The Role of Magnetic Forces in Space Exploration
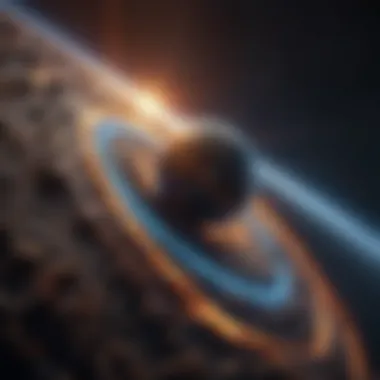
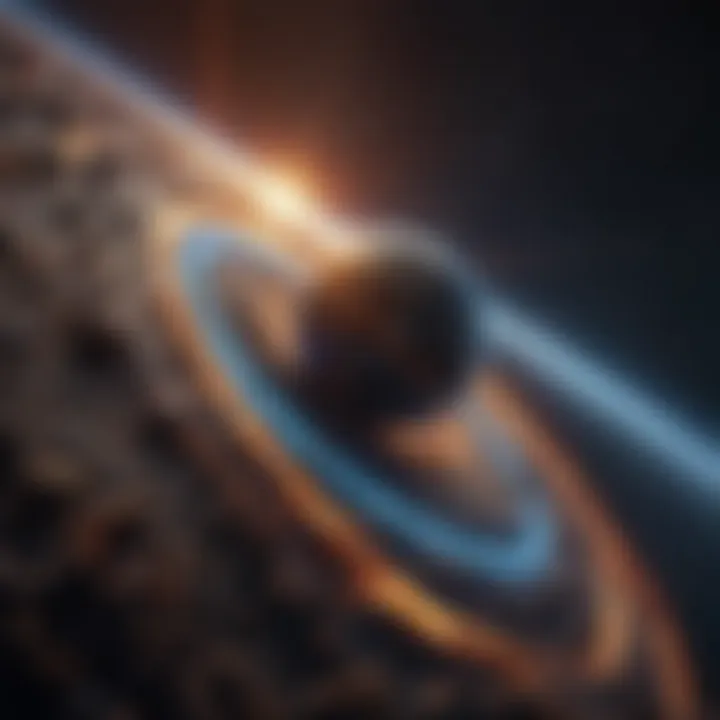
Intro
Magnets have always held a certain mystiqueโnot just on Earth but in the vastness of space as well. The intricate dance of magnetic fields influences a multitude of phenomena, ranging from the orbits of planets to the behavior of solar winds. In an environment where gravity's grip is lessened, the forces of magnetism wield a significant influence. This article seeks to delve into the unique role that these magnetic forces play beyond our planet, touching upon their origins, types, and the implications they hold for future exploration.
Understanding magnets in space is not merely an academic pursuit; it encompasses practical applications that impact spacecraft design, communication technologies, and even our safety as we venture further into the cosmos. The bridge between complex scientific knowledge and everyday technology reveals how vital these magnetic forces are in crafting the future of space exploration.
We'll explore key research findings, such as the discoveries surrounding planetary magnetic fields and the effects of solar activity. The journey will also unpack some advanced theories, making them accessible for all, ensuring that whether you're a student, researcher, or professional, you'll find value in the information presented here.
Understanding the Nature of Magnets
In the pursuit of unraveling the mysteries of the cosmos, one cannot overlook the profound influence of magnets. Understanding the nature of magnets serves as a cornerstone for grasping their role in space. Magnets are not just playground toys; theyโre fundamental players in the grand theater of astrophysics, influencing everything from planetary formation to the behavior of stars. By delving into the core principles of magnetism, we can better appreciate how they shape the universe.
This topic is significant because comprehending magnetism provides insight into various phenomena in spaceโmagnetic fields govern the interactions of celestial bodies. The characteristics of these fields dictate conditions in environments that can harbor life and those that are inhospitable. Without this understanding, much of astrophysics would remain enshrouded in mystery.
Moreover, the exploration of magnets extends beyond theoretical physics; it bears practical implications for the design of spacecraft and navigation systems critical for space exploration. To this end, itโs crucial to grasp the basic principles underpinning magnetism and to discern the various types of magnets and their properties.
Basic Principles of Magnetism
Magnetism itself springs from the interplay of electric charges. At the most fundamental level, when electrons circulate around atoms, their motion creates tiny magnetic fields. When many of these tiny magnets align, they can produce a larger, observable magnet. This underlying principle is what links electricity and magnetismโa relationship that bears profound consequences throughout physics.
