing these interactions and enhancing the overall reliability of quantum systems.
Software Platforms
Alongside remarkable hardware, the emergence of robust software platforms is equally essential to the maturation of quantum computing. The software ecosystem determines how effectively these advanced hardware systems can be utilized. With software tools that cater to quantum algorithms, the aim is to bridge the gap between complex theories and practical applications.
Current software developments include:
- Quantum Operating Systems: Platforms such as IBM’s Qiskit and Microsoft’s Quantum Development Kit allow researchers and developers to create algorithms that can run on quantum computers. These tools enhance accessibility to quantum technology, promoting experimentation and innovation.
- Frameworks for Algorithm Design: Libraries designed explicitly for quantum computing, such as PennyLane and Cirq, simplify the complex task of creating and implementing algorithms. These frameworks reduce the entry barrier for software developers wanting to contribute to the field without deep quantum physics expertise.
- Simulators: Before actual quantum machines can be utilized, simulators allow for the testing and evaluation of quantum algorithms in a more classical environment. Tools such as Qiskit Aer enable developers to simulate quantum circuits and gauge their performance without the need for extensive resources.
The ripple effect of advancements in quantum software stretches across multiple sectors, propelling forward various industries such as finance, drug discovery, and optimization problems. Early implementation and adaptations can lead to profound competitive advantages for companies willing to invest in these technologies.
Therefore, by understanding hardware and software developments, one can appreciate how they complement each other in driving the future of quantum computing. As these technologies progress, we inch closer to a world where complex problems can be solved far quicker than before, fundamentally altering the landscape of computation as we know it.
Challenges Facing Quantum Computing
The journey into quantum computing is full of promise but comes laced with a myriad of challenges. This section aims to dissect those obstacles that researchers and technologists encounter on the path toward harnessing quantum mechanics for practical applications.
One of the most critical facets of the conversation surrounding quantum computing has to do with its fragile nature. Quantum systems are sensitive to their environments, which leads us to a key challenge: decoherence.
Decoherence
Decoherence is perhaps one of the toughest nuts to crack in the realm of quantum computing. In layman's terms, it refers to the loss of quantum coherence, where the superposition of different quantum states becomes entangled with the environment, leading to an observable classical behavior. Imagine a delicate ballet performance interrupted by a loud noise; it disrupts the flow, much like how decoherence disrupts quantum processes.
The implications of decoherence are profound. Quantum bits (qubits) can lose their quantum properties quickly, resulting in errors. This poses significant barriers when attempting to run complex computations or maintain quantum states over time. It hampers the scalability of quantum systems, making it difficult to create a quantum computer that can outperform classical ones consistently.
- Environmental Interference: Factors such as temperature fluctuations and electromagnetic radiation can lead to decoherence.
- System Design: Developing systems with better isolation from environmental noise is critical.
Researchers are actively exploring methods to mitigate decoherence, including the use of quantum error correction techniques and isolated qubit designs. These advancements might pave the way for a new era in quantum computing, allowing us to harness these fragile particles rather than being held back by their limitations.
Error Correction
Following the understanding of decoherence, another essential challenge that needs addressing is error correction. In classical computing, error correction is a well-established discipline, but quantum error correction relies on completely distinct principles.
Due to the aforementioned fragility of qubits, traditional methods of error correction often fall flat. When a quantum state collapses, pinpointing the exact nature of that error becomes elusive. Here’s where quantum error-correcting codes come into play. These codes employ entanglement uniquely to safeguard quantum information while simultaneously performing error correction.
Even though these codes show promise, they require many physical qubits to protect a single logical qubit. Such redundancy leads to a substantial increase in the resource requirement, presenting further challenges. Thus, the quest for effective quantum error correction algorithms is ongoing.
The road to quantum computing is riddled with challenges, yet each step forward advances us closer to a technological revolution.
As these challenges are met, we can expect a clearer vista of the possibilities ahead in quantum computing, enhancing both our theoretical understanding and practical applications.
Implications for Cybersecurity
The relationship between quantum computing and cybersecurity paints a picture of both potential threats and innovative solutions. As quantum machines gear up to redefine computational boundaries, the dynamics surrounding data security enter murky waters. Understanding these implications is crucial not just for tech experts but also for organizations and individuals who rely on robust cyber defenses.
