The Evolution and Impact of Largest Computers in History
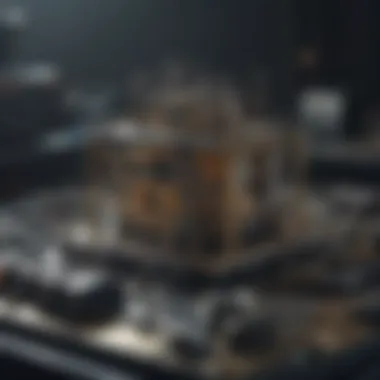
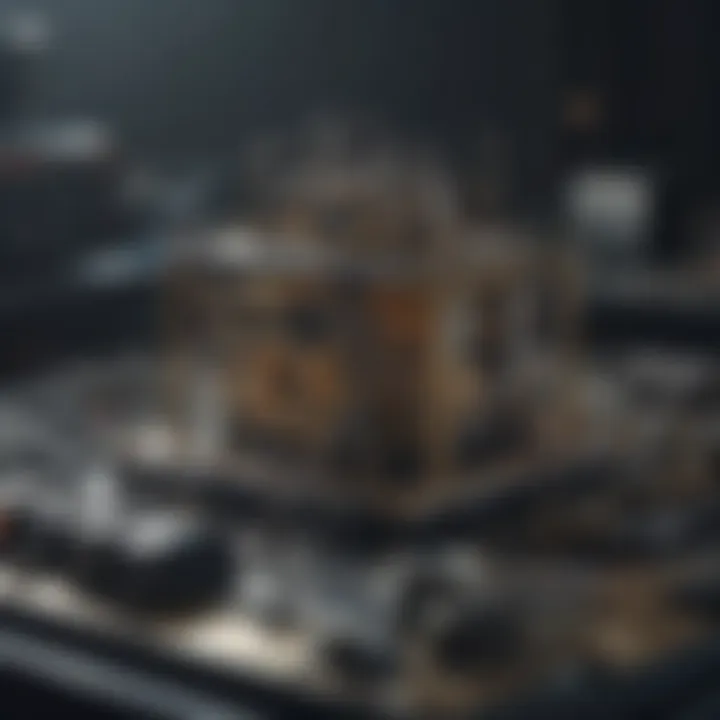
Intro
The landscape of computing has undergone radical changes since the inception of the first computers. Each leap forward has been accompanied by significant growth in computational power, size, and potential applications. Early mechanical systems paved the way for electronic computers, which paved the way for colossal supercomputers that dominate today's tech landscape.
These machines have not only advanced in sheer size and speed, but they've also carved out pivotal roles in various sectors. From scientific research pushing the boundaries of human knowledge to industrial applications optimizing efficiency, the influence of the largest computers is profound. In exploring this journey, we uncover not just technological advancements but also the socio-economic implications of these powerful machines.
Whether it's weather forecasting, simulating complex biological systems, or facilitating secure communications, the impact of large-scale computing is indisputable. Understanding this evolution is key to appreciating how these tools shape our daily lives and the world around us.
Key Research Findings
Overview of Recent Discoveries
In recent years, several intriguing developments have emerged in the realm of large computing systems. Innovations like quantum computing and neuromorphic computing are challenging what we understand about computational capabilities.
- Quantum Computers: They leverage the principles of quantum mechanics and promise to solve problems deemed intractable for classical computers. For example, Google claims to have achieved quantum supremacy with their Sycamore processor, which performed a specific task faster than the fastest classical supercomputer.
- Neuromorphic Computing: This approach mimics the human brain’s architecture and processes information in ways traditional computing can't. With systems like IBM's TrueNorth chip, researchers are delving into energy-efficient computation for AI applications.
These discoveries signal not just a step forward in computational speed but also a shift in how we think about problem-solving in a digital world.
Significance of Findings in the Field
The implications of these findings reverberate across multiple fields:
- Scientific Research: The ability to process vast amounts of data rapidly opens avenues in particle physics and genetics.
- Finance: Quantum computing could disrupt financial modeling, creating opportunities for unprecedented risk assessment.
- Artificial Intelligence: Neuromorphic architecture supports more human-like processing, enhancing machine learning capabilities.
Ultimately, these breakthroughs highlight the continuing need for a deeper understanding of computation's evolution—an awareness that fuels further technological advancement.
Breakdown of Complex Concepts
Simplification of Advanced Theories
Many people find advanced computing theories challenging to grasp. Concepts like parallel processing and distributed computing often feel daunting.
- Parallel Processing: This involves dividing tasks into smaller ones that a computer can handle simultaneously, which drastically speeds up processing time. Imagine a chef preparing multiple dishes at once instead of one after the other; that’s parallel processing in computing.
