Unveiling the Physics Behind Computer Technology
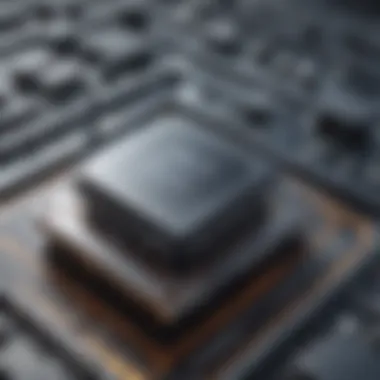
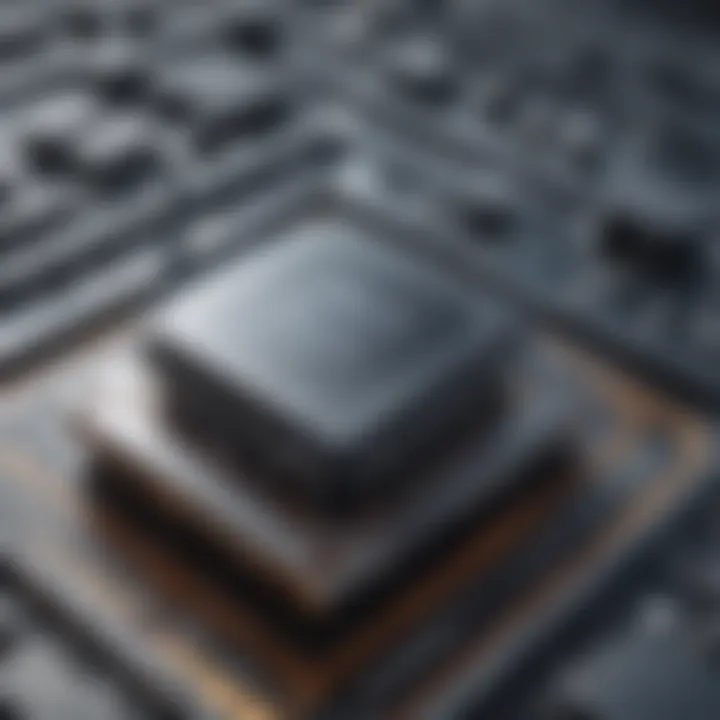
Intro
The role of physics in computer technology is both profound and intricate. At its core, computing relies on the manipulation of physical materials and energy. This dependence forms the basis for understanding how computers function, evolve, and integrate into our daily lives. By examining the principles of physics, we can appreciate how components like semiconductors and electromagnetic waves operate. These elements are not just technical concepts; they are the backbone of modern computing.
This article sets out to demystify these fundamental principles. Delving into topics ranging from the physics of matter to the complexities of quantum mechanics, we aim to provide clarity to learners, educators, and enthusiasts alike. Through an exploration of key research findings and complex concepts, we will uncover how these scientific ideas shape the world of computing.
Key Research Findings
Overview of Recent Discoveries
Recent advances in the field of computer physics have led to significant discoveries. Innovations in semiconductor materials have transformed the functionality and efficiency of computers. Researchers have identified new materials, such as graphene and transition metal dichalcogenides, which promise to enhance speed and reduce power consumption. These breakthroughs are not only theoretical but have practical implications in the design of next-generation electronics.
Moreover, the exploration of quantum computing has opened new avenues for processing information. The principles of superposition and entanglement have made it possible to perform calculations at unprecedented speeds. Several significant studies have demonstrated that quantum algorithms can solve specific problems much faster than classical ones.
Significance of Findings in the Field
The findings in both semiconductor research and quantum mechanics carry great weight in computer science. They challenge traditional paradigms and encourage a re-evaluation of existing designs. For instance, as the limits of classical computing approaches, these discoveries highlight the need for new approaches in information processing. This aligns with the ongoing quest for more powerful and efficient computing systems.
"Understanding the physics behind innovations is crucial for the next wave of technology." - An Expert in Computational Physics
Breakdown of Complex Concepts
Simplification of Advanced Theories
Complex theories such as quantum mechanics and semiconductor physics can be daunting. To simplify these concepts, it helps to break them down into their core components. For instance, when discussing semiconductors, one can focus on the basic idea of electron mobility and the significance of doping materials.
