An Examination of Nuclear Reactions: Fundamentals and Implications
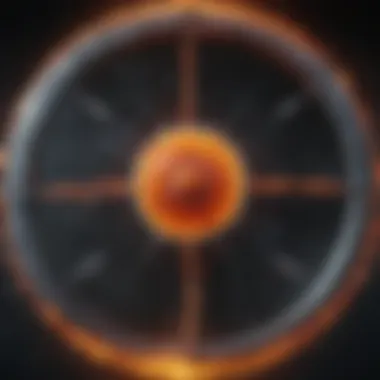
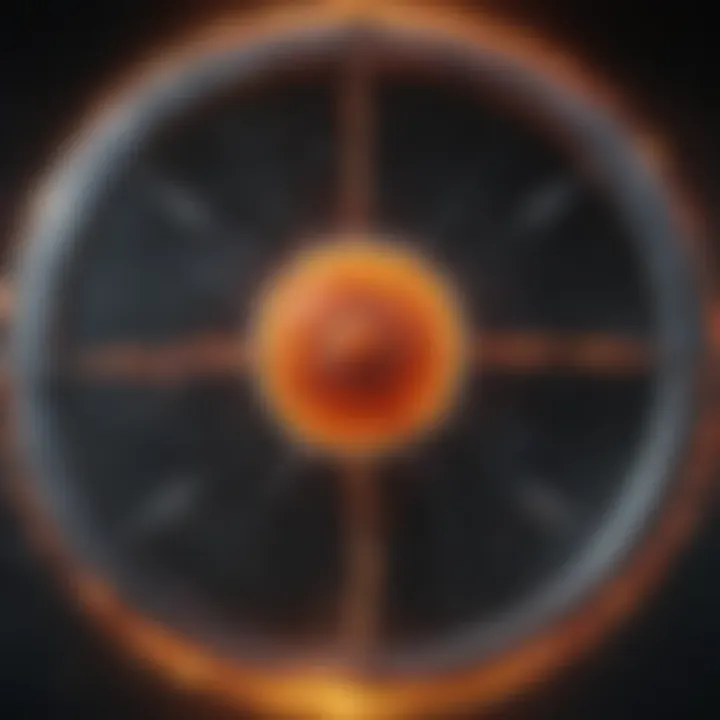
Intro
Nuclear reactions encompass a diverse range of processes that occur at the atomic level. They differ fundamentally from chemical reactions, which involve the rearrangement of electrons in atoms. In contrast, nuclear reactions involve changes in the nucleus of an atom itself. Understanding these reactions is essential for various applications, including energy generation and medical treatments. The profound impact of these reactions on society, policy, and the environment cannot be overstated.
This article aims to guide readers through the intricacies of nuclear reactions, detailing their fundamental principles and the implications of their applications. It will illuminate recent findings in the field, simplify complex concepts, and provide a structured overview of this crucial topic. As we navigate this discourse, it becomes clear how vital nuclear reactions are in shaping modern science and technology.
Key Research Findings
Overview of Recent Discoveries
In recent years, research in nuclear reactions has opened new avenues of understanding. One significant discovery is the identification of specific isotopes that can enhance energy output in reactors. For instance, studies reveal that isotopes like Uranium-235 and Plutonium-239 are central to fission reactions used in nuclear power plants. Furthermore, advancements are being made in fusion research, notably with projects such as ITER, which aims to demonstrate the feasibility of fusion as a sustainable energy source.
"Nuclear fusion has the potential to revolutionize how we view energy productionβproviding a safe, clean alternative to fossil fuels."
Significance of Findings in the Field
The findings are not merely academic; they have far-reaching implications for energy policy and safety protocols. With an increasing global focus on sustainability, understanding which nuclear reactions can provide safe energy solutions is crucial. Moreover, insights into medical applications of nuclear reactions, such as radiation therapy for cancer treatment, underscore their importance in healthcare. These discoveries reaffirm the necessity of ongoing research and development in the nuclear domain.
Breakdown of Complex Concepts
Simplification of Advanced Theories
Many find the theories behind nuclear reactions complex. Simplifying these can help readers grasp core principles. At its core, a nuclear reaction can be broken down into three primary processes: fission, fusion, and radioactive decay.
- Fission occurs when a heavy nucleus splits into smaller nuclei, releasing a large amount of energy.
- Fusion is the process where light nuclei combine to form a heavier nucleus, a reaction that powers the sun.
- Radioactive decay involves the transformation of an unstable nucleus into a more stable one, emitting radiation in the process.
Visual Aids and Infographics
Incorporating visual aids can significantly enhance understanding. Infographics illustrating the differences between fission and fusion help clarify these processes. For example, a diagram can show how Uranium-235 undergoes fission in a reactor, while another can depict the fusion process occurring in stars.
