Historical Earth Temperature: A Comprehensive Overview
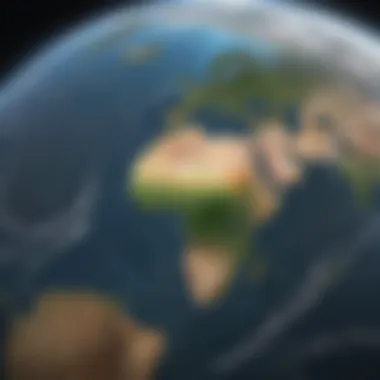
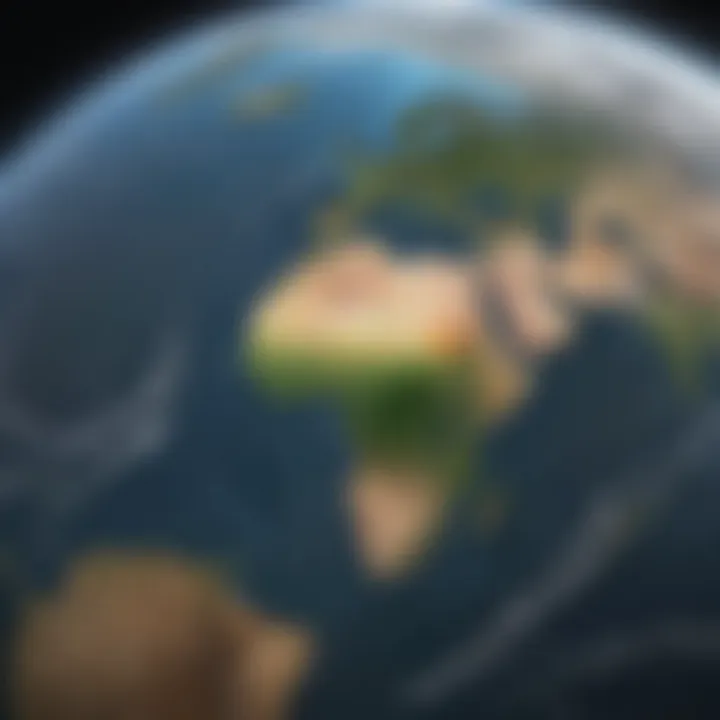
Intro
The exploration of Earth's historical temperature is a vital inquiry for environmental sciences. Understanding how temperature has fluctuated over geological periods enables scientists to draw parallels with present and future climate changes. This article presents a detailed overview of significant temperature shifts, highlighting geological periods and key climate events that have shaped our planet. It integrates diverse scientific findings and analyzes temperature records to illustrate their broader environmental and societal implications.
Key Research Findings
Overview of Recent Discoveries
Recent research has unearthed critical insights into Earth's climatic history. Paleoclimatology, the study of past climates, has provided data through methods such as ice core analysis, sediment core studies, and tree ring data. For instance, studies of ice cores from Greenland and Antarctica have revealed temperature variations corresponding with glacial and interglacial periods. These findings show that dramatic shifts have occurred due to natural factors like volcanic eruptions and solar cycles.
Significance of Findings in the Field
The significance of these temperature records cannot be overstated. The ability to connect historical data to present trends in climate change strengthens the narrative surrounding anthropogenic effects. According to a comprehensive review published in Nature, current temperatures are nearing their highest levels seen in the last 400,000 years. This correlation amplifies urgency for addressing climate-related issues.
The study of historical temperature changes provides a vital context for understanding current climate challenges.
Breakdown of Complex Concepts
Simplification of Advanced Theories
Many theories regarding climate mechanisms can appear complex. For example, the greenhouse effect describes how certain gases trap heat in the atmosphere, leading to warming. Simplifying these theories into fundamental concepts aids better retention and understanding. It's essential for students and professionals to grasp the mechanisms behind these changes while also recognizing how feedback loops can exacerbate warming.
Visual Aids and Infographics
Creating visual aids, such as charts and infographics, can substantiate the textual data provided. For example, comparing temperature records from various geological periods in a graph visually illustrates trends and anomalies. This way, readers can quickly interpret crucial data points without getting lost in dense scientific jargon.
Overall, an understanding of the historical context of Earth's temperatures equips all stakeholdersβstudents, researchers, and educatorsβwith the necessary knowledge to engage in informed discussions about climate policies and future research directions.
Foreword to Historical Earth Temperature
Understanding the historical changes in Earthβs temperature is critical for addressing both contemporary environmental challenges and predicting future climate scenarios. Historical temperature records offer insights into the natural variations of climate over eons, allowing researchers to investigate the patterns and drivers of climatic shifts. This section highlights why studying historical temperatures is not just an academic pursuit but is essential for understanding the broader implications for life on Earth today.