Likewise, in quantum mechanics, the fundamental concept of superposition can be explained through relatable examples. If we picture a bit being both 0 and 1 simultaneously in a quantum context, it becomes easier to grasp its implications for computational speed.
Visual Aids and Infographics
Visual aids play a critical role in enhancing understanding. Infographics that illustrate the behavior of electrons in a semiconductor can provide clarity. Diagrams showing quantum states in different configurations can help demystify the intricacies of quantum computing. For effective learning, combining textual information with visuals can reinforce the overarching themes presented.
In summary, the physics of computers is not merely a niche subject but rather a cornerstone of technological advancement. By appreciating these fundamental principles, one gains a better understanding of the intricacies that define modern computing.
Foreword to the Physics of Computers
Computers have become an integral part of modern life. They influence everything from communication to healthcare, education, and even entertainment. But to understand how computers work, one needs to delve into the underlying principles of physics that govern their operation. Physics provides a framework for understanding the behavior of materials and energy that computers rely on.
This section introduces the fundamentals of the physics behind computing. It emphasizes why these concepts are essential for students, educators, and professionals in the field. Selected principles, such as the properties of semiconductors and the characteristics of electromagnetic waves, play critical roles in the functioning of electronic devices. Being aware of these helps in the design and innovation of new technologies in the computer field.
Defining the Physics of Computing
The physics of computing encompasses a variety of topics that explain the interactions between electronic components. At its core, it involves understanding how matter and energy behave at microscopic levels, which directly influence the design and performance of computer systems.
Key areas include:
- Quantum Mechanics: Governs the behavior of particles at small scales, important for understanding semiconductors and quantum computing.
- Electromagnetism: Deals with the interactions of charged particles, significant for data transmission and signal processing in computer networks.
- Thermodynamics: Concerns heat and energy transfer, which is crucial in managing heat generated by computer operations.
By defining these concepts, we lay a solid foundation for how they contribute to computer technology.
Importance in Modern Technology
The relevance of physics in computer technology cannot be overstated. Understanding these principles leads to several benefits:
- Innovation: Grasping the principles allows for the development of cutting-edge technologies, such as faster processors and more efficient memory storage.
- Problem Solving: Knowledge of physical laws aids in troubleshooting hardware and software issues. Engineers who understand these principles can identify potential problems earlier and devise effective solutions.
- Interdisciplinary Applications: Physics integrates with disciplines like computer science and electrical engineering. This collaboration enhances solutions in diverse fields, from artificial intelligence to renewable energy solutions.
The impact of these principles is evident in everyday applications, making the study of physics in computing a vital area for anyone involved in technology.
"The innovations of tomorrow build upon the physics of today. Understanding these fundamental principles is key to progressing in computing technology."
Overall, this introduction sets the stage for deeper exploration into each significant component that drives the physics of computers.
The Role of Electromagnetism
Understanding electromagnetism is crucial for grasping how computers function in contemporary society. Electromagnetic principles govern numerous processes in computing, including the transmission of data and the functioning of components. These principles provide the framework for developing technologies that communicate and operate effectively.
Understanding Electromagnetic Waves
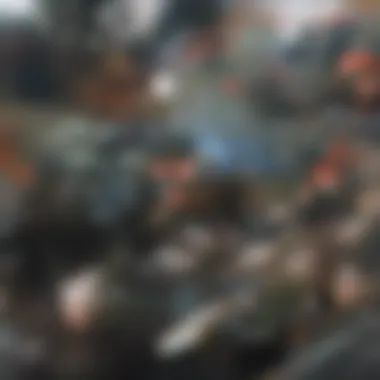
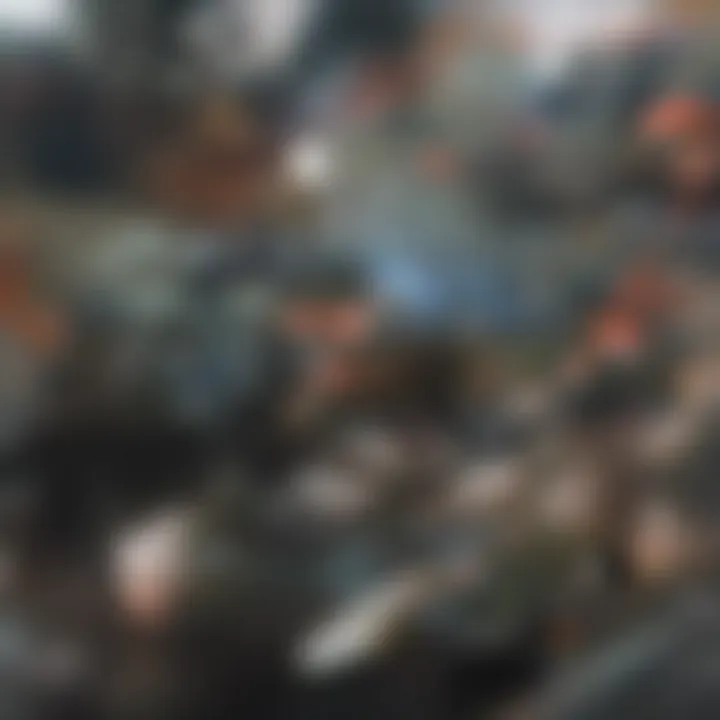
Electromagnetic waves are essential for transmitting information wirelessly. At its core, electromagnetic radiation consists of oscillating electric and magnetic fields. These fields propagate through space, transferring energy as they travel. A key aspect of electromagnetic waves is their diverse range of frequencies, which makes them suitable for various applications.