Key points to note about magnetic fields include:
- Magnetic fields are vector fields: They possess both direction and magnitude, indicated by field lines that emerge from the north pole and return to the south pole of a magnet.
- Like poles repel and opposite poles attract: This simple rule helps in understanding various interactions not just among magnets but also within planetary and stellar magnetism.
- Flux density: It describes the strength of a magnetic field at any point in space, which is crucial for engineers and scientists as they plan missions to explore magnetized bodies.
Magnetism is more than an abstract concept; it's a phenomenon that permeates our understanding of the universe.
Types of Magnets and Their Properties
Magnets can be categorized into three main types: permanent magnets, temporary magnets, and electromagnets. Each type possesses unique characteristics, contributing to their function in diverse applications.
- Permanent Magnets: These magnets maintain a persistent magnetic field due to the alignment of atoms within their structure. Common materials include neodymium and ferrite. They find use in a wide array of applications, from fridge magnets to sophisticated scientific instruments.
- Temporary Magnets: These materials act like magnets when exposed to a magnetic field but lose their magnetism once it's removed. This feature is commonly observed with iron shavings placed near a magnet, revealing short-term magnetization.
- Electromagnets: These fascinating devices generate a magnetic field when an electric current passes through a coil of wire. Transformer designs and magnetic levitation trains often utilize this principle. Their field strength can be varied, providing versatile applications in technology.
By grasping these variations among magnets, one gains a richer understanding of their utility in space exploration and other scientific endeavors.
"Understanding the intricacies of magnetism opens doors to advancements in technology and ignites the quest for knowledge about the universe's magnetic forces."
Magnetic Fields: An Astrophysical Perspective
Magnetic fields are not just invisible forces that keep our compasses aligned; they play a crucial role in the cosmos. When discussing astrophysics, itโs essential to understand the nature and implications of these magnetic fields. They guide the motion of celestial bodies, impact stellar evolution, and deeply influence planets, including our own Earth. Understanding how magnetic fields operate in space enriches our comprehension of myriad cosmic phenomena, from the birth of stars to the behavior of galaxies.
The significance of exploring magnetic fields in this context is multi-faceted. Firstly, magnetic fields affect the dynamics of space weather, which can have profound effects on satellite operations and even power grids on Earth. Moreover, the study of these fields enhances our knowledge of planetary habitability and the conditions necessary for life beyond our planet. With advancing technologies, we can analyze these magnetic forces in greater detail, opening the door for future interstellar endeavors.
The Generation of Magnetic Fields in Space
Magnetic fields are generated through various processes, even in the vast emptiness of space. One primary method is the dynamo effect, which occurs within the interior of celestial bodies. In essence, a celestial body like a planet or a star generates a magnetic field by the movement of electrically conductive fluids, such as molten iron in the Earthโs outer core. This dynamic motion produces electric currents, which in turn create magnetic fields that extend far into space.
However, celestial bodies are not the only players in the magnetic field game. Galactic winds, cosmic rays and plasma interactions also contribute. For example, when charged particles collide with magnetic fields within stellar winds, they produce complex magnetic structures and turbulence, which can be observed across vast distances. The variability and dynamism of these fields shape everything from the orbits of planets to the propagation of cosmic rays, making their study vital for todayโs astrophysicists.
The Role of the Sunโs Magnetic Field
The Sun's magnetic field is particularly fascinating, not just for its own sake, but for how it influences our entire solar system. Solar magnetic activity, including phenomena like sunspots and solar flares, is a direct result of this field. These activities have significant ramifications for space weather and can affect satellite systems and even ground-based technologies.
During solar flares, for instance, charged particles are ejected at alarming speeds. If they interact with Earth's magnetic field, it can lead to geomagnetic storms, which may disrupt communications and navigation systems. These storms can also create spectacular auroras, a visual reminder of the interplay between solar activity and Earth's magnetic field.
Moreover, understanding the mechanisms underlying the Sunโs magnetic field helps scientists predict its cycles and related solar phenomena. This knowledge is crucial as we venture further into space, aiming for missions to planets like Mars and beyond, where exposure to solar radiation becomes a significant concern.