By employing simple diagrams and charts, complex interactions can be elucidated, aiding the reader in visualizing the intricate dynamics at play in nuclear reactions.
Understanding nuclear reactions is pivotal for a future where energy demands grow. The findings and concepts outlined in this article reveal how these reactions form the bedrock of advancements in energy production and medical applications.
Prolusion to Nuclear Reactions
Nuclear reactions are fundamental processes that govern the behaviors of atomic nuclei. Understanding these reactions is crucial for many fields, including energy generation, medicine, and even astrophysics. This article aims to lay the groundwork for comprehending nuclear reactions by exploring what they are, their significance in various applications, and their implications for society.
The consideration of nuclear reactions is vital due to their large-scale impact. From nuclear power plants that contribute to energy resources, to medical technologies utilizing radioactive materials, the ramifications of these reactions are both broad and profound. They differ from chemical reactions, as they involve changes in the nucleus of an atom rather than interactions between electrons. This fundamental distinction is important to grasp.
Furthermore, advancements in nuclear technology present both opportunities and challenges. As society grapples with energy demands and medical needs, the role of nuclear reactions becomes increasingly pivotal. This article will discuss these aspects, starting with a basic definition.
Defining Nuclear Reactions
Nuclear reactions involve a change in the composition of an atomic nucleus. These reactions can involve the fission of heavy elements, fusion of light elements, or other processes such as radioactive decay. Unlike chemical reactions, which largely depend on electron interactions, nuclear reactions modify the nucleus itself.
A typical nuclear reaction might look like this:
- Fission: A heavy nucleus such as Uranium-235 absorbs a neutron, becomes unstable, and splits into two lighter nuclei, releasing energy.
- Fusion: Light nuclei, for instance, Hydrogen isotopes, combine under extreme pressure and temperature to form Helium, which also releases energy.
- Radioactive decay: An unstable nucleus loses energy by emitting radiation, transforming into a different element or isotope.
These processes have distinct characteristics and applications that will be explored further in the article.
Historical Context
The concept of nuclear reactions emerged in the early 20th century, coinciding with the discovery of the nucleus itself. Pioneers like Ernest Rutherford were instrumental in elucidating the structure of the atom. The first artificial nuclear reaction was achieved by Rutherford in 1917, when he managed to convert nitrogen into oxygen by bombarding it with alpha particles.
The development of nuclear reactions took a significant leap with the discovery of nuclear fission in 1938 by Lise Meitner and Otto Hahn. This discovery paved the way for the construction of atomic bombs and the development of nuclear power plants. The subsequent use of nuclear reactions during World War II underscored the potential and dangers associated with nuclear technology.
Today, the exploration of nuclear reactions continues, with research focused on harnessing fusion as a clean energy source and improving medical isotope production. Understanding the historical context allows us to appreciate the evolution of nuclear science and its implications for future advancements.
The Fundamental Science of Nuclear Reactions
Nuclear reactions represent a convergence of physical laws and phenomena that reveal the intricate behaviors of atomic nuclei. Understanding these principles is crucial, as they underpin various fields, from energy generation to medicine. This section will delve into the basic concepts of nuclear physics and explore the relationship between mass and energy, crucial components for comprehending how nuclear reactions occur and their wide-reaching implications.
Basic Concepts of Nuclear Physics
Nuclear physics studies the constituents and interactions of atomic nuclei. Central to this field is the understanding of protons and neutrons, collectively known as nucleons. The forces that hold these particles together are termed nuclear forces. Awareness of these basic concepts allows one to comprehend the behavior and stability of different isotopes. Isotopes, which are versions of elements with varying neutron numbers, play pivotal roles in nuclear reactions.
Additionally, the concepts of binding energy and nuclear stability are crucial. Binding energy refers to the energy required to break a nucleus into its individual protons and neutrons. A higher binding energy usually indicates a more stable nucleus.