High-frequency waves, such as microwaves and radio waves, enable wireless communication systems. They facilitate everything from mobile phone signals to Wi-Fi connections, allowing for rapid data transfer across vast distances. The design of antennas and receivers is heavily based on the characteristics of these waves.
"Electromagnetic waves are the backbone of modern wireless communication, seamlessly connecting devices across the globe."
The utilization of electromagnetic principles also influences how signals are encoded and transmitted. Signal modulation techniques, such as amplitude modulation (AM) and frequency modulation (FM), play a crucial role in ensuring clarity and efficiency in data transmission. Understanding these concepts is vital for those studying computer networks and telecommunications.
Applications in Computer Networks
Electromagnetic waves are integral to computer networks as they enable the transfer of data. In practice, various technologies utilize these waves to maintain communication between devices. For instance, Ethernet cables transmit data through electrical signals, whereas fiber-optic cables rely on light waves. Each method has its advantages.
- Transmission Speed: Fiber optics can transmit data at much higher speeds than electrical wires.
- Distance: Radio waves allow communication over much longer distances compared to wired solutions.
- Interference: Wireless systems may face more interference than wired systems, impacting data integrity.
Wireless networking technologies, such as Wi-Fi and Bluetooth, leverage electromagnetic waves for seamless connectivity. These systems offer flexibility and convenience, enabling devices to communicate without physical connections.
In total, understanding the role of electromagnetism provides a comprehensive view of how data is transferred and processed in computer networks. This knowledge is indispensable for students and professionals working with networking technologies.
As we explore further in this article, the foundational concepts surrounding electromagnetism will continuously intersect with various aspects of computer science, revealing the interconnected nature of these disciplines.
Semiconductors and Their Properties
Semiconductors play a crucial role in modern computing. They are the foundational materials for most electronic devices, enabling the functionality of transistors, diodes, and integrated circuits. Their importance stems from their unique properties that allow them to conduct electricity - but only under certain conditions. This section will explore the basic principles of semiconductor materials and their significance in the field of computer physics.
Material Science Basics
At a basic level, semiconductors are materials that have a conductivity between conductors, like metals, and insulators, such as rubber. Common semiconductor materials include silicon and germanium. The atomic structure of these materials includes four electrons in their outer shell, allowing them to form covalent bonds in a crystalline lattice. This structure gives rise to semi-conductive properties.
Key points about semiconductor materials include:
- Band Gap: The energy difference between the conduction band and the valence band. A small band gap allows for easier excitation of electrons.
- Intrinsics: Pure semiconductor materials exhibit low conductivity. Their conductivity improves when impurities are added.
- Temperature Sensitivity: The conductivity of semiconductors is significantly affected by temperature. As temperature increases, more electrons gain enough energy to jump to the conduction band.
The material science of semiconductors is an interdisciplinary study that combines physics, chemistry, and engineering. Understanding these properties is fundamental for anyone looking to delve into computer technology.
Doping and Electronic Properties
Doping is the process of adding impurities to a semiconductor to change its electrical properties. This technique is vital in creating n-type and p-type semiconductors, which vastly differ in their charge carrier types.