Threats to Traditional Encryption
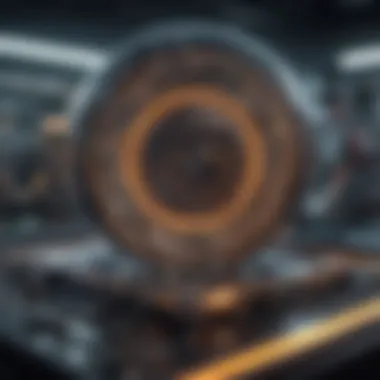
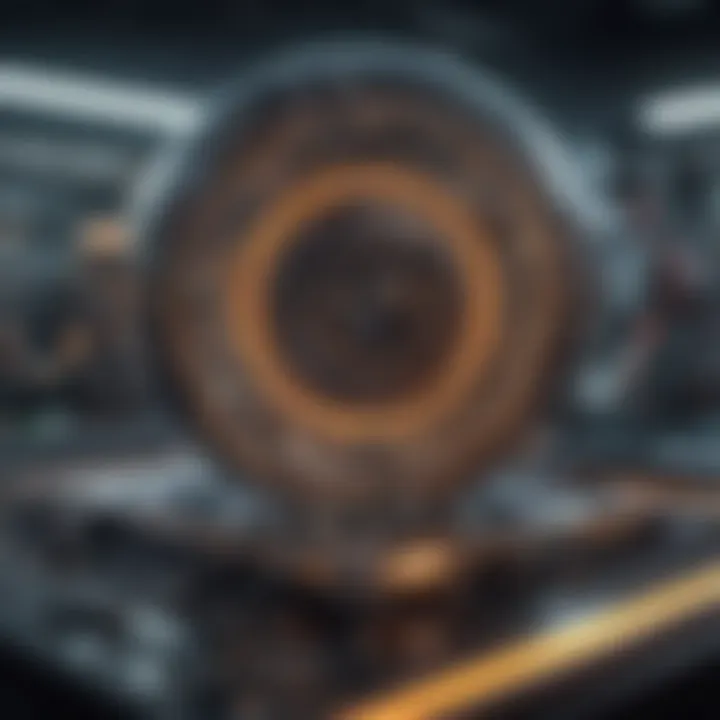
Quantum computers wield a capability that could pose significant challenges to current encryption schemes. Traditional encryption methods, such as RSA (Rivest–Shamir–Adleman) and ECC (Elliptic Curve Cryptography), rely on the computational difficulty of certain mathematical problems. The classic case is that of factoring large prime numbers, which, under normal circumstances, would take traditional computers ages to solve. However, when confronted with a quantum computer running Shor’s Algorithm, the situation changes dramatically.
"Shor’s Algorithm can factor large integers in polynomial time, rendering many of today’s encryption methods virtually useless should quantum computing become mainstream."
This newfound efficiency poses a direct threat to national security, finance, and private communication. Sensitive information that is encrypted today could become vulnerable tomorrow. The ramifications are vast; imagine a scenario where encrypted databases get decrypted overnight. Organizations may find themselves facing dire consequences from compromised data integrity and confidentiality.
Moreover, as devices and IoT (Internet of Things) proliferate, the invisibility of quantum threats becomes alarming. One might not even know their toaster is communicating sensitive data that could be unencrypted in the near future. Here’s what key concerns are emerging:
- Data Breach Risks: Potential loss of personal data due to quantum attacks could lead to identity theft or financial fraud.
- Infrastructural Vulnerabilities: Systems integral to power grids and health care could fall prey to attacks, undermining national security.
- Long-term Data Concerns: Information that needs to be secure for years could be exposed as quantum capabilities increase over time.
Post-Quantum Cryptography
In light of the threats posed by quantum computing, the field of post-quantum cryptography emerges as a beacon of hope. This area of research focuses on developing cryptographic systems that can resist quantum attacks. Unlike traditional systems that might crumble, post-quantum systems are built on mathematical challenges that are difficult for both classical and quantum computers to solve.
Several proposals are bouncing around the community, aiming to replace or supplement existing encryption methods. Examples of these projects include lattice-based cryptography, hash-based signatures, and multivariate polynomial equations, all designed with quantum resistance in mind.