- Distributed Computing: Involves several interconnected computers working on a task, like a team of builders constructing a house together instead of one working alone.
By simplifying these concepts, we can better appreciate the intricacies behind large computing systems and their applications.
Visual Aids and Infographics
Visual aids play a key role in helping audiences digest complex information. Diagrams illustrating the architecture of supercomputers can make understanding easier. Infographics summarizing the evolutionary timeline, from the ENIAC to today’s cutting-edge machines, can serve as quick reference points, enhancing retention and comprehension of the material.
In essence, as we chart the progress of the largest computers, blending visual tools with clear explanations enriches our understanding and appreciation of their role in shaping our world.
"The story of computing is a narrative of relentless pursuit, where each advancement builds upon the last, creating machines that challenge our very notion of possibility."
As we navigate through the evolution and impact of these machines, the stories behind them reveal why they matter not just in labs or data centers, but in the fabric of modern society.
Prelude to Large-scale Computing
Large-scale computing has evolved into a cornerstone of technological advancement, influencing not just the realm of computing but various sectors including research, health, industry, and environmental science. Understanding large-scale computing is essential in grasping how our modern world functions—where data is generated at lightning speed and processed across vast networks. In this article, we delve into the intricacies of large computers, their design, capabilities, and impact on our society.
The significance of this topic can hardly be overstated. Essentially, large computers, often referred to as supercomputers, push the envelope of what computational power means. They enable breakthroughs in fields otherwise hindered by calculation limits. Think of climate modeling, where minute changes in data can have monumental implications on prediction accuracy. Or consider genomic sequencing, where massive datasets can lead to groundbreaking discoveries in medicine. The scope is as vast as it is relevant.
In exploring this topic, a few key elements come to the fore:
- Technological Progress: Understanding large-scale computing illuminates how we transitioned from mechanical to digital systems.
- Real-World Applications: These machines are not just tools but are crucial in saving lives and shaping policy based on the data they provide.
- Future Directions: Grasping current capabilities lays the groundwork for predicting how technology might evolve, especially with trends like quantum computing on the rise.
By dissecting various aspects of large computing, we aspire to enhance knowledge, enabling not just technological adaptation, but fostering a culture of innovation. This exploration will focus on historic evolution, specific milestones, and the myriad ways these extensive systems influence modern life.
Defining Size in Computing
The term 'size' in computing can refer to a number of factors. In many cases, it denotes the physical dimensions of a machine, but more commonly, it refers to its performance capabilities—the sheer processing power and memory that a system can handle. Large computers often operate on massive scales. For instance, consider systems like the Fugaku supercomputer in Japan, which can handle over 442 petaflops, meaning it can perform over 442 quadrillion calculations per second.
The definition of size also encompasses the infrastructure necessary for large-scale computing. This can involve specialized cooling systems to manage heat emissions, vast databases, and connectivity protocols that allow for seamless operation among multiple units.
Historical Context of Large Computers
The journey of large computers can be traced back to the mid-20th century, when machines like the ENIAC marked the dawn of electronic computing. It was a behemoth of its time, occupying an entire room and consuming vast amounts of electricity. These early machines were programmed using cumbersome methods involving punched cards, making them far less user-friendly than today's systems.
As we moved through the decades, the demands for computational power grew rapidly. The introduction of transistors in the 1950s was a game-changer, allowing for smaller, faster machines that paved the way for more powerful processors. With the advent of integrated circuits, computers not only shrank in size but also packed considerably more punch.
The 1980s and 90s witnessed the rise of mainframe computers, which supported bulk data processing for businesses and organizations. These behemoths had the capability to serve multiple users and run complex applications simultaneously. Their influence reached industries like banking and finance, which required efficient transaction processing.
Moving into the 21st century, supercomputers emerged as the ultimate expression of large-scale computing. Setting records for performance, these machines are at the forefront of research in various fields, modeling everything from the universe's behavior at a cosmic scale to the microscopic processes within cells. This historical context not only underscores the rapid evolution of technology, but it also highlights how each advancement has built upon the last, leading us to the highly intricate and powerful systems we have today.