- N-type: When elements such as phosphorus or arsenic are added, they provide extra electrons. This creates an abundance of negatively charged carriers, facilitating improved conduction of electricity.
- P-type: Conversely, when elements like boron are introduced, they create holes or absence of electrons, leading to positively charged carriers. The movement of these holes also contributes to electric current.
The benefits of doping include:
- Increased conductivity: Doping significantly enhances the electrical conductivity of semiconductors.
- Control over properties: By adjusting the amount and type of dopants, engineers can fine-tune the electronic properties of the semiconductor to meet specific application requirements.
- Enhanced performance: Doped semiconductors enable the creation of devices that operate more efficiently, which is essential for modern computing needs.
Transistors: Building Blocks of Computers
Transistors play an essential role in the realm of computing. They are fundamental components that allow for the control and amplification of electrical signals within a device. Transistors replace previously used vacuum tubes and have enabled the miniaturization of technology. Without them, modern computing as we know it would not exist.
The advantages of transistors include their small size, energy efficiency, and reliability. They consume significantly less power compared to other technologies, which leads to longer battery life in portable devices. Additionally, their switch-like behavior allows for more complex computations and operations to be performed with greater speed and accuracy.
Another consideration when discussing transistors is their role in integrated circuits. Many transistors can be combined on a single chip, leading to powerful processors that drive everything from smartphones to supercomputers. Hence, understanding transistors is crucial for grasping how information is processed within computers.
Types of Transistors
Transistors come in various types, each with distinct characteristics and applications. The two primary types are bipolar junction transistors (BJTs) and field-effect transistors (FETs).
- Bipolar Junction Transistors (BJTs): These transistors utilize both electron and hole charge carriers. They are widely used for amplifying signals and have high current-carrying capability. BJTs can operate in various modes, including active, cutoff, and saturation. Their design is simple, making them relatively easy to use in a range of applications.
- Field-Effect Transistors (FETs): FETs depend primarily on electric fields to control the flow of current. This category includes metal-oxide-semiconductor FETs (MOSFETs), which are pivotal in digital circuits. MOSFETs offer high speeds and low power consumption, making them the preferred choice in modern CPU designs.
Understanding these types of transistors is vital as they impact how computers process data, perform calculations, and execute programs.
How Transistors Function
Transistors function primarily as switches or amplifiers in electronic circuits. Their operation relies on the movement of charge carriers, which allows them to control electrical current. In BJTs, the amplification process begins when a small input current at the base terminal allows a larger current to flow between the collector and emitter terminals.
FETs operate differently; they control the current via voltage. When voltage is applied at the gate terminal, it creates an electric field that either enhances or impedes the flow of current through the channel between the source and drain terminals.
This capability to control currents effectively enables transistors to function as the basic building blocks of logic gates used in computing. A single logic gate can perform simple tasks, while multiple gates combined can perform complex computations. This is how calculations are processed in systems, shaping the architecture of CPUs and overall computer systems.
In summary, transistors are foundational elements in digital computing, essential for information processing and energy efficiency. Their different types and functions embody the principles of electrical engineering and physics, illustrating how theoretical concepts translate into practical technology.