- Lattice-Based Cryptography: This method makes use of high-dimensional lattices, which are hard for quantum computers to decipher.
- Hash-Based Signatures: By focusing on the properties of hash functions, these signatures provide a secure means of authentication even in a quantum landscape.
- Multivariate Cryptography: This approach is based on solving systems of equations with multiple variables, which quantum machines struggle with.
The race is on to standardize post-quantum cryptographic algorithms. Organizations such as NIST (the National Institute of Standards and Technology) are actively engaged in this quest, leading the charge to create protocols suitable for the looming quantum era. The implications of adopting robust post-quantum solutions are far-reaching:
- Enhanced Security: Businesses can guard against potential data breaches with encryption that withstands quantum scrutiny.
- Reassured Communication: Private and governmental communications can remain secure, thereby stabilizing trust in relational contracts.
- Reduced Transition Troubles: By preparing now for quantum credentials, organizations lessen the shock of future compliance and security mandates.
In summary, the implications of quantum computing for cybersecurity are profound and far-reaching. Understanding the dual nature of these advancements—threat and opportunity—will allow stakeholders to prepare, adapt, and thrive in a shifting technological landscape.
Applications of Quantum Computing
The realm of quantum computing is not only a fascinating field of study but is also rapidly moving toward practical applications that can reshape industries. From pharmaceutical research to financial modeling, the benefits of quantum computing are on the precipice of being realized. These applications harness the unique strengths of quantum mechanics, offering tools and methodologies that far surpass classical computing capabilities. This section explores three pivotal areas where quantum computing stands to make significant impacts: pharmaceuticals, financial modeling, and artificial intelligence.
Pharmaceuticals
The pharmaceutical industry stands at the forefront of the quantum revolution. Traditional drug discovery processes can take years, sometimes decades, accompanied by exorbitant costs. This is largely due to the complexity of molecular interactions and the sheer number of potential compounds to investigate.
Quantum computing, with its ability to model molecular behavior with exceptional accuracy, presents a solution to these challenges. With algorithms that exploit quantum superposition and entanglement, researchers can simulate and analyze the properties of drug molecules in ways that were previously unimaginable.
For example, quantum computers can analyze protein folding, leading to the identification of compounds that can potentially target diseases more effectively. This has enormous implications for tackling everything from cancer to infectious diseases, offering the promise of more effective treatments developed in shorter timeframes. Moreover, the optimization of drug formulations can be vastly improved, potentially allowing the industry to reduce waste and lower the ecological footprint.
Financial Modeling
In the financial sector, quantum computing could very well be the ace in the hole. With markets that fluctuate at incredible speeds and vast amounts of data to analyze, conventional systems struggle to keep pace. Quantum algorithms can process complex datasets at lightning speed, granting financial analysts the ability to predict market movements more accurately.
Investments in quantum technology promise to enhance risk assessment strategies as well. Merging probabilistic models with quantum computing can lead to better scenario analysis—helping organizations forecast the regulatory, economic, and market changes with remarkable precision. As a result, institutions may find themselves better equipped to handle financial crises or capitalize on market opportunities before their competitors do.
Artificial Intelligence
Artificial intelligence (AI) aims to mimic human cognition, but its capabilities may soon be bolstered by quantum computing. Neural networks, the backbone of many AI applications, require extensive computations to learn from data. Quantum algorithms can potentially expedite these calculations, making machine learning processes significantly more efficient.
Moreover, pattern recognition and optimization problems—core aspects of AI—can be addressed using quantum technologies. As quantum systems can represent and manipulate vast datasets more effectively, they unlock the potential for developing advanced AI applications that can learn, adapt, and evolve much faster than traditional AI systems. This could benefit various sectors, from autonomous vehicles to personalized marketing strategies.
As quantum technologies continue to mature, their applications could redefine not just specific industries but indeed the entire digital landscape.
In summary, quantum computing is poised to offer groundbreaking solutions across various domains of human activity. From speeding up drug discovery in pharmaceuticals to enhancing financial forecasting and advancing artificial intelligence, its potential applications are as vast as they are exciting. As research and development progress, staying abreast of these advancements becomes crucial for those engaged in these sectors.