The relevance of examining historical temperature extends to various fields including geography, ecology, and even social sciences. Climate has intricate connections with biodiversity, agriculture, and human health, making the study of temperature history an interdisciplanary task. By deciphering how Earth's climates have fluctuated, we can appreciate how ecosystems have adaptedβor failed to adaptβover time.
Moreover, comprehensive knowledge of Earth's past temperatures informs climate policy and adaptation strategies. As modern society grapples with the repercussions of climate change, understanding historical trends aids in forecasting future patterns. It also provides valuable context for current debates surrounding greenhouse gas emissions and environmental sustainability.
The study encompasses various methodologies and data sources, from proxy records like ice cores and tree rings to direct measurements made by instruments over the past few centuries. Each approach offers its own strengths and weaknesses, contributing to a more nuanced view of temperature history.
Ultimately, the exploration of historical earth temperature can equip students, researchers, educators and related professionals with a deeper understanding of why and how Earthβs climate is changing. Armed with this knowledge, they may engage in informed discussions about environmental stewardship and the importance of preserving our planet for future generations.
Defining Historical Temperature Records
The concept of historical temperature records is crucial to understanding how Earth's climate has changed over time. By defining these records, we can establish a baseline for comparison and analyze deviations that indicate significant climatic shifts. Historical temperature data helps illuminate trends and events that have shaped the ecosystem and human experience. Understanding these records aids researchers in predicting future climate scenarios and informing policy decisions.
Types of Temperature Data
Proxy Data
Proxy data refers to indirect measures of historical temperatures. This can include evidence from ice cores, tree rings, and sediments. Such data provides insight into climate conditions when direct measurements were not available. One key characteristic of proxy data is its longevity. It often extends back thousands or even millions of years. This aspect makes it a beneficial choice for our analysis. The unique feature of proxy data is that it can reflect environmental changes over broad time scales. However, it may have disadvantages: the interpretations of proxy data can be complex and sometimes ambiguous.
Instrumental Records
Instrumental records consist of direct measurements of temperature taken by various instruments from the late 19th century onwards. These records offer precise and reliable information about changes in temperature over time. The key characteristic that sets instrumental records apart is their accuracy and resolution. Because of this precision, they are a popular option in climate studies. They allow for the identification of short-term trends. However, the coverage of these records varies globally, which can create gaps in data and pose challenges in analysis.
Paleoclimate Studies
Paleoclimate studies focus on understanding Earth's past climate through various means, including studying sediment layers, ice core samples, and other geological records. This type of data provides context about previous climates. A prominent feature of paleoclimate studies is their ability to reconstruct climate conditions over much longer periods than instrumental records. This long-term perspective makes paleoclimate data an essential choice for our overall objective. While highly informative, interpreting this data can also be quite complex due to its reliance on indirect measures.
Methods of Data Collection
Tree Rings
Tree rings serve as a biological archive of atmospheric conditions. Each ring represents a year of growth, and the thickness of each ring corresponds to the availability of resources like moisture and sunlight. A key characteristic of tree rings is their annual resolution, providing detailed insights into climate variations year by year. This method is beneficial because trees can provide data dating back thousands of years. However, the interpretation can sometimes be influenced by other environmental factors, such as soil quality or species-specific growth patterns.