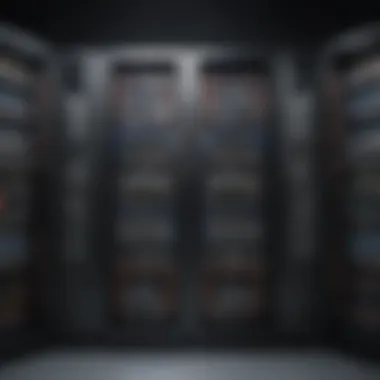
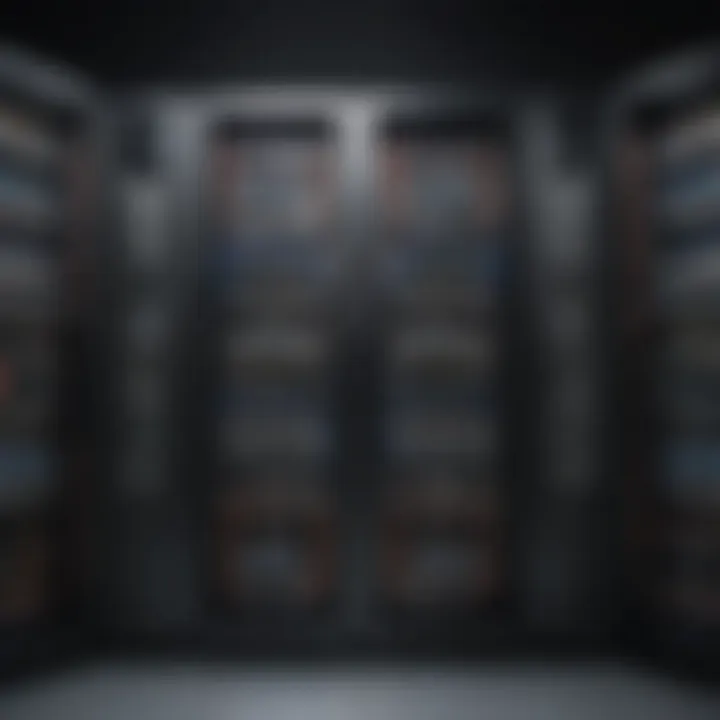
The Evolution of Computers
The evolution of computers is not just a tale of technological advancements but also a reflection of society's thirst for knowledge and efficiency. As we journey through the vast history of computing systems, each milestone reveals how engineers, scientists, and visionaries transformed the landscape of computation. From rudimentary machines to sophisticated supercomputers, this evolution highlights the interplay between innovation and necessity. Understanding this evolution is vital, as it lays the groundwork for the ongoing advancements that continue reshaping fields such as artificial intelligence, climate modeling, and data analysis.
Mechanical Beginnings
The story begins in the 19th century, with mechanical computing devices that laid the foundation for future developments. Charles Babbage's Analytical Engine was a pivotal concept, blending the ideas of automation and computation. Though never completed in his lifetime, the design showcased elements like a programmable interface and memory storage, similar to today’s computers. This kind of visionary thinking highlights how early pioneers grasped the potential for machines to perform tasks beyond simple calculations.
The abacus is often underestimated but played a significant role in early computation. It demonstrated that even basic tools could enhance mathematical understanding and efficiency. Furthermore, mechanisms like the Jacquard Loom utilized punched cards to control complex patterns, foreshadowing future programming methods.
Transistors to Integrated Circuits
With the dawn of the 20th century, significant strides were taken. The invention of the transistor in 1947 marked a transformative leap in computing. Tiny but powerful, transistors replaced cumbersome vacuum tubes, reducing size and increasing reliability. This shift allowed for the development of smaller, more efficient computers and paved the way for the integrated circuit (IC) in the 1960s. These ICs allowed multiple transistors to be embedded on a single chip, revolutionizing not just computers but electronics as a whole.
The implications of these advancements were profound. Gone were the days of machines occupying entire rooms; now, universities and businesses could have computers on their desks. This democratization of computing power opened doors to vast untapped areas in research and science, enhancing fields ranging from weather forecasting to inventory management.
Rise of Supercomputers
In the latter part of the 20th century, a new breed of computers emerged—the supercomputer. These colossal systems were designed to tackle complex calculations at lightning speed, making them indispensable in specialized fields. Starting with the CDC 6600 in 1964, which could perform up to three million instructions per second, the supercomputer has evolved remarkably over decades.
What makes supercomputers particularly fascinating is how they harness parallel processing capabilities to divide and conquer large datasets. Notable examples include the IBM Blue Gene and Fugaku, which have made amazing contributions to scientific discovery. They power climate modeling, genetic research, and simulations that can predict viral outbreaks.
"The effective use of supercomputers accelerates the pace of research, shaping understanding in areas previously thought insurmountable."
In sum, the evolution of computers showcases an incredible journey of ingenuity and progress. From mechanical systems to integrated circuits and onto supercomputers, each phase reveals how technology continuously adapts and meets the world's needs. As we move forward, it’s crucial to recognize these historical milestones; they are the building blocks of the future"s technological landscape.