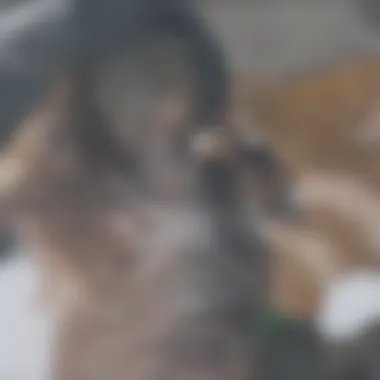
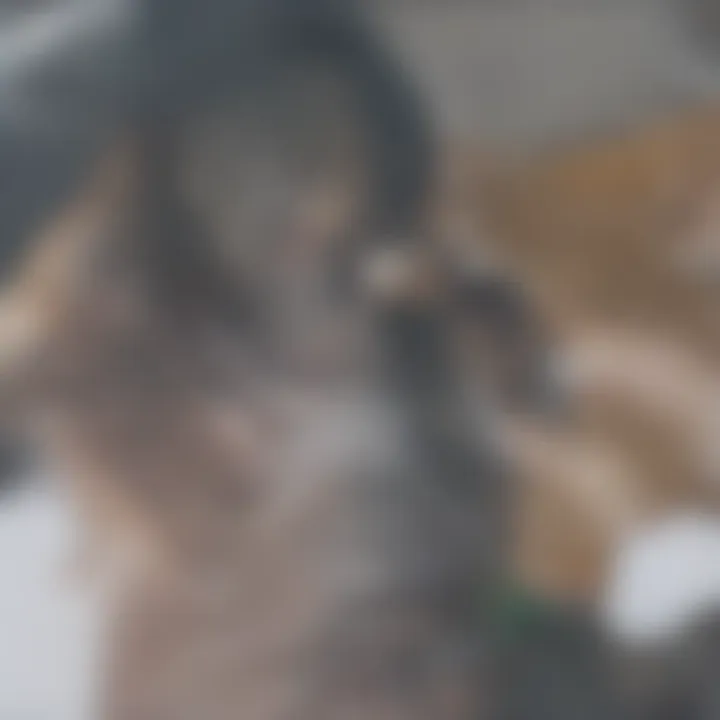
Interplay Between Mass and Energy
Einstein's Mass-Energy Equivalence
Einstein's mass-energy equivalence, encapsulated in the equation E=mcΒ², serves as a cornerstone in nuclear physics. This principle asserts that mass can be converted into energy and vice versa, effectively linking the two fundamental measures in physics. Its contribution to the understanding of nuclear reactions cannot be overstated. It explains why nuclear reactions release vast amounts of energy compared to chemical reactions, where energy changes are relatively minor.
The key characteristic of this concept is its simplicity and elegance. It aids in predicting the energy released during nuclear fission or fusion, making it highly beneficial for this article. One unique feature of mass-energy equivalence is its applicability across various scientific fields, helping to unify theories of energy. The primary disadvantage, however, resides in the complexity of achieving the conditions needed for significant mass-energy conversion, such as in stars or advanced nuclear reactors.
Conservation Laws
The conservation laws, particularly concerning mass-energy and momentum, form a fundamental aspect of nuclear reactions. These laws dictate that certain quantities remain constant before and after reactions. In nuclear processes, despite the conversion happening, the total energy remains constant, honoring the conservation of mass-energy. This relationship is not only a key feature but also a foundational pillar for predicting reaction outcomes and system behaviors.
The benefits of these principles are evident, enabling scientists to model reactions accurately and design reactors with specific outputs. One unique aspect of conservation laws is their universality; they apply to all physical processes, making them immensely significant in any discussion about nuclear reactions. A potential disadvantage is that real-world scenarios often introduce variables that can complicate strict adherence to these laws, especially in chaotic environments or at the quantum level.
Understanding the fundamental science of nuclear reactions requires a deep appreciation of the laws governing matter and energy. The implications of these reactions reach far beyond theoretical knowledge, influencing technology and our daily lives in profound ways.
In summary, the fundamental science encapsulated in nuclear reactions is essential for grasping the nature and impact of nuclear phenomena. By mastering these basic principles, one can appreciate how they shape various applications, from generating power in nuclear plants to innovative medical treatments.
Types of Nuclear Reactions
Nuclear reactions are crucial to various scientific and practical fields. They form the backbone of many processes, from energy generation to medical advancements. Understanding the types of nuclear reactions can help elucidate their unique properties and applications. The three main types of nuclear reactions are fission, fusion, and radioactive decay. Each type exhibits distinct characteristics and plays a critical role in our technological landscape.
Fission
Fission refers to the process where a heavy nucleus splits into two lighter nuclei along with a release of a significant amount of energy. This reaction is most commonly associated with elements like uranium-235 and plutonium-239. The energy yield from fission is substantial; it can release millions of electron volts per reaction.
One of the pivotal applications of fission is in nuclear power plants, where it serves as the primary reaction to generate electricity. In these power facilities, the heat produced during fission is used to create steam that turns turbines, generating electrical energy. Besides energy production, fission plays a role in nuclear weapons, where the energy released can lead to catastrophic results.
Here are some key aspects of fission:
- Chain Reaction: Fission has a unique characteristic known as a chain reaction. This occurs when one fission event causes additional fission events. This principle is fundamental in designing nuclear reactors.
- Control Mechanisms: Power generation through fission necessitates careful control. Control rods made of materials like boron or cadmium are used to absorb excess neutrons, ensuring the reaction proceeds at a manageable rate.