Ice Cores
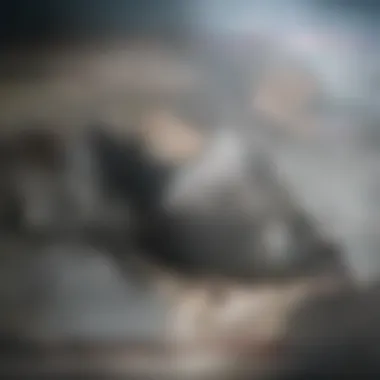
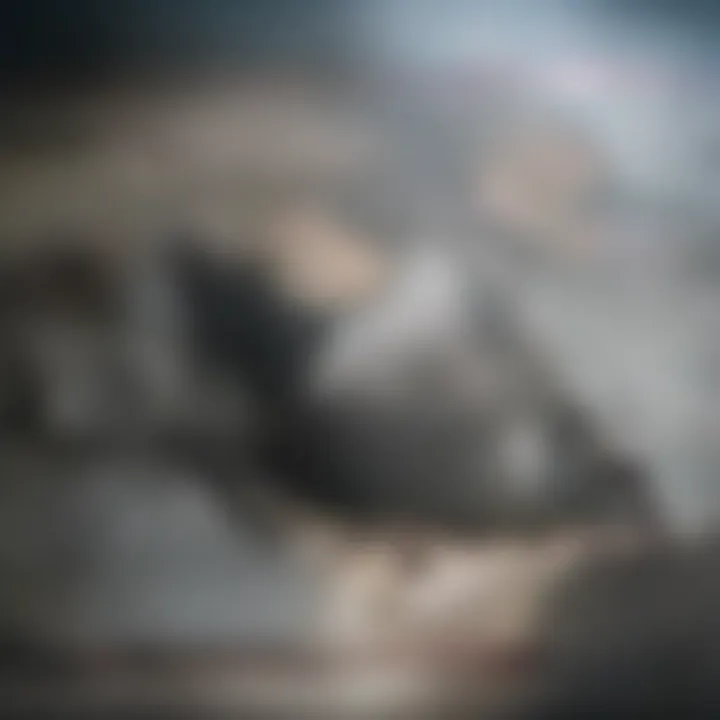
Ice cores are cylindrical samples extracted from ice sheets. They preserve trapped air bubbles and other substances, allowing scientists to analyze ancient atmospheres. One essential characteristic of ice cores is their ability to provide a continuous record of past temperatures and greenhouse gas concentrations. This makes them a popular tool for climate researchers. The unique feature of ice cores is their capability to reach back tens of thousands of years, yielding valuable insights into the Earthβs climatic history. However, the process of extracting and analyzing ice cores can be logistically challenging and costly.
Sedimentary Analysis
Sedimentary analysis examines layers of sediment deposited over time. This method can reveal past environmental conditions through the study of fossilized remains and chemical signatures within the layers. A key characteristic of sedimentary analysis is its capacity to provide long-term climate records. Because sediments accumulate gradually, they can encompass vast periods of time. This method is advantageous as it integrates information from various sources. However, the interpretation of sedimentary records can be complicated, as layers may be disturbed over time, leading to potential misinterpretations.
Understanding these diverse data types and collection methods is essential for reconstructing Earth's climate history. Without such knowledge, our insights into climate change would remain incomplete.
Geological Time Scale and Climate
Understanding the geological time scale is crucial in the study of Earthβs historical temperature. It provides a framework that emphasizes the chronological order of significant climatic and geological events over the vast expanse of geologic time. This topic is not only central to historical climate studies, but it also influences how scientists predict future climate shifts. By segmenting Earthβs history into eons, eras, and periods, researchers can more accurately correlate temperature fluctuations with major geological and biological changes, enhancing our understanding of climate dynamics.
Eons and Eras Overview
The geological time scale consists of four primary eons: Hadean, Archean, Proterozoic, and Phanerozoic. Each eon is characterized by significant geological and biological developments. The Phanerozoic eon, which spans the last 541 million years, is especially notable for the appearance of abundant fossil records and complex life forms. Within the Phanerozoic, the eras are further divided into periods, allowing for a more detailed exploration of climate variability and temperature changes through different epochs.
Major Climate Events
Snowball Earth
Snowball Earth refers to a hypothesis suggesting that during certain periods in Earthβs history, the planet was nearly or entirely frozen. This occurred during the late Proterozoic, around 720 to 635 million years ago. The key characteristic of Snowball Earth is its extensive glaciation, which is supported by geological evidence such as glacial deposits found in tropical regions. This event is beneficial for the article as it showcases extreme climate shifts and the planetβs ability to recover from such conditions. A unique feature of Snowball Earth is the profound impact it had on life, leading to evolutionary changes that would shape future ecosystems. However, the potential disadvantage in emphasizing this event lies in its speculative nature, as some aspects of this hypothesis remain contentious among scientists.
Permian-Triassic Extinction
The Permian-Triassic extinction, occurring approximately 252 million years ago, marks the most significant extinction event in Earthβs history, resulting in the loss of nearly 96% of marine species and 70% of terrestrial vertebrate species. This event is pivotal for understanding Earthβs historical temperature as it was associated with drastic climate changes, including increased volcanic activity which led to elevated greenhouse gas levels. The key characteristic of this extinction is its role in reshaping biodiversity on the planet. Its inclusion in this discourse is popular due to the insights it provides about climate's influence on life and vice versa. A unique feature of this event is the geological evidence it leaves behind, such as sedimentary rock layers that indicate shifts in fauna and flora. The disadvantage here is that the complexity of contributing factors makes it difficult to isolate temperature influences.*
Past Greenhouse Periods
Past greenhouse periods in Earth's history, such as the Cretaceous and Eocene, provide critical insight into the relationship between elevated atmospheric temperatures and ecological conditions. During these periods, global temperatures were significantly higher compared to current levels, impacting species distribution and ecosystem structure. The key characteristic of past greenhouse periods is the prevalence of lush vegetation and diverse life forms in regions that are now temperate or even polar. This is a beneficial aspect for this article as it allows for direct comparisons with current climate conditions and future implications of greenhouse gas emissions. A unique feature of these periods is the existence of fossil records indicating a rich biodiversity that thrived under warmer climates. However, the downside is that projecting future climate change based on past greenhouse warm periods can be complicated due to many variables involved.