Key Milestones in Computer Development
The journey of computing has seen several pivotal milestones, each contributing to the vast landscape of technology that we navigate today. Understanding these key developments is crucial. They not only exemplify the technological turmoil of their times but also serve to highlight the transformative impacts these machines have had on society. Each milestone encapsulates a shift not just in size, but in capability, accessibility, and societal implications. Significantly, these milestones reveal the pathways taken toward fostering collaboration among various fields, from mathematics to engineering, making them relevant to students, researchers, educators, and professionals alike.
ENIAC: The First General-Purpose Computer
The Electronic Numerical Integrator and Computer, better known as ENIAC, marks a watershed moment in computer development. Developed in 1945, it represented a formidable leap from mechanical calculators to a fully electronic computing system capable of performing a wide range of tasks. ENIAC was not just impressive for its size—consuming over 1,800 square feet and weighing nearly 30 tons—but also for its functionality.
This computer operated on a decimal system, processing data at speeds previously unimaginable. One of the notable aspects of ENIAC’s design was its programmable capability, allowing it to execute different tasks without physical rewiring. This milestone underscored the importance of programmability in computing, a concept that is now fundamental in today's computational paradigms.
"ENIAC was a demonstration that machines could perform complex calculations faster than any human, opening the floodgates for the future of technology."
IBM and the Mainframe Era
The 1950s and 1960s heralded the era of IBM, particularly with the launch of the IBM 7094 system. Where ENIAC laid the groundwork, IBM significantly intensified the focus on reliability and efficiency in computing. This model showcased innovations such as multiprogramming, where multiple tasks could be processed simultaneously—a significant efficiency leap.
Mainframes became the backbone of many organizations, from governments to financial institutions, transforming how data was managed. These computers helped facilitate the dawn of the information age, allowing vast quantities of information to be processed and stored. The economic implications cannot be overstated; economies began to leverage these systems to enhance productivity and effectiveness in ways previously deemed unattainable.
The Emergence of Quantum Computing
Fast-forward to the 21st century, we witness the rise of an entirely different paradigm: quantum computing. Unlike classical computers, which utilize bits for processing, quantum computers operate using qubits, allowing them to perform multiple calculations simultaneously. This duality of operation introduces unparalleled efficiency, particularly for complex problem-solving in fields such as cryptography or material science.
Current quantum systems, while still in the experimental stage, suggest that they could outperform traditional supercomputers. The implications are profound, touching everything from how we secure online transactions to developing new materials that could revolutionize manufacturing processes.
As we look ahead, distinguishing between these developments emphasizes not only their construction but their broader impact that continues to shape both our industries and daily lives.
Architectural Designs of Large Computers
The architectural designs of large computers play a critical role in defining their performance, functionality, and deployment in various applications. In a time where computational speed and efficiency are absolutely vital, understanding the different architectures becomes essential for both current users and future developers. The right architectural design not only maximizes performance but also impacts scalability and adaptability in rapidly evolving tech environments.
Centralized vs. Distributed Systems
When it comes to large-scale computing, one primary architecture revolves around centralized and distributed systems. Centralized systems consolidate resources in one location, allowing for easier management and centralized control. Imagine a library where all books are packed into one giant room; it’s efficient, yes, but it might also create bottlenecks if too many people try to access the materials at once.
On the flip side, distributed systems scatter resources across many locations, which can bolster performance. This resembles a network of small libraries, each stocked with a unique collection tailored to localized needs. Users can access data simultaneously without hammering one central point. This flexibility can significantly lower the risk of downtime and allows for more effective load distribution.
Each approach has its own benefits and drawbacks. Centralized systems can be simpler to maintain but may succumb to single points of failure. Distributed systems can be fault-tolerant and more efficient but can also lead to increased complexity, especially when it comes to synchronization and network management.
High-Performance Computing Architectures
High-performance computing (HPC) architectures are designed to handle computations that require immense processing power. Here, we typically see a blend of multiple processing units working harmoniously to perform tasks that a single machine would struggle with. The architecture usually includes powerful CPUs, high-throughput storage solutions, and large memory bandwidth.
Modern HPC systems utilize various architectures, such as cluster computing, grid computing, and cloud computing. In cluster computing, individual nodes work together as one powerful system, often connected through a high-speed network. Grid computing, meanwhile, leverages different resources across various locations, consolidating them into a virtual system. Cloud computing simplified this further by offering flexible resources on demand, resembling an on-the-go computing buffet.
Thanks to these designs, large computers are now capable of performing complex simulations, such as predicting the weather or modeling economic scenarios, which require handling massive datasets and conducting innumerable calculations in parallel.