- Radioactive Waste: A significant consideration in fission is the generation of radioactive waste. These byproducts can remain hazardous for thousands of years, requiring effective management strategies.
"The potential of fission in energy generation is vast, but the management of its implications is equally significant."
Fusion
Fusion is the reverse of fission. This process involves two light nuclei combining to form a heavier nucleus, accompanied by an enormous release of energy. Fusion is the reaction that powers stars, including our sun, where hydrogen nuclei fuse to form helium.
The implications of fusion are profound. If harnessed effectively, fusion could provide a source of energy that is cleaner and virtually limitless. Unlike fission, the primary fuel isotopes for fusion, such as deuterium and tritium, are abundant. New advancements in fusion research aim to develop reactors that can achieve sustained fusion reactions.
Key points regarding fusion include:
- Energy Yield: The energy released in fusion reactions is considerably larger than that of fission reactions, making it an attractive option for future energy solutions.
- Safety: Fusion offers safety advantages over fission. There is no risk of a runaway reaction, as the conditions for fusion are difficult to maintain. If containment is lost, the reaction simply ceases.
- Environmental Impact: Fusion generates minimal radioactive waste. Most byproducts return to non-radioactive forms, addressing some of the environmental concerns associated with fission.
Radioactive Decay
Radioactive decay refers to the process through which unstable atomic nuclei lose energy by emitting radiation. This decay can occur in several different modes, including alpha decay, beta decay, and gamma decay. Each type has unique properties and implications.
Radioactive decay is significant for several reasons:
- Natural Process: It is a natural process occurring over a time scale characterized by half-lives, which can vary drastically among different isotopes.
- Applications in Medicine: One of the most practical applications of radioactive decay is in the field of medical imaging. Radioisotopes are used in diagnostic techniques such as PET scans and radiation therapy for cancer treatment. For instance, technetium-99m is widely used in diagnostics due to its favorable properties.
- Dating Techniques: Radioactive decay also underpins methods such as carbon dating, which enables scientists to determine the age of organic materials.
In summary, the types of nuclear reactions encompass a wide spectrum of phenomena, each with its application and implications. They are essential for advancing human knowledge and technological capabilities in several domains.
The Role of Isotopes in Nuclear Reactions
Isotopes play a critical role in nuclear reactions, influencing both the processes and the outcomes of these complex interactions. Their importance extends into various fields, making it essential to understand their characteristics and applications. In essence, an isotope is a variant of a specific chemical element that has the same number of protons but differs in the number of neutrons. This variation leads to different atomic masses and properties, which can significantly impact how that isotope interacts in nuclear reactions.
One key benefit of isotopes in nuclear reactions is their ability to serve specific functions based on their unique nuclear properties. For instance, some isotopes may be more stable or have longer half-lives than others, making them favorable for particular applications. In contrast, other isotopes might exhibit more rapid decay, which can be advantageous in different scenarios, such as medical treatments or energy production.
Understanding isotopes also involves recognizing their various applications in both energy generation and medical fields. The manipulation of isotopes is fundamental in designing reactors and medical procedures aimed at improving outcomes. Each isotope can bring distinct advantages or disadvantages, reflecting the necessity of careful selection based on the intended use.
Understanding Isotopes
To comprehend the role of isotopes, one must first grasp what defines them. Isotopes differ from each other by their neutron count, leading to variations in mass and stability. For example, Carbon-12 and Carbon-14 are both isotopes of carbon. While Carbon-12 is stable and commonly found in nature, Carbon-14 is radioactive and decays over time. This decay is utilized in radiocarbon dating, underscoring how isotopes can fundamentally alter scientific practices.
Moreover, the concept of isotopes extends beyond simple definitions to include their behavior in reactions. Depending on their nuclear structure and stability, isotopes may participate in nuclear reactions with differing efficiencies and products.