The Last Glacial Maximum
The Last Glacial Maximum (LGM) is a significant period in Earth's climate history. It marks the time when ice sheets were at their greatest extent, roughly 26,500 years ago. Understanding this era is essential for scientists studying climate change because it offers insights into extreme climatic conditions and its impact on ecosystems. By examining how ecosystems adapted during this period, researchers can draw parallels to current climate challenges, allowing for a better comprehension of potential future impacts.
Characteristics of the Last Glacial Maximum
During the LGM, global temperatures were significantly lower than today. Ice sheets, particularly in North America and Eurasia, expanded substantially, covering vast land areas. Sea levels dropped due to the vast amounts of water trapped in glaciers. This period was characterized by stark climate zones. Regions now known for temperate weather experienced harsher conditions. Such characteristics highlight the adaptability of the Earth's systems and demonstrate the resilience of different species faced with dramatic changes in their environment.
Impacts on Ecosystems
Flora and Fauna Changes
The LGM brought about major shifts in flora and fauna. Many plant species adapted to cold conditions, with reduced diversity in warmer areas. Arctic tundra expanded, providing habitat for cold-tolerant species. This shift contributed to the overall biodiversity of these regions. The specific aspect of this change illustrates how organisms either migrated to find suitable conditions or adapted to survive. Flora and fauna changes during this epoch highlight the importance of adaptability. Some species thrived in this colder climate, while others did not, which points to the precarious balance ecosystems maintain. The ability to learn from these shifts can inform current conservation efforts.
Migratory Patterns
As climate shifted, so did the migratory patterns of many species. Animals began to move towards areas that provided the necessary resources to survive. These new migratory routes often expanded the territories of various species. The key characteristic of these migratory patterns was that they were often in response to environmental stress. The adjustments made by animals could tell us a lot about how species respond to climate variations. Understanding these patterns is crucial as current climate change continues to affect species movements and behaviors, providing essential insights for future studies.
Habitat Alterations
The habitable areas on Earth were not static during the LGM. As temperatures dropped and ice sheets expanded, habitats changed dramatically. This resulted in habitat alterations where many species either thrived or became extinct. The unique feature of these habitat alterations is that they mirrored changes in temperature rather than merely availability of food. It is vital to acknowledge these changed habitats, as they inform the ecological dynamics and adaptive processes of many life forms. Recognizing how these habitats altered contributes to our understanding of ecological resilience and vulnerability in todayβs climatic shifts.
The Last Glacial Maximum serves not only as a historical benchmark but also as a critical point of reference for understanding how ecosystems can adapt to extreme climatic changes.
The knowledge gained from studying the Last Glacial Maximum equips researchers and policymakers with a deeper understanding of climate dynamics, enhancing the capacity for future climate adaptation strategies.
The Holocene Epoch
The Holocene Epoch, beginning approximately 11,700 years ago, marks a significant period in Earth's climate history. As the most recent geological epoch, it follows the last glacial maximum and is characterized by a relatively stable and warm climate that enabled human civilization to thrive. Understanding the Holocene is essential, as it provides a backdrop for analyzing contemporary climate changes and their potential future impacts. The climatic conditions during the Holocene allowed for the establishment of agriculture, urbanization, and industrialization. Hence, this epoch serves as a critical reference point for environmental and societal studies.
Temperature Trends during the Holocene
During the Holocene, global temperatures have been relatively stable, with variations that can be linked to natural climate cycles. The instrumental record from the past few hundred years shows a gradual increase in temperatures. Notably, the warmest period of the Holocene occurred around 5,000 to 7,000 years ago, known as the Holocene Thermal Maximum. This episode was marked by higher temperatures, which supported diverse ecosystems and human agriculture.
However, recent climate data indicates a significant anomaly. The pace of warming observed in the last century is unprecedented compared to previous temperature trends during the Holocene. This rapid temperature increase highlights the urgency of understanding both past and present climate dynamics.