"Magnetic fields serve as both guides and guardians; they shape the paths of galaxies and protect planets from cosmic threats.โ
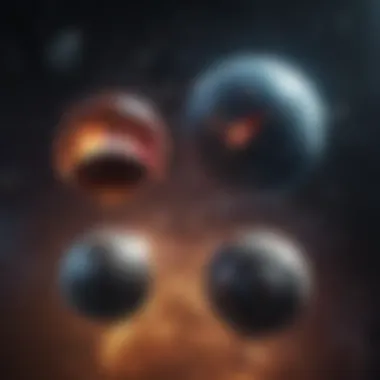
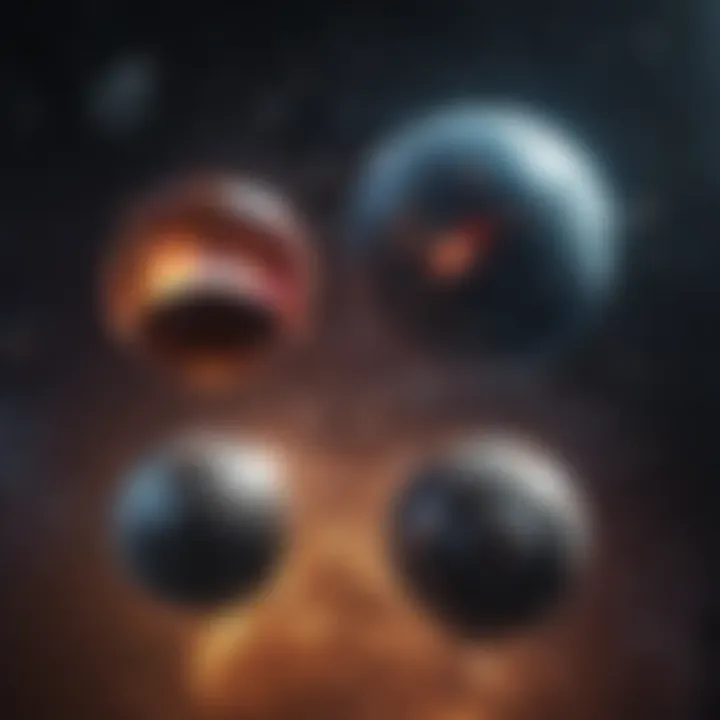
Overall, the study of magnetic fields from this astrophysical perspective is not just academic; itโs essential for future explorations and understanding of our universe.
Planetary Magnetic Fields: Earth and Beyond
Understanding planetary magnetic fields is crucial for several reasons. They serve not just as protective barriers against cosmic radiation, but also influence various atmospheric and geological processes on planets. For Earth, its magnetic field is often taken for granted; however, it plays an essential role in shielding the planet from harmful solar winds, which could strip away layers of the atmosphere and create hostile conditions for life. Similarly, examining the magnetic fields of other planets expands our comprehension of planetary formation and the potential for habitability across the universe.
The Earthโs Magnetic Field: Formation and Function
The Earthโs magnetic field originates from the movement of molten iron and nickel in its outer core, creating a dynamo effect. This process is a bit like swirling a spoon in a cup of coffee, generating a magnetic field surrounding the planet. The magnetic poles are not fixed; they wander over time due to changes in the flow of molten metal beneath the Earthโs crust. This ever-changing nature has implications for navigation systems as well as the behavior of satellites in orbit.
Forming a protective barrier, the Earthโs magnetic field deflects charged particles from the sun, safeguarding both living organisms and technological systems. Without this magnetic shield, life as we know it could be vastly different or even nonexistent. The creators of global positioning systems, or GPS, must consider these magnetic variations when designing their technology to maintain accuracy.
Comparing Planetary Magnetic Fields
Planetary magnetic fields vary widely across the celestial landscape, each with unique characteristics that reflect their planetary environments. The comparison of these fields highlights the diversity of magnetic forces at play throughout our solar system and beyond.
- Terrestrial Planets: Mercury has a weak magnetic field, likely due to its small size and slow rotation. Meanwhile, Venus, despite being similar in size to Earth, lacks a significant magnetic field entirely. This absence can be attributed to its dense atmosphere and slow rotation.
- Gas Giants: On the other hand, Jupiter possesses a powerful magnetic field, much stronger than Earthโs. It extends well beyond its atmospheric boundary and affects its moons vastly. The interaction with Io, one of its moons, produces spectacular auroras, illuminating the polar regions.