Future Prospects of Quantum Computing
The future of quantum computing stands as a pivotal frontier, stirring a myriad of discussions in the realms of technology, science, and economic potential. This domain is not merely a theoretical construct; it is quickly morphing into a practical reality. Its importance within this article is substantial, focusing on the multifaceted elements that make quantum computing an electrifying prospect.
Commercialization
The journey toward the commercialization of quantum computing is already in the works, with companies like IBM, Google, and D-Wave leading the charge. For stakeholders across diverse industries, the implications of commercial quantum computing are manifold:
- Enhanced Processing Power: Businesses are keen on harnessing quantum's capabilities to solve problems traditional computers can't handle efficiently, such as optimization tasks in logistics and complex simulations in biochemical research.
- Cost Reduction: Initially perceived as an expensive endeavor, advancements in quantum network infrastructure will likely lower costs over time. As technology matures and becomes more accessible, even small enterprises may harness quantum solutions.
- New Market Opportunities: Quantum technologies open up venues for entirely new sectors. For instance, quantum-enhanced machine learning can lead to breakthroughs in sectors like personal finance, predictive analytics, and cybersecurity.
The race has begun, yet it requires concerted effort among businesses, researchers, and policymakers to create an ecosystem that fosters innovation.
"Quantum technology will, in the not-so-distant future, redefine what's feasible in computation and beyond."
Exploration of New Algorithms
Another critical aspect of the future of quantum computing is the exploration of new algorithms tailored to leverage the unique capabilities of quantum systems. Just as classical computing evolved predominantly through algorithm development, quantum computing is expected to follow a similar trajectory. Here are a few notable points:
- Optimization Algorithms: One of the primary areas of interest is the development of algorithms that exploit quantum superposition and entanglement for faster optimization solutions. This could lead to advancements in fields like logistics, supply chain management, and financial modeling.
- Quantum Machine Learning: There's an ongoing exploration of algorithms that integrate machine learning principles with quantum computing. These quantum machine learning algorithms aim to analyze data sets at unprecedented speeds, potentially revealing insights that classical systems could miss.
- Quantum Simulation: Algorithms specifically designed for simulating quantum systems are being developed. This work has profound implications for chemistry and materials science, enabling rapid testing and optimization that could lead to new materials and drugs.
As these algorithms and technologies develop, they will redefine computational paradigms and create a profound impact on diverse fields—spanning from the arts to pure sciences.
The path ahead for quantum computing is laden with challenges but glimmers with promise, paving the way for innovative advancements in our understanding of computation.
Finale
The exploration of quantum computing is not merely an academic endeavor; it carries significant implications for multiple fields, significantly enhancing the discourse surrounding cutting-edge technologies. The importance of this topic in the context of the article lies in its potential to revolutionize how computing is approached, optimizing processes that were once deemed infeasible under classical paradigms.
Summarizing Key Insights
Throughout the article, various elements of quantum computing have been unpacked. Let’s reflect on the critical insights:
- Fundamental Principles: Understanding of superposition, entanglement, and quantum interference establishes the foundation on which quantum computing stands.
- Qubits: The transition from classical bits to qubits reflects a paradigm shift in information processing, showcasing how quantum technology operates
- Quantum Algorithms: Algorithms such as Shor’s and Grover’s exemplify the superiority of quantum computing in specific computational tasks, hinting at transformative potential.
- Applications: Sectors such as pharmaceuticals, finance, and artificial intelligence unveil pathways where quantum technologies can deliver significant advancements.
- Future Prospects: As we lean towards commercialization and research into new algorithms, the landscape of computing appears vibrant with possibilities.
Looking Ahead
Peering into the horizon of quantum computing, the future may hold more than just technical innovations; it promises a rethinking of processes and solutions.
- Rapid Advancements: Continuous developments in hardware and software underpinning quantum technologies could lead to breakthroughs that are hard to imagine today.
- Interdisciplinary Collaboration: As this field grows, collaborations across disciplines will be crucial. Scientists, engineers, and industry leaders must join forces to navigate the intricate challenges ahead.
- Ethical Considerations: With immense power comes responsibility. The societal implications of quantum computing, particularly in security and privacy, demand thoughtful consideration and strategic frameworks.
In essence, the conclusion is not just an endpoint; it is a gateway to further inquiry, discussion, and implementation of quantum computing that beckons the interests of students, researchers, educators, and professionals alike.