Applications of Isotopes in Reactions
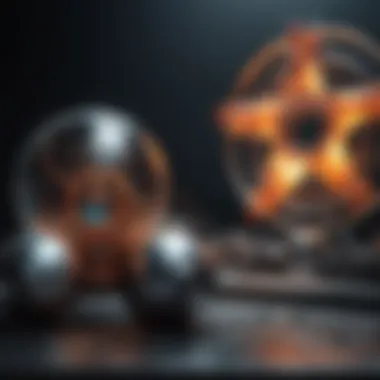
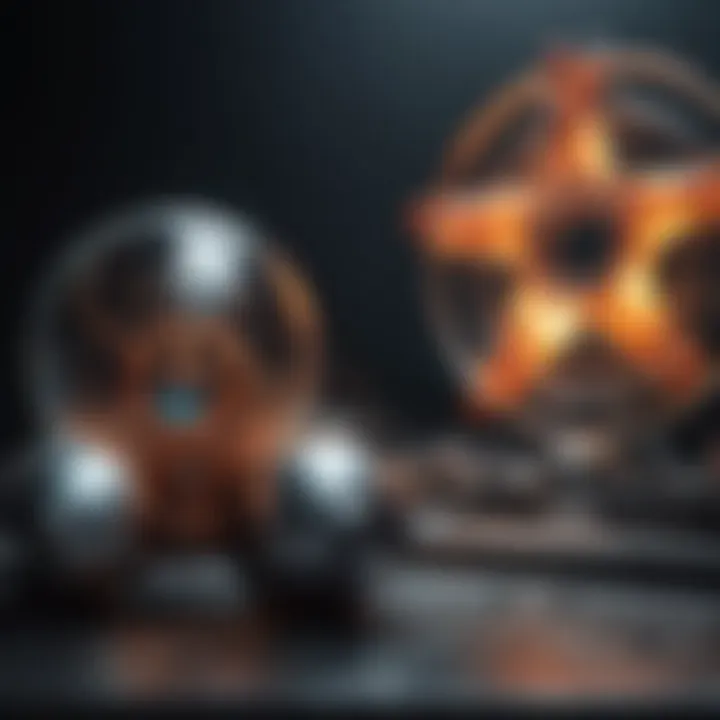
Medical Isotopes
Medical isotopes are pivotal in diagnostics and treatment strategies in healthcare. For instance, Technetium-99m is widely used in medical imaging. It provides vital information about the functionalities of organs and tissues, aiding in the detection of diseases. The key characteristic of medical isotopes is their ability to decay in a manner that emits radiation detectable by imaging devices. Their short half-lives minimize the radiation exposure to patients, making them a popular choice.
"The use of medical isotopes has transformed surgery and patient care, providing precise imaging that was not possible before."
However, one unique feature of medical isotopes is their generation process, often requiring complex facilities and significant resources. This can be a disadvantage, especially in areas with limited access to advanced technologies.
Nuclear Fuel
Nuclear fuel isotopes are fundamental to energy production. Uranium-235 and Plutonium-239 are primary examples, used extensively in nuclear reactors. Their capability to undergo fission releases large quantities of energy, which is harnessed for electricity generation. The key characteristic of nuclear fuel isotopes is their ability to sustain nuclear chain reactions, making them essential for reliable energy supply.
The unique feature of nuclear fuel isotopes lies in their energy density; they provide substantially more energy per atom than traditional fuel sources. However, this comes with challenges, such as ensuring safety and managing nuclear waste. The long-lived nature of some fission products complicates waste disposal, necessitating robust management strategies.
In summary, isotopes significantly impact nuclear reactions across various domains. Their unique properties and applications highlight the importance of understanding their role in both scientific research and practical implementations.
Mathematics of Nuclear Reactions
Understanding the mathematics behind nuclear reactions is essential. The calculations can clarify how energy transformations occur during fission and fusion processes. Also, it lays foundation for predicting the behavior of radioactive materials. Grasping these concepts allows scientists and engineers to design safer nuclear systems and innovate in medical applications.
Energy Calculations in Fission and Fusion
Energy calculations in nuclear reactions, particularly fission and fusion, involve several crucial steps.
- Nuclear Binding Energy: This energy measures the stability of a nucleus. It is the energy required to disassemble a nucleus into its individual protons and neutrons.
- Einsteinβs Mass-Energy Equivalence: This principle states that mass can be converted into energy and vice versa, expressed in the equation E=mcΒ². In nuclear reactions, a small amount of mass loss can result in large energy release during fission or fusion.
- Q-Value: The Q-value of a nuclear reaction is the amount of energy released or absorbed. It is calculated based on the mass difference between reactants and products. This calculation is beneficial when determining if a particular reaction is favorable.
The importance of these energy calculations is clear. They can predict the efficiency and outcomes of nuclear reactions, guiding both energy generation techniques and safety measures in handling radioactive substances.
Half-Life and Radioactive Decay Calculations
The concept of half-life is fundamental to understanding radioactive decay. The half-life of a radioactive isotope is the time required for half of the nuclei in a sample to undergo decay. This measure is critical in fields such as nuclear medicine and waste management.
- Decay Constant: This is a value that represents the probability of decay of a nucleus per unit of time. It is related to half-life via the formula:[ T_1/2 = \frac0.693\lambda ]where ( T_1/2 ) is the half-life and ( \lambda ) is the decay constant.