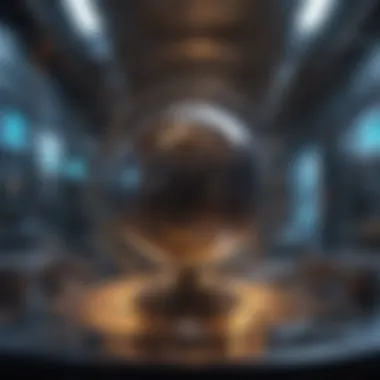
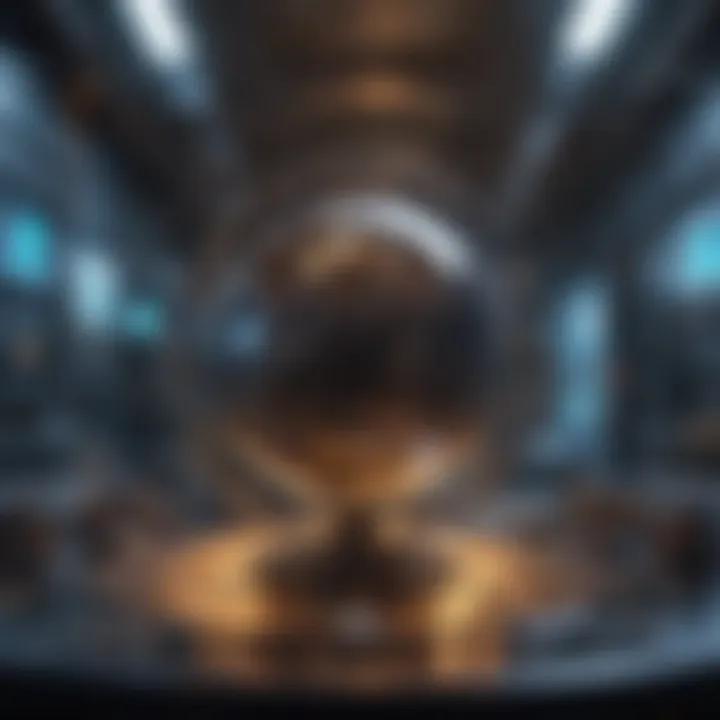
Information Encoding and Processing
In the realm of computing, information encoding and processing stands as a cornerstone. This section explores the mechanisms by which data is represented, managed, and manipulated within computer systems. Understanding these processes is crucial because they directly influence how efficiently computers can perform tasks.
Data encoding defines the way information is stored, communicated, and interpreted. Consider the difference between digital and analog systems, as each approach has its own merits and drawbacks. Digital systems generally provide greater precision and reliability, making them essential for todayβs computing technologies. In contrast, analog systems can capture the nuances of data but often at the cost of fidelity and noise.
Furthermore, the method of information processing determines how quickly a computer can execute operations. Efficient algorithms and architectures enable faster computation, which is vital in applications from simple calculations to complex simulations.
Digital vs. Analog Systems
Digital systems utilize discrete values to represent information. This characteristic allows for higher accuracy and easier data manipulation. Examples include binary encoding, where data is represented using two states: 0 and 1. As a result, digital signals are less susceptible to degradation from noise, making them ideal for robust data transmission and storage.
On the other hand, analog systems represent data in a continuous form. This can capture the smooth variations of real-world signals, such as sound and temperature. Nonetheless, analog systems face limitations in terms of precision, as minor fluctuations may alter the output. As a result, while analog technology has its place in specific applications, digital computing systems have predominated in modern technology.
The choice between digital and analog systems impacts not only technical performance but also user experience and reliability.
Binary Code Fundamentals
Binary code is the fundamental language of computers. Each piece of data, whether text, image, or sound, is converted into binaryβsequences of zeros and ones. The importance of binary code lies in its ability to simplify complex information into a manageable format for computers to process.
Binary operates on a base-2 numeral system. In this system, each digit represents an exponential power of 2. For example, the binary number 1011 breaks down as follows:
- 1 Γ 2^3 (8)
- 0 Γ 2^2 (0)
- 1 Γ 2^1 (2)
- 1 Γ 2^0 (1)
This equals 8 + 0 + 2 + 1 = 11 in decimal notation.
Through binary code, data structures become manipulable by computer processors. However, the limitations of binary systems come into play when representing certain types of data, like graphics and sound. Advanced encoding techniques have emerged to enhance the encoding of such data, yet binary remains the core foundation, shaping all digital communication and computation today.
Thermodynamics in Computing
Thermodynamics plays a crucial role in the field of computing, particularly in how computers generate and manage heat. As components become more densely packed and operate at higher speeds, the resulting heat can have profound effects on performance and reliability. Understanding the principles of thermodynamics allows engineers to create systems that not only operate efficiently but also remain within safe temperature ranges. This section delves into two essential aspects of thermodynamics related to computing: heat generation and management, as well as energy efficiency techniques.
Heat Generation and Management
Computers generate heat primarily due to the electrical resistance encountered in their components, especially in semiconductors and transistors. As electricity flows through these materials, energy is converted into heat. The rate of heat generation depends on several factors, including:
- The amount of current passing through the device.
- The resistance of the materials used in the components.
- The operational speed of the circuits.
Effective heat management is critical for maintaining the performance of computing systems. If heat is not dissipated properly, components can overheat, leading to throttling or even permanent damage. Common techniques for managing heat include:
- Heat sinks: These devices absorb and disperse heat away from critical components.