- Ice Giants: Uranus and Neptune present peculiar magnetic fields that are inclined at strange angles relative to their rotational axes. This misalignment raises questions about their internal structures and the dynamics of their atmospheres.
"Understanding planetary magnetic fields unveils the underlying processes that dictate not only how planets evolve but also their capability to support life."
In summary, studying planetary magnetic fields equips us with insights into a planet's history and its potential habitability. The variations across different celestial bodies prompt further investigation into the relationship between magnetic fields and the existence of life, deepening our understanding of the cosmos.
For more insights, you can visit Wikipedia or explore related discussions on Reddit to expand on these fascinating aspects.
The Interaction of Solar Wind and Magnetic Fields
Understanding how solar wind interacts with magnetic fields is crucial in appreciating the myriad dance of cosmic forces shaping our universe. This interaction influences not just the space environment around planets, but also affects satellite operations, astronaut safety, and even our Earthly weather patterns. As magnetic fields emanate from celestial bodies, they engage with the charged particles of the solar wind, creating a symphony of reactions that can lead to spectacular phenomena such as auroras and can also pose significant challenges to space exploration.
Understanding Solar Wind
Solar wind, in simplest terms, is the stream of charged particlesโmostly electrons and protonsโemitted by the surface of the Sun. Think of it as the solar equivalent of a breeze, but a mighty one, traveling across the solar system at speeds topping 400 kilometers per second. This stream of particles is not constant; it varies in intensity depending on solar activity, such as solar flares or coronal mass ejections.
The solar wind plays a significant role in shaping the space environment. It carries the Sun's magnetic field into interplanetary space, forming what is known as the heliosphere, a vast bubble around the solar system. Engaging with the magnetic fields of planets, the solar wind helps define the boundaries of planetary magnetospheres.
This interaction is not just a scientific curiosity. In fact, it has tangible consequences for humanity. For example:
- Satellite Disruption: Increased solar wind activity can interfere with satellite operations, affecting communications and GPS systems.
- Space Weather Forecasting: Understanding solar wind dynamics enables scientists to predict space weather, protecting astronauts and technologies on Earth.
The complexities of solar wind provide a rich ground for research, helping unlock further secrets about our cosmic neighborhood.
Magnetosphere: Earth's Protective Shield
Earth's magnetosphere is a formidable force, acting as a shield that protects our planet from the solar wind's incessant barrage. Generated by the movement of molten iron in Earthโs outer core, the magnetic field extends into space, forming a bubble that deflects the majority of incoming solar particles.
When the solar wind encounters this shield, it distorts the magnetic field, compressing it on the sunlit side and creating a long tail on the opposite side. This striking configuration is crucial for keeping harmful solar radiation at bay and maintaining the atmospheric integrity essential for life.
Some of the key aspects of this protective shield include:
- Auroras: When solar wind particles collide with Earth's magnetic field lines, they can enter the atmosphere near the poles, resulting in the stunning displays of light known as auroras.
- Radiation Belts: The magnetosphere traps some of the solar wind's particles, creating zones of radiation known as the Van Allen radiation belts, which protect the surface from high-energy cosmic rays.
"Earth's magnetosphere is a testament to the complex interplay of magnetic forces and solar radiation, ensuring a safe haven for life on our planet."
While the magnetosphere does an impressive job, it is not foolproof. Periods of heightened solar activity can lead to geomagnetic storms, which can disrupt power grids and pose risks to astronauts and spacecraft systems. Thus, recognizing and understanding the dynamic interaction between solar wind and Earth's magnetic fields is vital for both protecting current technologies and planning future explorations beyond our planet.
Applications of Magnetism in Space Exploration
The utilization of magnetism in space exploration is not just a curious point of interest, but a pivotal aspect that governs how we design, navigate, and communicate in outer space. When we talk about magnetism in this context, weโre not merely referencing an abstract physical principle. Rather, we enter into the realm where theoretical constructs meet practical applications, thereby impacting the entire framework of space missions.
The importance of this topic lies in its multifaceted nature. One significant element is the way magnetic fields are harnessed to protect spacecraft from the harsh conditions of space. For instance, the design considerations must take into account the interactions between charged particles from the solar wind and the magnetic fields generated by spacecraft.