- Exponential Decay: The quantity of a radioactive substance decreases exponentially over time. The relationship can be expressed in the equation:[ N(t) = N_0 e^-\lambda t ]where ( N(t) ) is the remaining quantity at time ( t ), ( N_0 ) is the initial quantity, and ( e ) is the base of the natural logarithm.
These calculations are not only theoretical. They have practical implications in determining safety measures regarding the disposal of nuclear waste and in calculating doses in radiation therapies. Overall, mathematics in nuclear reactions provides necessary insight to innovate and apply nuclear technology responsibly.
Practical Applications of Nuclear Reactions
The practical applications of nuclear reactions span various fields, each contributing significantly to advances in technology and medicine. Understanding these applications helps to elucidate the significant role that nuclear physics plays in contemporary society. From power generation to medical treatments, nuclear reactions provide solutions for many challenges faced in diverse sectors.
Nuclear Power Generation
Nuclear power generation harnesses the energy released from nuclear fission to produce electricity. This method provides a substantial portion of energy resources worldwide. The main advantage of nuclear power is its ability to generate large amounts of energy from a small amount of fuel, typically uranium-235 or plutonium-239. Unlike fossil fuels, nuclear energy emits significantly lower levels of greenhouse gases, making it a more environmentally friendly option for large-scale energy production.
However, nuclear power comes with its considerations. The risk of nuclear accidents, such as those that occurred at Chernobyl and Fukushima, raises valid safety concerns. Furthermore, the management of nuclear waste remains an unresolved issue, prompting discussions around disposal methods and long-term storage solutions.
Medical Applications of Nuclear Reactions
The medical field also benefits immensely from nuclear reactions, particularly through two main applications: chemotherapy and diagnostics. These procedures utilize radioactive isotopes to either treat diseases or gather important health information.
Chemotherapy
Chemotherapy often employs radioactive isotopes to target and destroy cancer cells. The key characteristic of chemotherapy is its ability to penetrate and attack cells that divide rapidly, which is a hallmark of cancerous tissues. This aspect makes chemotherapy a popular choice in cancer treatment, as it can lead to significant reductions in tumor size and the stopping of cancer progression.
A unique feature of chemotherapy is its combination with other methods, like surgery and radiation therapy, allowing for a more comprehensive treatment plan. However, the approach has its disadvantages. One notable drawback is the damage it can cause to healthy cells, leading to side effects like nausea, fatigue, and compromised immunity.
Diagnostics
In diagnostics, nuclear reactions facilitate imaging techniques that provide essential information regarding the functioning of internal organs. Using radiotracers, medical professionals can obtain valuable insights into conditions such as heart disease, thyroid issues, and cancers. The beneficial aspect of using nuclear diagnostics lies in its non-invasive nature, allowing doctors to gather crucial information without the need for exploratory surgery.
The unique feature of this approach is its ability to visualize processes in real time, which is essential for timely and accurate diagnosis. Nonetheless, it is important to consider the exposure to radiation, which can pose risks to patients, though these are generally minimized by limiting the duration and dosage used in imaging.
In summary, nuclear reactions play a transformative role in both energy production and medical advancements. The ramifications of these applications influence not only technological progress but also societal health outcomes.
Nuclear Reactions and Society
Nuclear reactions play a critical role in shaping societal structures and advancing technological progress. The implications of these reactions extend far beyond the confines of laboratories, affecting energy policies, medical practices, and public opinion. Understanding how society interacts with nuclear reactions is essential in addressing contemporary challenges such as climate change, health care, and national security.
Ethical Considerations
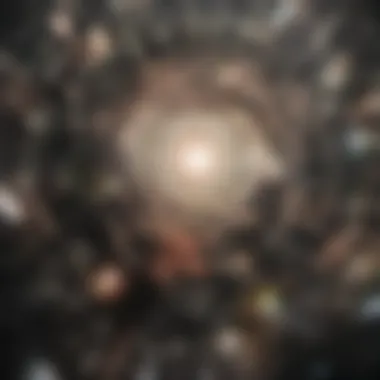
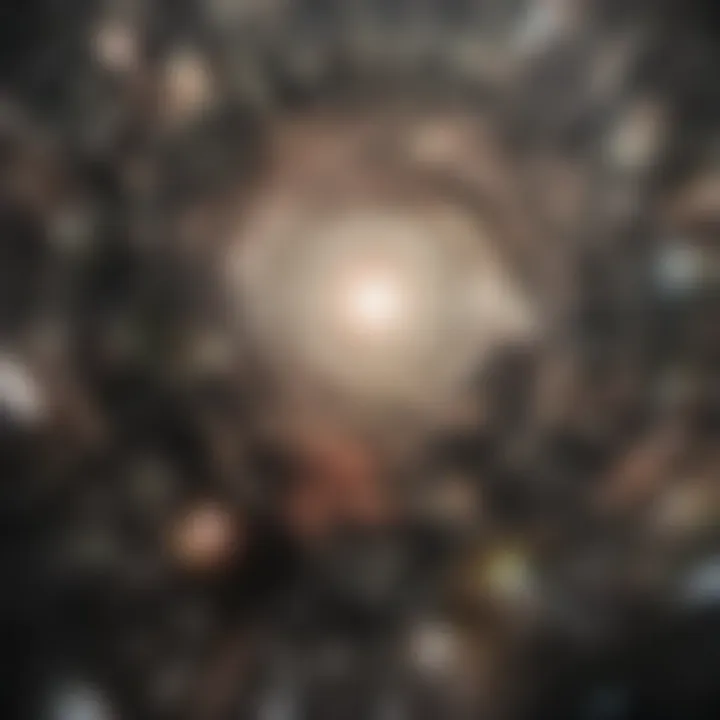
When discussing nuclear reactions, ethical considerations cannot be overlooked. The potential benefits of nuclear technology must be balanced with the risks it poses.