The Role of Parallel Processing
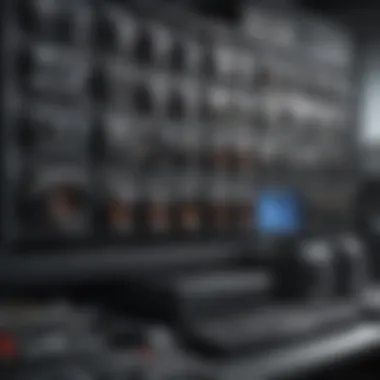
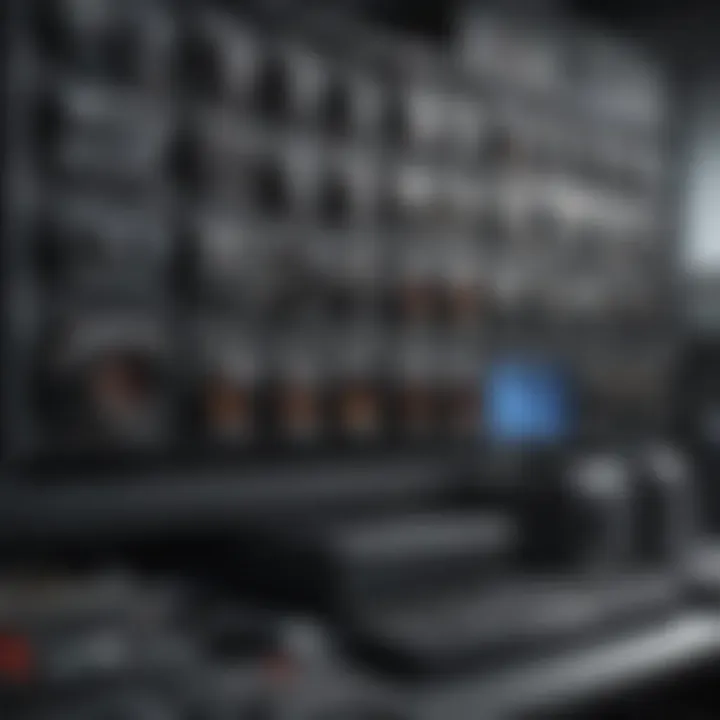
Parallel processing is another linchpin in the design of large computers. This approach relies on executing multiple calculations or processes simultaneously, drastically speeding up tasks that can be divided into smaller, independent sub-tasks. Think of parallel processing like a well-coordinated team of chefs at a restaurant; each chef works on a separate dish, significantly reducing the total time needed to prepare a meal.
In high-performance environments, the ability to crunch data faster means the difference between insight and obsolescence. For example, applications in scientific research, such as genomics or pharmaceutical modeling, utilize parallel processing to analyze vast datasets quickly. By breaking down complex computations into smaller, manageable parts, systems can complete tasks much more efficiently and even tackle problems that were previously deemed unsolvable.
"In an era where time is often equated with value, the ability to process tasks in parallel gives organizations a competitive edge."
In summary, the architectural designs of large computers encompass critical choices between centralized versus distributed systems, the power of high-performance computing, and the effectiveness of parallel processing. Each design plays a pivotal role in shaping how these machines meet the demanding needs of users and industries today, creating a framework that can honed for future developments in computing.
Whether in a research lab or a cloud computing center, knowing these architectural nuances can help make informed choices on the technologies and systems employed to drive innovation.
Applications of the Largest Computers
The role of large computers in the modern world extends far beyond simple calculations; they are the backbone of numerous fields and industries. From scientific breakthroughs to advancements in healthcare, these colossal machines have enabled us to process and analyze staggering amounts of data. Understanding their applications provides insights not only into their technological capabilities but also their potential to drive social progress.
Scientific Research and Data Analysis
Large computers have fundamentally transformed the landscape of scientific research. They are pivotal in handling complex simulations and calculations that were once the domain of theoretical conjectures. For instance, consider the Large Hadron Collider in Geneva, where physicists search for the elusive Higgs boson particle. The data generated, amounting to petabytes, isn't something a regular computer can handle; it requires the power of supercomputers to process and analyze such a vast quantity of information.
Moreover, big data analytics has changed the way researchers approach experiments. With tools powered by large computers, data from various sources can be integrated and analyzed swiftly. This ability not only accelerates the research timeline but also enhances accuracy and reliability of results.
Some key contributions include:
- Complex simulations: Models of physical phenomena like climate change or molecular biology are computed at unprecedented scales.
- Data mining: Enabling researchers to uncover patterns and correlations in large datasets, thus informing decisions in real-time.
"Big data isn't just about the volume of data; it's about the insights we can extract from it."
Climate Modeling and Predictions
In an era where climate change looms as an existential threat, the involvement of large computers in climate modeling cannot be overstated. These machines provide the necessary compute power to run expansive models that simulate the Earth's climate systems over decades or even centuries.