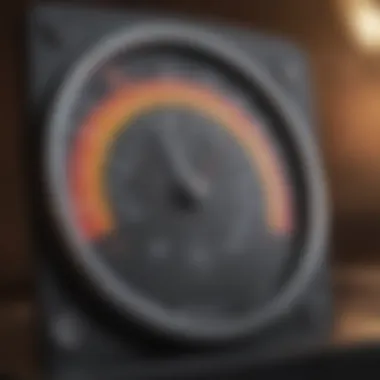
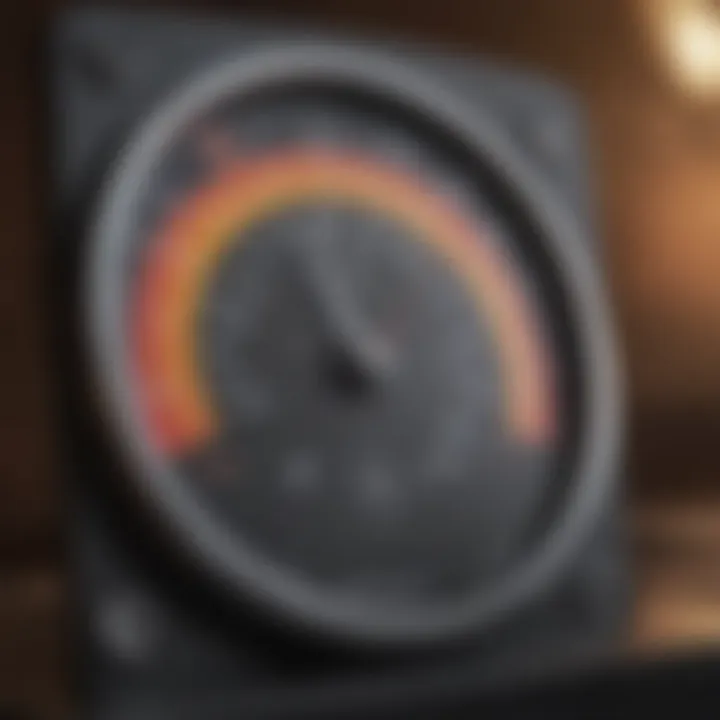
Human Influences on Climate
Human activities have had profound effects on the climate during the Holocene, especially in the last few centuries. Understanding these influences is critical for comprehending the ongoing climate crisis.
Agricultural Practices
Agricultural practices, developed in the Holocene, played a major role in altering land use and ecosystems. The shift from hunter-gatherer societies to settled agriculture allowed humans to modify landscapes extensively. Major crops like wheat and rice, domesticated during this epoch, changed natural environments, leading to deforestation and soil degradation.
One key characteristic of agricultural practices is their ability to support larger populations. However, as farming expanded, so did its environmental impact. The expansion often resulted in loss of biodiversity and greenhouse gas emissions from land conversion and livestock.
Land Use Changes
Land use changes have accelerated during the Holocene, particularly with urbanization and industrialization. Moving away from natural ecosystems, modern land development has radically transformed landscapes. This alteration contributes to changing local climates and affecting ecological systems.
The principal advantage of land use changes is improved food production, but these practices can lead to negative outcomes. Habitat destruction, increased carbon emissions, and enhanced vulnerability to climate-related disasters are significant concerns.
Industrial Revolution Effects
The Industrial Revolution, commencing in the 18th century, marked a pivotal point in human environmental interaction. It introduced mass production and extensive fossil fuel usage, significantly increasing greenhouse gas emissions.
A key characteristic of the Industrial Revolution is its transformative impact on economies and lifestyles. While it spurred technological advances, the consequences for Earth's climate are alarming. The rapid increase in carbon dioxide and methane levels is driving global warming at an unprecedented rate.
In summary, understanding the Holocene Epoch reveals how temperature trends and human influences have shaped the planet's climate. Recognizing these patterns is crucial to address the challenges posed by contemporary climate change.
Comparative Analysis of Historical Temperature Fluctuations
The exploration of historical temperature fluctuations entails a crucial part of understanding the climate dynamics of Earth. This section examines various factors that contribute to temperature changes over geological time scales. By analyzing both natural and anthropogenic influences, we can appreciate the complex interplay of elements that have shaped our planetβs climate. This comparative analysis also informs current climate policies and future research trajectories.