- Benefit-Risk Analysis: Each nuclear process, such as fission or fusion, brings forth significant advantages. These include energy generation with low greenhouse gas emissions and medical applications through isotopes. However, the associated risks, particularly those linked to nuclear waste and accidents, create ethical dilemmas. The decision-making process must incorporate public safety alongside technological advancement.
- Informed Consent in Medicine: In medical applications, the use of nuclear medicine, including chemotherapy and diagnostic imaging, demands informed patient consent. Patients need to understand the risks and benefits before undergoing procedures that involve radioactive materials. Educating the public about these processes is critical for ethical transparency.
- Environmental Impact: Nuclear energy generates power with minimal air pollution. Yet, the environmental consequences of nuclear waste disposal raise ethical questions. Long-term storage solutions require careful planning and transparency to ensure safety for future generations.
"The ethical framework should guide research and policy in Nuclear Science, balancing innovation with moral responsibility."
Public Perception and Policy
Public perception of nuclear reactions can significantly influence policy decisions. The widespread fear stemming from nuclear accidents, as witnessed in incidents like Chernobyl and Fukushima, shapes public attitude towards nuclear energy.
- Fear vs. Facts: Misinformation and sensationalized media coverage can lead to fear and resistance. It becomes imperative to promote a better understanding of nuclear science and its benefits. Clear communication and evidence-based information can help alleviate fears.
- Government Regulations: Policymaking around nuclear energy is often driven by public opinion. Hence, it is crucial that policymakers consider scientific perspectives when formulating regulations. Striking a balance between safety and progress in nuclear research requires thoughtful engagement with the public.
- Sustainable Energy Policies: As global awareness around climate change grows, the role of nuclear energy as a clean energy alternative becomes important. Promoting policies that embrace nuclear as a sustainable energy source can help mitigate carbon emissions.
In summary, understanding nuclear reactionsβ societal implications highlights the importance of ethical engagement and public discourse. The ongoing dialogue between scientists, policymakers, and the public is essential to build trust and advance nuclear technology responsibly.
Safety and Environmental Implications
Understanding the safety and environmental implications of nuclear reactions is essential in todayβs world. As nuclear technology advances, the potential benefits come with significant responsibilities. Issues such as nuclear waste management, risk assessment, and public safety become crucial components in the overall evaluation of nuclear processes. This subject prescribes a dual lens through which we examine how nuclear energy impacts society and the environment. Beneath the scientific complexities lies the need for thorough oversight and practical applications of safety protocols.
Nuclear Waste Management
Nuclear waste management refers to the processes involved in handling, storage, and disposal of radioactive materials generated from nuclear reactions. Waste can remain hazardous for thousands of years, necessitating meticulous strategies to mitigate risks.
- Types of Waste: Nuclear waste can be categorized into low-level waste, intermediate-level waste, and high-level waste, each requiring varied handling and storage solutions.
- Storage Solutions: Safe storage involves methods such as deep geological repositories, above-ground facilities, and spent fuel pools. The primary goal is to isolate waste from the environment effectively.
- Recycling Initiatives: Some countries have adopted recycling methods to recover usable materials from spent nuclear fuel, reducing overall waste. However, this comes with its own set of safety concerns and economic factors.
Management of nuclear waste not only focuses on immediate safety but also considers long-term environmental impact and sustainability. Governments and organizations must collaborate closely to establish effective regulations and communication about safety measures to ensure public confidence.
Risk Assessment in Nuclear Energy
Risk assessment in nuclear energy involves evaluating the potential hazards associated with nuclear power facilities and their operations. Effective risk assessment integrates data collection, analysis, and predictive modeling to find areas of vulnerability.