- Fans and cooling systems: Active cooling methods that circulate air to provide additional heat dissipation.
"Effective thermal management is not just an improvement; it is a necessity for modern computing systems."
- Thermal interface materials: Substances applied between surfaces to enhance heat transfer.
- Liquid cooling: A more advanced technique using liquids to absorb heat effectively.
Each of these methods aims to balance the need for high performance with the essential requirement of keeping temperatures in check. Engineers constantly innovate in thermal management strategies, as maintaining a lower temperature not only enhances reliability but also prolongs the lifespan of computer components.
Energy Efficiency Techniques
As the demand for computing power grows, so does the need for energy-efficient computing solutions. This is where thermodynamics intersects with energy considerations, leading to various techniques aimed at reducing energy consumption while maximizing performance. Notable techniques include:
- Dynamic voltage and frequency scaling (DVFS): This method adjusts the voltage and frequency according to the current workload, allowing for significant power savings during low activity periods.
- Energy-efficient architectures: Developing processor architectures focused on reducing power consumption while delivering high performance.
- Component integration: Reducing the number of components in a system can lead to decreased energy consumption due to less heat generated overall.
- Software optimizations: Writing code that minimizes process cycles can also have a substantial impact on energy use.
Incorporating these energy efficiency techniques is essential not just for the environment, but also for reducing operating costs in data centers and individual computing devices. With the increasing scarcity of energy resources, sustainable computing practices become more critical than ever.
Thermodynamics is a fundamental aspect of computing that encompasses both heat management and energy efficiency. By understanding the principles involved, stakeholders can make informed decisions to ensure that computer systems are both powerful and sustainable.
Quantum Computing: A Physics Revolution
Quantum computing represents a significant shift in the field of computing, influenced heavily by the principles of quantum mechanics. Traditional computing relies on bits as the smallest unit of information that can exist in one of two states: 0 or 1. Quantum computers, however, utilize quantum bits, or qubits, which can exist in multiple states simultaneously due to the phenomenon known as superposition. This capability is essential for performing complex calculations at speeds that were previously unimaginable.
One of the key benefits of quantum computing is its potential for solving problems that are currently intractable for classical computers. For instance, tasks such as factoring large numbers, simulating quantum systems, and complex optimization can be executed significantly faster. Moreover, the unique properties of quantum entanglement and superposition allow quantum computers to perform multiple calculations at once, enabling them to tackle various algorithms that classical systems would struggle with.
However, this revolutionary technology comes with challenges. The principles of quantum mechanics are inherently probabilistic, meaning that outcomes are not always guaranteed. This introduces complexities in programming and algorithm design. Additionally, qubits are highly susceptible to decoherence, leading to errors in computation. Hence, developing error correction pathways and stable qubit systems remains an active area of research.
In summary, quantum computing is a frontier of modern physics and computer science. Its implications reach far beyond theoretical experiments, posing a real opportunity for breakthroughs in numerous fields, including cryptography, materials science, and artificial intelligence.