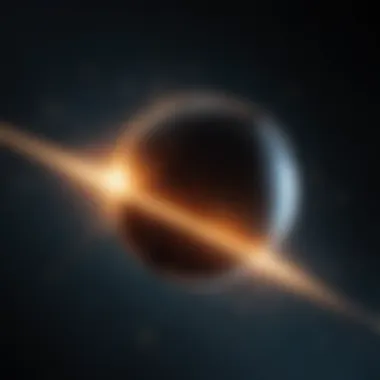
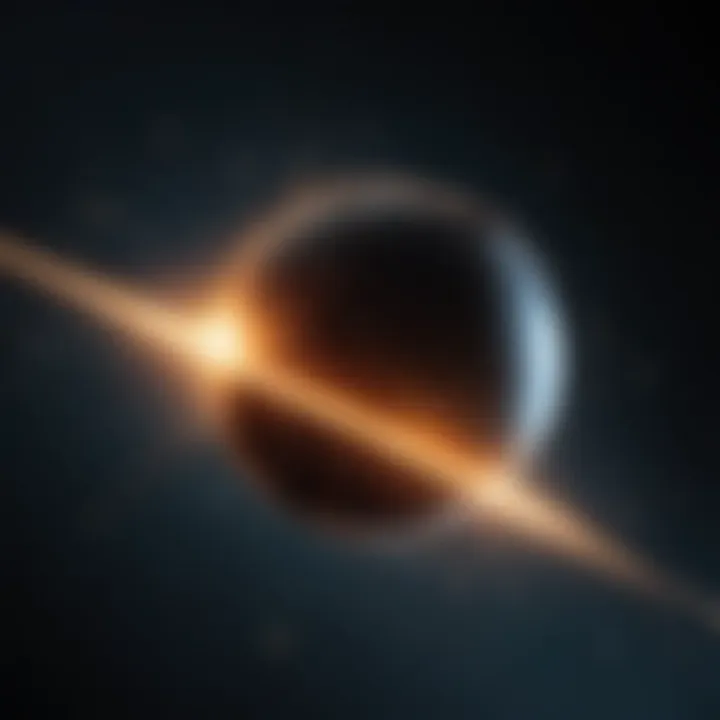
Moreover, these considerations open up a discussion on how magnetic forces can assist in maneuvering spacecraft efficiently, affecting everything from trajectory paths to energy consumption. Itโs a thing that affects both costs and mission success rates, making it a critical area of focus for engineers and researchers alike.
Designing Spacecraft with Magnetic Considerations
When engineers set out to design spacecraft, the impact of magnetism cannot be understated. In areas like shielding, the magnetic field generated by the spacecraft can be employed to deflect incoming solar wind particles, which could otherwise compromise the onboard instruments and the missionโs integrity.
Some specific elements include:
- Material Selection: Choosing materials that effectively interact with magnetic fields can enhance protection against cosmic radiation.
- Magnet Placement: The strategic placement of magnets can optimize interactions with external magnetic fields and improve the overall stability of the spacecraft.
- Performance Testing: Ongoing assessments during the design phase help to understand how the spacecraft will perform in a dynamic magnetic environment.
The goal here is to create a vehicle that remains resilient while navigating through unpredictable magnetic phenomena across various celestial landscapes.
Magnetic Navigation Systems for Spacecraft
The concept of navigating through space using magnetic forces may sound like something out of a science fiction novel, but it has profound implications in our current technological landscape. Magnetic navigation systems can help spacecraft determine their location and orientation with greater precision. This becomes especially relevant when we consider the vast distances and complex gravitational interactions present in space environments.
Key benefits of magnetic navigation include:
- Increased Accuracy: Traditional navigation systems can struggle with uncertainties in the vastness of space. Integrating magnetic navigation can enhance overall accuracy.
- Redundancy: In case electronics fail, a well-designed magnetic navigation system can serve as a failsafe.
- Energy Efficiency: Utilizing magnetic fields could lead to more energy-efficient maneuvers, hence extending the operational lifespan of missions.
"Incorporating magnetism into navigation systems alters our capabilities and helps ensure the longevity and success of space missions."
In summary, the applications of magnetism in spacecraft design and navigation can significantly reshape our approach to space exploration. The depth of research and attention to magnetic interactions represents a crucial investment in the pursuit of understanding the universe. With a keen focus on these applications, we stand to not only enhance existing technologies but propel future explorations into uncharted territories.