- Operational Risks: Risks during operation include equipment failure, human error, or natural disasters. Regular training and assessments are essential in minimizing such risks.
- Emergency Preparedness: Plans must be established for emergency response, as nuclear accidents, while rare, can lead to severe consequences for public safety and the environment. This includes drills, resource allocation, and public communication strategies.
- Regulatory Oversight: Agencies, such as the Nuclear Regulatory Commission (NRC) in the United States, are tasked with creating and enforcing safety standards. Compliance with these regulations is paramount to ensuring stability and public trust in nuclear energy initiatives.
In summary, nuclear reactions hold immense potential but come with inherent risks. The protection of communities and the environment is non-negotiable. Proper management of nuclear waste and stringent risk assessment protocols are essential to developing a safe nuclear future.
Future Prospects of Nuclear Research
Nuclear research is at a pivotal moment, with promising advances that could reshape existing paradigms. The future of nuclear energy and its applications is tied closely to innovation in both fusion technology and isotope research. This section discusses the importance of these advancements and the accompanying implications that must be considered.
Advancements in Fusion Technology
Fusion technology presents the prospect of an abundant and clean energy source. Unlike fission, which splits heavy atoms, fusion combines light nuclei, typically isotopes of hydrogen, to release vast amounts of energy. One significant advancement is the development of tokamak reactors. The ITER project in France is a prime example, aiming to demonstrate the feasibility of fusion as a large-scale and carbon-free source of energy.
The potential benefits of fusion energy include:
- Minimal Waste: Fusion produces less long-lived radioactive waste compared to fission.
- Abundant Fuel Supply: Isotopes like deuterium can be extracted from seawater.
- Safety: There is a significantly lower risk of catastrophic accidents because the fusion process does not involve a chain reaction.
Despite its potential, challenges remain. The technical difficulty of maintaining the extremely high temperatures required for fusion, around 15 million degrees Celsius, is significant. Moreover, achieving net energy gain has yet to be realized consistently.
Innovative Applications of Isotope Research
Isotope research continues to open new avenues across various fields, notably in medical and environmental sciences. The exploration of isotopes offers insights that transform theoretical practices into practical applications. For instance, in medicine, isotopes are used in diagnostics and treatments.
Some primary applications include:
- Medical Imaging: Isotopes like Technetium-99m are widely used in advanced imaging techniques to diagnose conditions.
- Targeted Radiation Therapy: Isotopes can be directed at cancer cells, minimizing damage to surrounding healthy tissue.
In environmental science, isotopes can track pollutants and study climate change impacts. Research into radioactive isotopes provides tools for understanding complex geological processes.
As isotope technology progresses, ethical questions arise regarding safety and regulatory frameworks. It is crucial to address these concerns to ensure that innovation benefits society without unintended consequences.
"The future of nuclear research lies in harnessing the potential of fusion and isotopes, balancing safety, sustainability, and ethical considerations."
Finale
In summation, the realm of nuclear reactions remains a cornerstone of modern scientific inquiry, impacting various domains from energy production to medical advancements. Understanding the key elements discussed in this article helps to illuminate the complexities and ramifications of these reactions. The implications of nuclear science are both profound and multifaceted, pointing towards significant benefits alongside critical considerations for safety and ethical considerations.
Summary of Key Points
- Nuclear Reactions Defined: It is essential to distinguish between nuclear and chemical reactions, focusing on the fundamental differences in mechanisms and outcomes.
- Types of Nuclear Reactions: We examined fission, fusion, and radioactive decay, revealing their unique properties and potential applications.
- Role of Isotopes: Understanding isotopes is crucial for harnessing their applications, particularly in medical fields and energy generation.
- Mathematical Underpinnings: The calculations surrounding energy yield in fission and fusion highlight the intricate relationship between mass and energy.
- Societal Implications: The societal discourse surrounding nuclear power and ethics remains paramount, influencing public perception and policy formation.
- Safety Concerns: Proper management of nuclear waste and risk assessment in nuclear energy play a significant role in public safety and environmental protection.
The Future of Nuclear Science
The future of nuclear science looks promising, with ongoing advancements poised to redefine our capabilities. Research in fusion technology holds the prospect of almost limitless energy, which could drastically change energy dynamics globally. Moreover, the innovative applications of isotopes are expected to expand, bringing new methodologies in medicinal treatments and diagnostic procedures.
The evolution of regulations and policies will also be vital. As society grapples with the environmental challenges of energy production, integrating nuclear options into mainstream solutions will demand careful oversight. Stakeholders must balance progress with safety to ensure that future developments serve the collective good without compromising ethical standards.
"The future of nuclear science hinges on responsible exploration and implementation that aligns technological advancements with humanity's best interests."