Basics of Quantum Mechanics
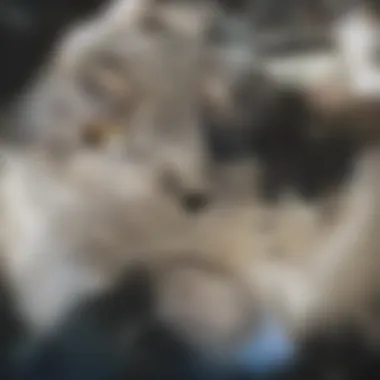
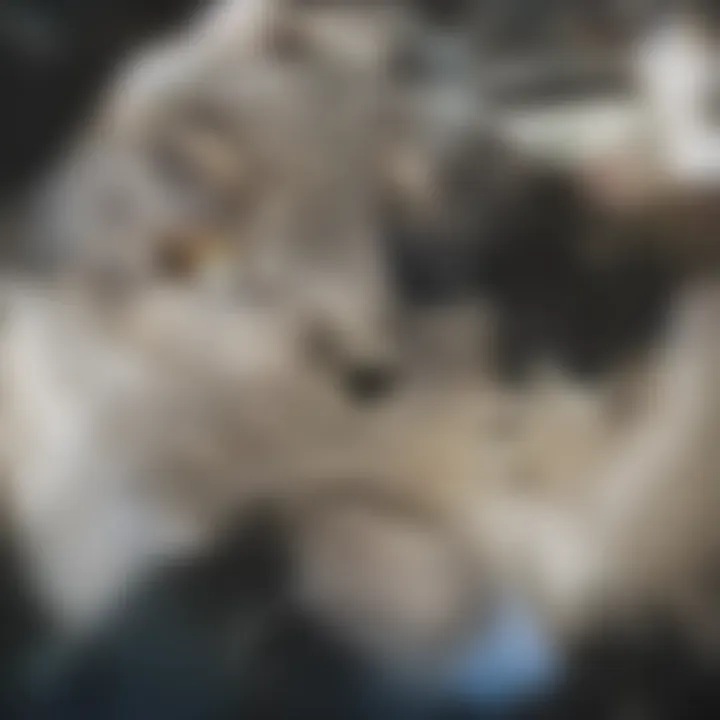
The foundation of quantum computing lies in the principles of quantum mechanics, which governs the behavior of particles at the atomic and subatomic levels. Unlike classical physics, which is deterministic, quantum mechanics introduces a probabilistic framework. Key concepts include:
- Superposition: The ability of a quantum system, like a qubit, to be in a combination of states rather than in a single state.
- Entanglement: A phenomenon where two or more qubits become interconnected, allowing the state of one to instantly influence the state of another, regardless of the distance separating them.
- Quantum interference: The process by which probabilities of different outcomes can combine to amplify desired results and cancel out unwanted outcomes.
Understanding these principles is crucial for grasping the significance of quantum computing. They dictate how qubits operate and interact, distinguishing quantum systems from classical ones.
Qubits and Their Advantages
Qubits serve as the fundamental building blocks of quantum computers. Their operation hinges on the unique properties of quantum mechanics, which confer significant advantages over classical bits:
- Parallelism: Qubits can represent multiple combinations of 0s and 1s at once, allowing quantum computers to perform many calculations simultaneously.
- Speed: For specific problems such as optimization or quantum simulations, quantum algorithms can dramatically reduce the time required for computation compared to classical algorithms.
- Efficiency: Certain problems can be solved in polynomial time as opposed to exponential time, especially in fields like cryptography and database searching.
- Complex Problem Solving: Quantum computers can simulate physical systems with high complex interactions, providing insights that classical computers cannot.
The Future of Computer Physics
The future of computer physics holds profound implications for technology and society at large. As we advance, the interplay between physics and computing will drive the next generation of technological innovation. This section delves into emerging technologies and the challenges that accompany them, illuminating the path forward in this ever-evolving field.
Emerging Technologies
Emerging technologies are at the forefront of computer physics. They promise enhancements across various aspects of computing, and their development can redefine how computers operate fundamentally. Key emerging technologies include:
- Quantum Computing: This area explores quantum bits or qubits that operate under the principles of quantum mechanics. Quantum computers can perform complex calculations much faster than classical computers. The implications for fields such as cryptography, materials science, and optimization are immense.
- Neuromorphic Computing: This technology mimics the structure and functioning of the human brain. It aims to enhance processing efficiency while reducing power consumption. Neuromorphic systems can bring significant advances in artificial intelligence, machine learning, and robotics.
- Photonic Computing: Utilizes light instead of electrical signals for processing and transferring information. Photonic circuits can yield faster data transmission with significantly lower energy consumption, addressing the growing demands for speed and efficiency in computing.
- Flexible Electronics: The development of flexible materials allows for innovative hardware designs. This technology can lead to the creation of portable, lightweight devices that can be integrated into everyday objects, enhancing the Internet of Things (IoT).
Adopting these technologies in computing can lead to not just faster but also smarter systems. However, along with potential benefits, emerging technologies may bring unforeseen implications that need careful consideration.
Challenges and Opportunities
With the prospect of advanced technologies comes a host of challenges and opportunities. Some critical challenges include:
- Technical Limitations: Many emerging technologies are at exploratory stages and face significant hurdles in materials science and engineering. For instance, scaling quantum computing to practical applications presents complex issues related to qubit stability and error correction.
- Integration and Standardization: New technologies must be effectively integrated with existing systems. The lack of established standards can lead to compatibility issues, complicating the deployment of these innovations.