Supercomputers like the Earth System Grid usher in a new wave of climate research through high-resolution simulations. They allow scientists to create predictive models that can forecast weather patterns, helping us prepare for and mitigate the effects of natural disasters. This data can be pivotal for urban planning, agriculture, and disaster response strategies.
Some significant capabilities include:
- Long-term forecasts: Predicting climate trends and their socio-economic impact helps governments and organizations prepare.
- Ecosystem modeling: Helping scientists understand the interconnectivity within ecosystems, critically important for biodiversity conservation.
Impact on Healthcare and Genomics
The healthcare sector has witnessed a revolutionary change with the application of large computing systems. From genomics research to patient data management, these computers significantly enhance our understanding of complex health issues. They enable the analysis of genetic sequences, which has implications for personalized medicine.
By processing vast datasets from medical records, large computers aid in identifying patterns that can lead to breakthroughs in disease prevention and treatment. For instance, the ability for researchers to analyze the human genome was bolstered by supercomputers, making it possible to identify markers for diseases much more quickly than before.
Considerations for healthcare applications:
- Speed of analysis: Faster processing leads to quicker diagnoses and treatment solutions, ultimately saving lives.
- Predictive analytics: By analyzing historical data, computers can help predict outbreaks and manage resources efficiently.
With the ongoing evolution of computational power, the potential applications of large computers in scientific research, climate studies, and healthcare are boundless. Their impact on these areas signifies a key development in our ability to tackle some of the most pressing challenges of our time.
Comparative Analysis of Large Systems
When it comes to large-scale computing, the conversation cannot skip past comparative analysis. Understanding how these behemoth machines stack up against one another illuminates the technological landscape, offering insights into their functionality, utility, and evolution. Just like rivers that carve valleys over millennia, supercomputers have shaped the contours of modern science and industry. Analyses of performance metrics, consumption efficiency, and cost versus performance guide decision-makers, researchers, and engineers in navigating this complex realm.
In addressing this topic, we not only recognize the sheer size and capability of these systems but also focus on their impact in real-world applications. By scrutinizing various elements, we uncover the factors that contribute to effective computing solutions. This is essential, given that today’s technological advancements must not only be powerful but also sustainable and economical.
Top Ten Supercomputers: Performance Metrics
The race to build the fastest computer showcases a range of metrics that define these machines. Performance often hinges on factors like FLOPS (floating-point operations per second), which measures a computer's ability to perform complex calculations rapidly. For instance, Fugaku, developed by RIKEN and Fujitsu, has consistently claimed the top spot in supercomputing benchmarks with its impressive capabilities.
Key metrics to consider include:
- FLOPS Measurement: Top-tier systems often exceed an exaflop, meaning they can perform at least 10^18 FLOPS.
- Memory Bandwidth: The speed at which data can be read from or written to memory plays a crucial role in performance.
- Processing Power: The number of CPUs and GPUs can directly influence computational speed.
Analyzing these metrics reveals the underlying engineering choices and the trade-offs made. It helps clarify not only speed but also reliability and scalability in handling large datasets.
Power Consumption and Efficiency
Power consumption is an essential consideration in the deployment of large computing systems. While performance is the buzzword, efficiency in power usage often dictates long-term sustainability. Comparison metrics like performance-per-watt gain traction here, emphasizing how many computations can be performed per unit of energy consumed.
For instance, systems like Summit, developed by IBM, illustrate the demon that lurks behind the power-saving facade. Despite its incredible capabilities, its thirst for energy places it under scrutiny. Addressing efficiency means prioritizing not just raw computational ability but also the operational costs and environmental impact. Some alarming figures include:
- Total Energy Consumption: Leading supercomputers can consume megawatts of power, driving up operational costs.
- Cooling Requirements: A large part of energy consumption goes into keeping these systems cool, reinforcing the importance of efficient designs.
Therefore, fostering energy-efficient computing remains vital for the future.