Natural Climate Variability
Volcanic Activity
Volcanic activity plays a significant role in climate variations by releasing large amounts of ash and gases, particularly sulfur dioxide, into the atmosphere. These emissions can lead to short-term cooling effects following a major eruption. Notably, the 1991 eruption of Mount Pinatubo caused a global temperature drop of about 0.5 degrees Celsius for a couple of years. The key characteristic of volcanic activity is its ability to influence climate rapidly, making it a vital point of focus in this article. The uniqueness of volcanic emissions lies in their temporary yet dramatic impact on atmospheric conditions. However, the disadvantage is that these fluctuations can be misinterpreted as natural climate change, obscuring long-term trends induced by human activity.
Solar Radiation Changes
Changes in solar radiation are another primary natural influence on Earthβs temperature. Solar cycles, which last about 11 years, can affect the amount of solar energy reaching the Earthβs surface. The key characteristic of these cycles is their periodic nature, making them reliable indicators for certain climatic trends. In this article, understanding solar radiation changes helps clarify whether historical temperature patterns align with solar activity or other climatic factors. The unique feature of solar variation is its long-term consistency in modulating Earthβs climate. A downside, however, is that this factor is often overshadowed by human-induced changes, leading to undervaluation of its role in recent climate shifts.
Ocean Currents Influence
Ocean currents serve as a mechanism for redistributing heat across the planet, profoundly impacting global climate patterns. The key characteristic of ocean currents is their capacity to transport warm water from the tropics to the poles, influencing temperatures in coastal regions and beyond. This article benefits from including ocean currents as they illustrate the interconnectedness of different climate components. The unique aspect is that short- and long-term modifications in currents can lead to significant climate variances, such as during El NiΓ±o and La NiΓ±a events. Nonetheless, their complexity can be both an advantage and a disadvantage in analysis, as untangling their effects from cases of natural variability can be challenging.
Anthropogenic Climate Change
Greenhouse Gas Emissions
The rise of greenhouse gas emissions presents a critical challenge in understanding recent temperature increases. Human activities, especially fossil fuel combustion, have led to unprecedented levels of carbon dioxide and methane in the atmosphere. The key characteristic of these emissions is their long-lasting influence on Earth's climate system. This article highlights greenhouse gas emissions because they directly correlate with observed global warming. The unique feature is the rapid increase of these gases compared to natural occurrences, causing alarm among scientists. A significant disadvantage is that while they are the primary drivers of current changes, measuring their impact accurately demands comprehensive models and long-term datasets.
Urban Heat Islands
Urban heat islands are localized areas that experience significantly warmer temperatures than their rural surroundings due to human activities, particularly in cities. The key characteristic of urban heat islands is their ability to amplify temperature increases in urban settings. They are a vital aspect for this article as they illustrate the effects of land-use changes on local climates. The uniqueness of urban heat islands lies in their prevalence in densely populated areas, leading to both health risks and energy usage implications. However, identifying their full contribution to broader climatic changes is complex, marking a disadvantage in analyses focused on global temperature trends.
Deforestation Impact
Deforestation dramatically impacts climate by altering local and global temperature patterns. Trees play a vital role in sequestering carbon dioxide, and their removal directly increases atmospheric CO2 levels. The key characteristic of deforestation is its ability to change land surface properties, impacting albedo and local temperatures. This article discusses deforestation impact, providing insights into its long-term ecological consequences. The unique feature of deforestation is its immediate effects on ecosystems and climate feedbacks. However, its impacts are sometimes difficult to isolate from other human factors, which can complicate analysis.
"Understanding the interplay of natural variability and anthropogenic factors is essential for grasping the complexities of historical temperature changes."
Exploring these comparisons equips researchers and policymakers to make informed decisions about environmental management and climate adaptation strategies.
Modeling Temperature Changes
Modeling temperature changes is essential in understanding Earth's climate history and predicting future trends. This section focuses on various climate models and their implications for comprehending historical temperature patterns. Models help scientists simulate climate scenarios, evaluate natural climate variability, and assess human impacts on the environment. They provide insights into how different variables interact within the climate system, thus contributing to both academic research and policy-making.