- Ethical Considerations: As emerging technologies produce powerful capabilities, ethical dilemmas surface. For instance, quantum computing could undermine current cryptographic systems, raising concerns about data privacy and security.
Opportunities are vast, including:
- Economic Growth: Investments in new technologies can stimulate economic growth, leading to job creation in high-tech sectors.
- Scientific Advancement: Breakthroughs in computing can further scientific research in various fields, such as healthcare, climate science, and physics.
- Sustainability: Enhanced energy efficiency from new computing methods may contribute to sustainability efforts, addressing global challenges related to energy consumption and climate change.
"The future of computer physics is not just about faster computers, but smarter solutions that enhance our everyday lives."
Understanding the future of computer physics requires navigating both the exciting advancements and the potential pitfalls. This dual perspective will be essential for students, researchers, educators, and professionals in guiding the next phases of computing development.
Finale
The conclusion serves as a pivotal section that synthesizes the various insights presented throughout this article. It encapsulates the essence of the physics that underpin computing technology, making connections between fundamental principles and their practical implications in modern society. Recognizing the significance of topics such as semiconductors, electromagnetism, and quantum computing not only enhances understanding but also highlights the ongoing evolution of computer technology.
Recap of Key Concepts
Reflecting on the key concepts explored in this article, we have seen how each element plays an indispensable role in the functionality of modern computers:
- Electromagnetic Waves: Fundamental for data transmission across networks. They facilitate communication in various technologies, such as Wi-Fi and cellular networks.
- Semiconductors: The backbone of computer hardware, enabling control over electrical properties to create efficient circuits and transistors.
- Transistors: Building blocks essential for computation, allowing for the creation of logic gates that form the foundation of binary calculations.
- Thermodynamics: Critical in managing heat generation and ensuring energy efficiency within computer systems.
- Quantum Computing: Represents a transformative approach, harnessing quantum mechanics to transcend conventional limitations of processing power.
The integration of these components reveals a complex tapestry of interactions that define how computers operate today. Each concept, while unique, contributes to a broader understanding of computing as a scientific and technological pursuit.
The Interdisciplinary Nature of Computing
Computing stands at the intersection of various scientific disciplines. Physics, material science, and information theory converge to create the technology that shapes our world. This interdisciplinary approach enriches our comprehension of computers and fosters innovation.
- Physics provides foundational knowledge about energy, matter, and their interactions, essential for understanding electronic devices.
- Computer Science utilizes these physical principles to create algorithms, software, and systems that optimize data processing.
- Engineering applies this knowledge in the design and manufacture of computer components which are efficient and scalable.
The collaboration among these fields encourages advancements in technology. Understanding physics enhances the ability to address new challenges presented by emerging technologies, ensuring that computer systems continue to evolve and improve. By embracing an interdisciplinary mindset, we can anticipate the future of computing and the scientific innovations that will drive it.
Citing Key Literature
Citing key literature is essential for establishing a basis for the arguments conveyed in this article. Important studies and papers exert influences on both academic and practical aspects of computer physics. From foundational texts in electromagnetism to advanced research in quantum computing, literature serves as a gateway to understanding underlying principles and methodologies.
Some notable items might include:
- The Principles of Electric Circuits by Thomas L. Floyd, which discusses circuit theory and its applications.
- Introduction to Quantum Mechanics by David J. Griffiths, presenting foundational concepts in quantum theory relevant to computing.
- Articles in peer-reviewed journals such as IEEE Transactions on Computers, which reports on new advancements.
Accessing these resources ensures readers can connect theoretical concepts to practical applications. Understanding the history of research also provides context for current technologies and future advancements.
Further Reading Resources
For those interested in deepening their understanding of the physics of computers, several resources can serve as valuable tools for exploration:
- Computer Organization and Design by David A. Patterson and John L. Hennessy: A comprehensive text that covers hardware and architecture.
- Online repositories like Wikipedia and Britannica, which furnish summaries and detailed entries on numerous topics related to computing physics.
- Subreddit communities such as r/compsci where discussions about computer science theories are commonplace, offering insights and peer opinions.
Engaging with these resources cultivates a more rounded perspective on the materials and techniques that compose modern computing systems. This can spark new ideas, leading to a broader understanding of how the principles of physics are applied in creating the technology that shapes our world.