Magnetism and Cosmic Phenomena
Magnetism in cosmic contexts is a bridge between observable phenomena and fundamental physics. Understanding how magnetism interacts with various astronomical entities sheds light on the universe's mysteries. In this discussion, we explore how magnetic forces not only shape celestial bodies but also influence the behavior of matter and energy in deep space. To not lose sight of the big picture, we dive specifically into pulsars and black holes, both of which present fascinating cases showcasing the significance of magnetism in the cosmos.
Pulsars and Their Magnetic Fields
Pulsars are rapidly rotating neutron stars, known for their lighthouse-like beams of radiation. The magnetic fields of these entities are exceptionally strongโup to a trillion times greater than Earth's. This immense magnetism plays a critical role in their behavior. The rotation of a pulsar can create intense electromagnetic radiation. As the pulsar spins, it emits beams of radiation that sweep across space, creating a rhythmic pulse detectable from immense distances.
The generation of magnetism in pulsars arises from the collapse of massive stars. When a star exhausts its nuclear fuel, its core collapses under gravity. This collapse greatly compresses its magnetic field, intensifying it dramatically. The resulting magnetic structure is complex. For example, the strongest known pulsar, PSR J1748-2446ad, has a magnetic field trillions of times that of Earth, and when scientists study it, they gain insight into the unresolved aspects of physics, including the state of matter under extreme conditions.
- Key Elements of Pulsar Magnetism:
- Strong magnetic fields influencing radiation patterns.
- Insights into atomic behavior under extreme densities.
- Detection methods assisting in mapping cosmic phenomena across vast distances.
Black Holes and Magnetic Accretion Disks
Black holes, the enigmatic regions in space where gravitational forces are so strong that nothingโnot even lightโcan escape, also showcase the significant role of magnetism. Surrounding a black hole is often an accretion disk, composed of gas and debris spiraling into the event horizon. Intriguingly, itโs here that magnetic fields come into play.
The interplay between the accretion disk's matter and the black holeโs immense gravity generates heat and produces electromagnetic radiation. Additionally, the magnetic fields are crucial for the dynamics and evolution of the disk itself. They act to channel the infalling material, potentially leading to phenomena like relativistic jets, which are streams of particles ejected at nearly the speed of light.
- Considerations in Black Hole Magnetism:
- Magnetic fields influence material inflow and outflow dynamics.
- Theoretical models address energy extraction from spinning black holes.
- They offer clues to the nature of space-time and fundamental forces.
"Magnetism shapes the narrative of cosmic evolution and helps unravel intriguing mysteries of the universe."
The unfolding research about pulsars and black holes serves as a key to advance our understanding of astrophysical processes and fundamental physics. Each discovery not only enhances our comprehension of magnetism's role in the cosmos but it also propels the field of astrophysics forward, piecing together the intricate puzzle of our universe.
Magnetic Fields and Exoplanets
Exploring the magnetic fields surrounding exoplanets is crucial for our comprehension of these distant worlds. The sheer variety of conditions that exist on exoplanets can be observed and understood better through their magnetic characteristics. The significance of magnetic fields ties closely into subjects such as habitability, atmospheric retention, and protection against solar and cosmic radiation. Understanding these aspects can reveal whether a planet might host life or support human exploration in the future.