Climate Models Overview
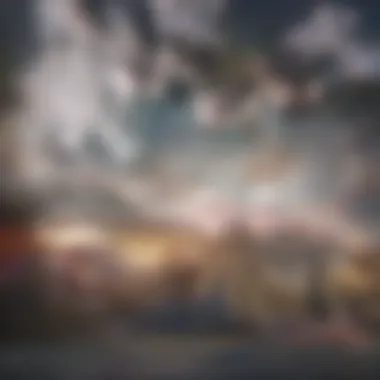
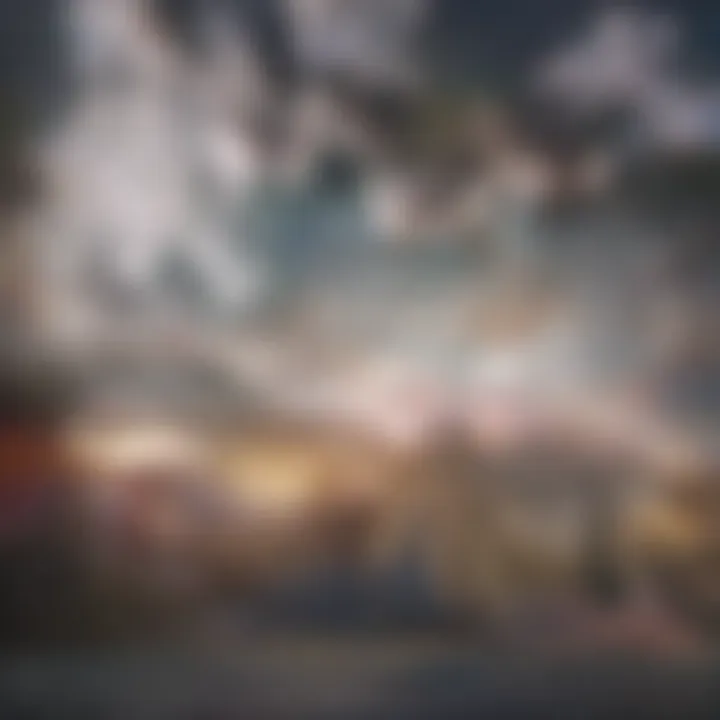
Climate models are critical instruments in studying temperature fluctuations. They classify into two main categories: global climate models and regional climate models. Each model serves a specific purpose and offers unique insights into climate behaviors.
Global Climate Models
Global Climate Models (GCMs) simulate climate systems on a planetary scale. Their primary characteristic lies in their comprehensive representation of the Earth's atmosphere, oceans, and land surfaces. GCMs are favorable due to their ability to integrate vast amounts of data across different regions and time scales. This feature allows for a more holistic understanding of climatic conditions.
One unique aspect of GCMs is their grid-based structure, where the Earth's surface is divided into a three-dimensional grid. This structure enables the model to perform calculations on multiple variables, like temperature and precipitation, simultaneously. However, they may have limitations in localizing specific weather patterns and events due to their broader scope, which can obscure detailed regional impacts.
Regional Climate Models
Regional Climate Models (RCMs) are designed to focus on specific geographical areas. Their key characteristic is their high resolution and sensitivity to local features such as topography and land use. This aspect makes them particularly beneficial for studying localized climate phenomena. A significant advantage of RCMs lies in their ability to provide finer projections for smaller regions, which can inform local decision-making.
Despite their advantages, RCMs may rely heavily on GCM outputs for boundary conditions, introducing potential uncertainties. Their results can differ significantly from GCMs when considering broader trends, thus requiring careful interpretation when applied to larger contexts.
Uncertainty in Projections
Uncertainty in projections is a fundamental aspect of climate modeling. It reflects the inherent variability in climate systems and the limitations of current models. A notable characteristic of this uncertainty is its connection to model parameters, initial conditions, and future greenhouse gas emissions scenarios. This makes it a topic of great importance for climate research.
Understanding uncertainty helps researchers communicate risks and challenges associated with specific climate predictions. It serves as a vital reminder of the complexities involved in modeling Earthβs climate behaviors. While uncertainty can hinder precise predictions, it also guides future research by identifying which factors need more attention or data.
Validation of Historical Data in Models
The validation of historical data in models is crucial for establishing confidence in climate projections. By comparing modeled temperatures with observed data, researchers can refine their models and enhance their accuracy. This process involves rigorous testing against proxy records and instrumental data, which allows scientists to evaluate how well models reproduce past climate conditions. Maintaining accuracy is a priority in climate studies, especially as society faces climate-related challenges.
Temperature Anomalies and Their Significance
Understanding temperature anomalies is crucial in the context of climate science. These anomalies refer to deviations from the average temperature within a given period, providing insights into climatic trends and shifts. Monitoring these fluctuations is essential since they help scientists analyze whether changes are part of natural variability or a consequence of human activities. By examining temperature anomalies, we can better understand potential risks and impacts related to climate change.