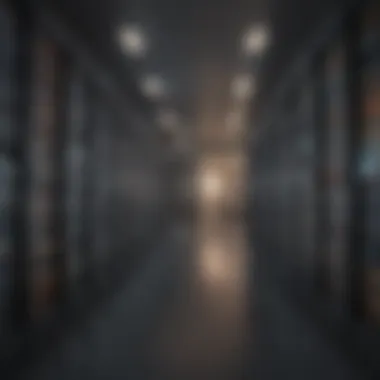
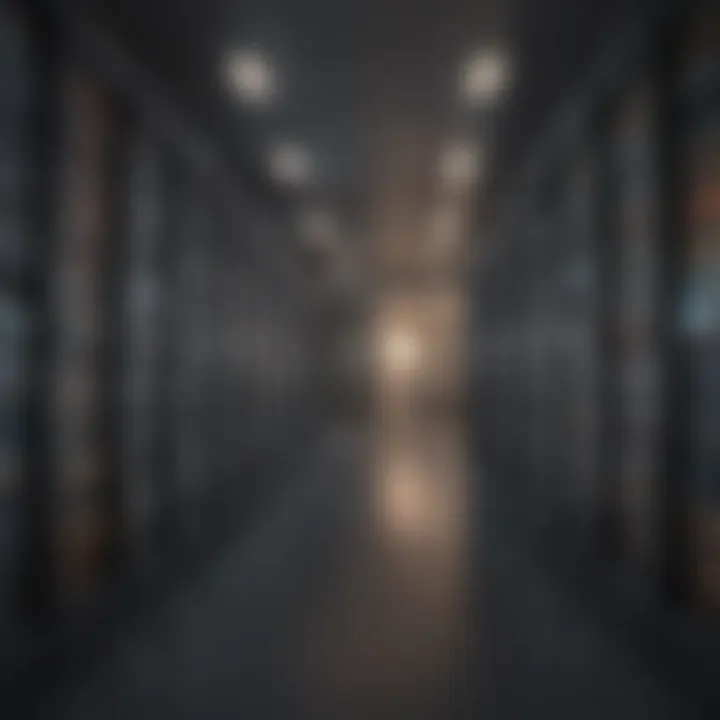
Cost vs. Performance Analysis
The balance between cost and performance can resemble a high-stakes game of chess. Investing in a cutting-edge supercomputer can run into the billions, and stakeholders must weigh the return on investment carefully. The challenge lies in finding a sweet spot that satisfies performance needs without breaking the bank.
Key elements in this analysis encompass:
- Initial Costs: From development to installation, the seed cost can be astronomical.
- Operational Costs: Beyond just the sticker price, ongoing maintenance, power, and cooling expenses keep the financial strain high.
- Performance Upgrades: As technology advances, adaptation can require additional investment to keep pace with competitors.
By dissecting cost versus performance, organizations can ascertain whether a high-performance system delivers a bang for their buck. The stakes are high, as the wrong choice can lead to wasted resources and missed opportunities.
Achieving a thorough comparative analysis of large computer systems not only facilitates better choices but also harnesses technology's multifaceted nature, ultimately aiming for innovation that benefits society.
Challenges in Building Large Computers
The journey of creating large computers is riddled with challenges that require expertise and innovative solutions. Understanding these challenges helps comprehend the scale and complexity tied to such monumental machines. The larger the machine, the more intricacies come into play. Here we delve into three specific issues that developers and engineers face when building these giants: technology limitations, maintenance and reliability issues, and environmental concerns.
Technology Limitations
Technology is the backbone of any computer system, but when it comes to building large computers, certain limitations pose significant hurdles. For instance, the miniaturization of components has made it possible to pack more processing power into smaller devices. However, as developers push the boundaries of what technology can achieve, they often encounter physical and engineering constraints.
- Heat Management: Large computers generate enormous amounts of heat, which needs to be managed effectively. Traditional cooling methods may not suffice when systems operate at high performance for extended periods.
- Scalability: As more cores and functionalities are added to a system, ensuring that all components work together seamlessly becomes increasingly difficult. Architectures may need to evolve continuously to keep pace with growing demands.
- Power Supply: Meeting the power requirements for massive systems can be problematic. Not only does it involve high costs, but it also raises questions about sustainability.
Maintenance and Reliability Issues
Maintenance of large computer systems is not just a simple task; it’s an ongoing responsibility that involves meticulous planning and execution. Large-scale systems require routine checks to ensure reliability and performance, and this often brings challenges like:
- Downtime: Unlike smaller systems, any malfunction in a large computer can result in significant downtime, impacting users dependent on its operations.
- Complexity of Repairs: When a fault occurs, diagnosing the exact issue can feel like finding a needle in a haystack. The systems are complex, and pinpointing the problems demands specialized skills and tools.
- Lifecycle Management: Keeping track of the system's performance over time and making informed decisions about upgrades or replacements only makes the maintenance process even more intricate.
"The journey of technology is often a balancing act between what is possible and what is practical."
Environmental Concerns
Building large computers doesn't just come with technical obstacles; it also raises environmental considerations. The impact of these machines on the environment cannot be brushed aside:
- Energy Consumption: Large computing systems can consume vast amounts of electricity, leading to increased carbon footprints. This is a pressing issue in an era where energy efficiency is paramount.
- E-Waste: The lifecycle of large computers often results in substantial electronic waste. As components become obsolete, proper disposal or recycling of these materials becomes crucial to minimize pollutants.
- Resource Usage: The metals and materials needed to construct these systems can lead to resource depletion if not sourced sustainably.