Detecting Magnetic Fields Around Exoplanets
Detecting magnetic fields around exoplanets is no simple feat. Scientists utilize several methods to identify and study these magnetic phenomena. The primary techniques include:
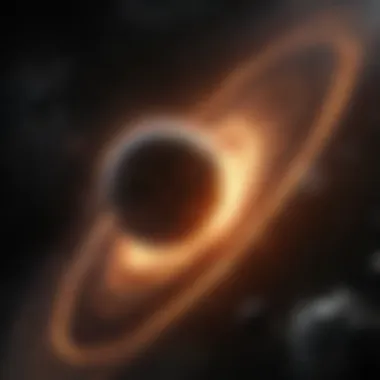
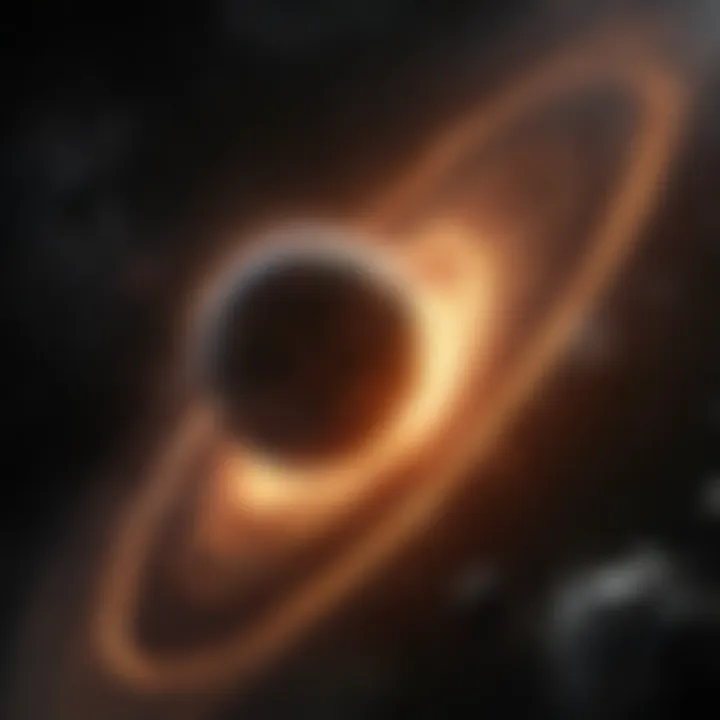
- Stellar Magnetic Activity: The interaction between a star's magnetic field and its planets can provide clues. When a planet transits in front of its star, the way the starโs light changes can indicate magnetic influences.
- Radio Emissions: Some exoplanets send out radio waves due to their magnetic fields. Researchers have been employing radio telescopes to catch these signals. This method is becoming a fruitful avenue in understanding exoplanetary magnetism.
- Magnetospheric Imaging: New imaging techniques being developed allow scientists to visualize the magnetic environment surrounding exoplanets. This method enriches our understanding with more data about how these magnetic fields interact with stellar wind and other cosmic phenomena.
These methods not only shed light on the characteristics of exoplanets but also their potential for hosting life. It's worth noting that even a weak magnetic field can play a significant role in protecting a planet's atmosphere from stripping away, which could otherwise make it uninhabitable.
The Habitability of Exoplanets and Magnetic Protection
The relationship between magnetic fields and the habitability of exoplanets is a fascinating topic in astrobiology. A robust magnetic field can act as a shield, safeguarding a planet's atmosphere from solar winds and cosmic radiation. Without this protection, water, a key ingredient for life, could evaporate or be stripped away over time.
Some considerations include:
- Atmospheric Retention: A strong magnetic field can help maintain an atmosphere. For instance, Mars, which has a tenuous magnetic field, has a thin atmosphere that makes sustaining liquid water a challenge.
- Radiation Shielding: Planets with substantial magnetic fields can deflect harmful solar particles, reducing the radiation exposure on the surface. This could be a decisive factor for potentially habitable zones around stars.
- Potential for Life: The search for life on exoplanets often starts with checking their magnetic characteristics. A planet's ability to maintain its atmosphere and surface conditions suitable for life hinges on its magnetic field.
In summary, the investigation of magnetic fields around exoplanets not only deepens our understanding of these distant celestial objects but also highlights the broader implications for habitability and exploration within our universe.
"The tangled interplay between an exoplanet's magnetic field and its environment can either foster or inhibit the conditions necessary for life."
By continuing our explorations and employing innovative detection techniques, we might unveil the secrets these exoplanets hold for possible future studies and discoveries.
Challenges of Studying Magnetism in Space
Understanding magnetism in the cosmos presents notable hurdles for scientists and researchers. Despite the fascination of this topic, the intricacies of magnetic phenomena in space are often overshadowed by technological and analytical challenges. For those immersed in the study of astrophysics, grasping these challenges is essential, as they affect our broader understanding of celestial bodies and their magnetic interactions.
Instruments for Measuring Magnetic Fields
Measuring magnetic fields in space is no walk in the park. Instruments designed for this purpose need to operate under extreme conditions, such as varied temperatures, vacuum, and intense radiation.
- Fluxgate Magnetometers: These devices detect and measure magnetic fields with high precision. They work by using a magnetic core to sense changes in the magnetic field, allowing for accurate readings even from distant celestial bodies.