Recent Temperature Anomalies
In recent decades, temperature anomalies have showcased a worrying trend. Studies indicate that global temperatures have risen significantly, with anomalies becoming increasingly pronounced. For instance, the last two decades have seen record-breaking average temperatures each year. This increase is largely attributed to anthropogenic factors, particularly greenhouse gas emissions from industrial activity, transportation, and deforestation.
Data from NASA and NOAA highlight the growing patterns of anomalies across different regions. Areas such as the Arctic are experiencing stark rises in temperatures, with some regions warming nearly four times faster than the global average. This poses risks not just locally, but also globally, as changes in polar temperatures affect ocean currents and weather patterns.
Significance for Future Studies
Implications for Climate Policy
The rising temperature anomalies are critical for shaping climate policy. Policymakers need to take into account how these shifts impact ecosystems, economies, and public health. One of the key characteristics of this implication is the urgency for action. Policymakers can utilize data on temperature anomalies to advocate for stronger regulations on emissions and promote sustainable practices. Furthermore, by addressing the latest climate data, policies can be more adaptive to the rapid changes observed.
The unique feature of this implication lies in its evidence-based approach. It is beneficial as it grounds policy measures in scientific reality, allowing policymakers to make informed decisions that could mitigate adverse climatic effects. However, some challenges arise in aligning political agendas with the scientific data, which can delay crucial changes needed in policy.
Adaptation Strategies
Adaptation strategies are essential to cope with the implications of rising temperature anomalies. These strategies enable communities and ecosystems to adjust to changing temperatures and their impacts. A significant characteristic of these strategies is their focus on resilience. By building infrastructure that can withstand extreme weather, such as flood-resistant buildings, societies can protect themselves against the adverse effects of climate change.
One unique aspect of adaptation strategies is their locality. Tailoring strategies to fit specific regional needs enhances their effectiveness. For example, coastal cities might focus on seawall construction while inland regions may prioritize drought-resistant agriculture. However, resources can be a limitation. Without sufficient funding and planning, the effectiveness of these strategies may diminish, underscoring the importance of collaborative efforts.
Research Directions
Exploring research directions surrounding temperature anomalies offers a roadmap for future climate studies. It highlights the need for continuous data collection and analysis to understand climate dynamics better. The ongoing investigation into feedback loops, such as those between temperature increases and glacier melting, is particularly important.
A key characteristic of this aspect is its interdisciplinary nature. Incorporating knowledge from climatology, biology, and social sciences provides a holistic view of the climate crisis. Unique features of this direction include collaborative research initiatives that bring together scientists from various fields to address complex climate issues. However, multidisciplinary approaches can also pose challenges in communication and methodology standardization. Nonetheless, the potential benefits of a united effort make it a promising avenue for further examination.
Temperature anomalies are not just numbers; they signify real-world impacts on our environment, society, and future.
End
The conclusion of this article emphasizes the significance of understanding the historical changes in Earth's temperature. Historical temperature records provide valuable insights into how natural processes and human activities have shaped our climates over centuries. By exploring these influences, we can better understand today's climate challenges.
Key Takeaways
- Understanding Fluctuations: Historical data reveals how Earth's climate has fluctuated through various geological periods. This knowledge is essential for recognizing patterns that can be important in predicting future shifts.
- Impacts of Human Activity: A profound takeaway is the impact of human actions on climate. Human-induced factors such as greenhouse gas emissions and land-use changes have accelerated temperature changes, further highlighting the urgency for climate action.
- Climate Models: The integration of historical temperature data into climate models enhances their accuracy. These models are pivotal in forecasting future climate scenarios and preparing society for impending changes.
- Climate Policy Implications: Understanding past climate events can guide policymakers in developing effective strategies aimed at mitigating climate change. Historical context is critical to enhancing adaptive strategies and resilience measures.
Future Research Imperatives
Future research must focus on several imperatives to deepen our understanding of historical temperature trends:
- Expansion of Data: Researchers should strive to expand the range of available temperature data. This includes improving the collection of proxy data from remote areas, such as ice cores and sediment layers.
- Interdisciplinary Approaches: It is crucial to adopt interdisciplinary perspectives that combine geology, ecology, and atmospheric sciences. This holistic approach will allow for a more comprehensive understanding of climate systems and their interrelated components.
- Technological Advancements: Continued advancement in technology can aid in refining climate models. Leveraging machine learning and artificial intelligence can improve predictive capabilities.
- Public Awareness and Education: Raising awareness about historical climate changes deserves focus. Educating the public on these themes is vital to foster an informed community that can engage in climate action.
In summary, the conclusion underscores the complex interplay of historical temperature changes with today's climate realities. Grasping this connection is imperative for ensuring a more sustainable future.