In summary, the challenges in building large computers are multifaceted and ever-changing. Recognizing these hurdles is essential not just for engineers but for anyone invested in the field of computing technology. Tackling these issues ensures that future innovations can push boundaries while remaining mindful of their impact on society and the planet.
Future Directions in Computing Size
The landscape of computing is in a constant state of flux, propelled by rapid advancements in technology and the urgent demands of contemporary society. As we navigate the path forward, understanding the future directions in computing size becomes essential. This section sheds light on key trends, potential integrations, and considerations regarding future computing systems.
Trends in Quantum and Neuromorphic Computing
The rise of quantum computing marks a significant paradigm shift in the world of large computing systems. Unlike classical computers that use binary data, quantum computers harness the principles of quantum mechanics, enabling them to process information in a fundamentally different way. This capability could revolutionize problem-solving in fields such as cryptography, materials science, and complex systems modeling.
Neuromorphic computing, on the other hand, mimics the neural structure of the human brain. These systems are designed to perform tasks like pattern recognition and decision-making much more efficiently than traditional architectures. The convergence of these two technologies can lead to innovations that transcend the limitations of present-day systems. Not only do they promise faster processing speeds but also increased energy efficiency.
"Quantum leap in computing could open doors to untapped potential."
As research intensifies, we may see a fusion of quantum and neuromorphic capabilities, paving the way for computers that are not only powerful but also remarkably efficient.
Integration of AI in Large Systems
Artificial Intelligence is no longer confined to niche applications; it is fast becoming an integral part of large computing systems. When incorporated thoughtfully, AI can enhance the performance, reliability, and usability of these systems. For instance, AI algorithms can optimize resource allocation dynamically, ensuring that computational prowess is maximized without the proportional increase in energy consumption.
Moreover, the integration of AI can help manage and analyze the vast amounts of data generated by large computers. With predictive analytics and machine learning techniques, large systems can identify trends and anomalies efficiently, providing insights that drive informed decision-making in real-world applications.
As we consider the trajectory of computing size, it is clear that AI will be a foundational element that transforms how these machines function and interact with their users. The synergy between massive computational power and intelligent software could create systems capable of tackling societal challenges previously deemed insurmountable.
Potential for Miniaturization
While we often associate size with power, the future could see a paradoxical trend: the miniaturization of powerful computing systems. The advent of advanced materials and fabrication techniques paves the way for smaller, yet highly potent, computational devices. Innovations such as 3D stacking of chips and enhanced photonic interconnects can lead to developments that allow for high-performance systems in more compact formats.
This shift towards miniaturization has far-reaching implications. In remote locations or challenging environments, powerful yet portable computing could become an asset for scientific research, disaster response, and medical technologies. Tiny yet powerful computers can be integrated in everyday objects—think smart appliances or wearables—creating networks of interconnected systems that draw on collective computational strength.
End: The Significance of Size in Computing
Large computers have played a pivotal role in shaping the technological landscape. Their size often mirrors their capacity to handle complex processes and vast amounts of data simultaneously. It’s more than just dimensions; it’s about how the scale impacts performance, efficiency, and capability in various fields. From scientific research to artificial intelligence, the implications of building larger systems stretch far and wide.
One significant aspect worth noting is the advancement of problem-solving capabilities. Large computers enable researchers and organizations to tackle challenges that require immense computational power. For instance, in fields such as climate modeling, the ability to simulate vast and intricate systems can lead to more accurate predictions and better understanding of future scenarios. With the substantial computational strength needed for such tasks, size truly translates to capability in a direct manner.
Furthermore, size plays a key role in the design and architecture of computational systems. The evolution of large computers has influenced how we think about data processing and storage. Architectures such as multi-core processing and distributed systems demonstrate how scaling up can enhance performance and efficiency. Such advancements have given rise to innovative solutions across various industries, from healthcare to finance.
"Large systems are not just about power—they're about possibilities. The potential they unlock is what makes them significant in our moving world."
Additionally, the economic aspect cannot be overlooked. Organizations investing in larger computing systems often find they can reduce time-to-market for critical projects and improve efficiency in operations. While the initial costs can be daunting, the long-term benefits frequently outweigh the investment. Cost efficiency is tied closely with size; larger systems can handle multiple tasks concurrently, which can lead to lower overall operational costs.
Yet, size brings its own set of challenges, including maintenance, energy consumption, and environmental impacts. As these systems grow, their energy demands soar, prompting questions about sustainability in computing practices. Developers and engineers are now tasked with finding a balance between computing power and ecological responsibility. Indeed, the future holds an ongoing dialogue about how we can scale computing without leaving a hefty footprint on our planet.