- Search Coil Magnetometers: A simpler yet effective tool, this instrument relies on Faraday's law of electromagnetic induction. It provides real-time data on fluctuating magnetic fields, though it might not reach the accuracy of fluxgate models.
- Satellites and Probes: Missions like the Parker Solar Probe and the Magnetospheric Multiscale mission are equipped with specialized magnetometers to gather data on solar and planetary magnetic fields. The satellite's ability to travel through different regions of space enhances the breadth of magnetic studies.
Each of these instruments carries its own set of challenges. Calibration in space can be tricky, and each device needs to be resilient enough to endure the harshness of its environment while delivering consistent results. A slight deviation in readings can lead to significant misinterpretations, complicating any ensuing analysis.
Data Interpretation and Its Complexities
Once data is gathered from instruments, the next step is interpretationโa process that can be akin to navigating a labyrinth without a map. The information harvested from magnetic field measurements can be complex, often requiring advanced mathematical and statistical techniques to make sense of it.
Consider the following:
- Variability in Data: Measurements can shift based on local space weather conditions, solar activities, or even the presence of other magnetic fields. Researchers need to account for these variations, which can muddy the interpretation.
- Modeling Challenges: Creating accurate models of magnetic fields might involve using theoretical frameworks that are not always straightforward. Factors like turbulence and interactions among fields can complicate the modeling efforts.
- Integration with Other Data: Correlating magnetic data with other astrophysical observations, such as temperature or plasma density, is crucial but can also convolute interpretations. The relationships aren't always linear or easily discernible, demanding a multifaceted approach.
"Challenges in data interpretation are not just about numbers. Theyโre about piecing together a larger narrative of how magnetism influences our universe."
In essence, while the instruments allow us to measure magnetic fields, the interpretation of that data presents its own set of battles. Researchers often spend as much time wrestling with analytical methods as they do collecting magnetic field measurements.
In summary, exploring the challenges of studying magnetism in space reveals not just what is being faced, but also the potential that lies at the intersection of instrument design and data interpretation. The vastness of space continually pushes the limits of our understanding, making it an exciting yet demanding frontier.
Future Directions in Space Magnetism Research
The field of magnetism in space has come a long way since the early days of space exploration. As we delve deeper into the cosmos, understanding magnetic fields is not just an academic exercise; itโs crucial for future missions and explorations. The and of space are not merely backgrounds but dynamic entities that have profound effects on everything from planetary atmospheres to the safety of astronauts.
Emerging Technologies in Magnetism Studies
As researchers push the boundaries, innovative technologies emerge that revolutionize how we study space magnetism.
- Satellite-Based Instruments: Satellites equipped with advanced sensors allow scientists to measure and monitor magnetic fields in real-time. These tools can capture fluctuations that ground-based observatories might miss.
- CubeSats and Small Satellites: Smaller, more cost-effective satellites are becoming increasingly popular for studying local magnetic fields around celestial bodies. Their ability to work in swarms enhances data collection immensely.
- Teleportation of Magnetic Data: Imagine being able to send magnetic field data instantaneously back to Earth. Emerging quantum technologies hint at this capability, which could transform our understanding of transient phenomena in space.
These and other technologies hold promise for unveiling details about cosmic phenomena that remain enigmatic. By leveraging these tools, scientists hope to develop more accurate models of magnetism in various space environments, paving the way for safer and more efficient exploration.
The Promise of International Collaborations
The exploration of magnetism in space is no small feat. It requires a concerted effort across borders, involving various nations and organizations. International collaborations can yield vast benefits:
- Shared Resources and Expertise: Different countries possess unique capabilities and knowledge. By pooling these resources, research teams can tackle more complex problems than they could on their own.
- Standardizing Measurement Techniques: When multiple agencies work together, they can refine measurement methods for magnetic fields. This standardization enhances data comparability and reliability.
- Joint Missions: Missions like the European Space Agency's Swarm project demonstrate how collective endeavors can lead to groundbreaking discoveries. By working together, nations can share the costs and risks of space missions, making ambitious projects feasible.
"United we stand, divided we fall" โ this adage rings especially true in the realm of space research, where cooperative effort can lead to a greater understanding of our universe.
In summary, the future of space magnetism research hinges on embracing emerging technologies and fostering international collaborations. With these strategic approaches, we can look forward to unveiling new mysteries of magnetic forces that dance across the vastness of space.