Harnessing Quantum Computing for Future Innovations

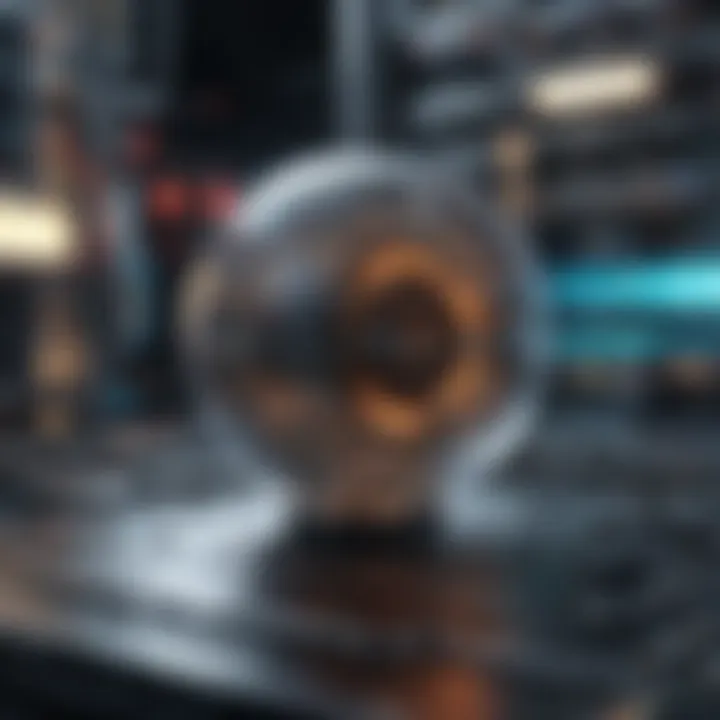
Intro
Quantum computing, a frontier in tech and science, stands poised to revolutionize many sectors. At its core, it harnesses the principles of quantum mechanics to process information in a way that classical computers can’t. With vast potential in fields ranging from cryptography to material science, understanding the basics and implications of quantum technology can reshape our world.
This article delves into the groundbreaking capabilities of quantum computers. It promises to break down the mountain of complexities and jargon surrounding the topic, making it accessible to a diverse audience. With quantum computing still in its infancy, the window of opportunity is wide open for those eager to explore its potential.
Key Research Findings
Overview of Recent Discoveries
In the last few years, research into quantum computing has surged. Think of it like a race with quantum representatives burning rubber towards the finish line. You have institutions like IBM and Google revealing their quantum processors, while startups like Rigetti and IonQ are making inroads with novel approaches. The discovery of quantum supremacy in 2019 marked a pivotal moment. Google’s Sycamore processor completed a calculation in 200 seconds that would have taken the world’s fastest supercomputer approximately 10,000 years to solve. This raised more than just eyebrows in the scientific community.
Significance of Findings in the Field
These discoveries aren't just small reverberations—they're seismic shifts. The implications reach deep into various domains:
- Cryptography: Quantum computers could crack conventional encryption methods, pushing the need for post-quantum cryptography to the forefront.
- Healthcare: Quantum simulation can expedite drug discovery, accurately modeling molecular interactions.
- Artificial Intelligence: Advanced algorithms fueled by quantum speed can process vast data sets in seconds, reshaping machine learning.
"The advent of quantum computing may mark the dawn of a new era in computational capability, offering solutions to problems previously deemed insurmountable."
Breakdown of Complex Concepts
Simplification of Advanced Theories
No one likes being lost in a maze of technical jargon. That’s why breaking down these advanced theories makes sense.
- Qubits: These are the building blocks of quantum computing. Unlike regular bits, which can be 0 or 1, qubits can be both, thanks to superposition. Imagine flipping a coin—while it’s spinning, it can be viewed as both heads and tails.
- Entanglement: This spooky action at a distance, as Einstein called it, allows qubits to be intricately connected. Changes to one qubit can instantaneously affect another, no matter the distance.
Visual Aids and Infographics
To truly grasp these concepts, visualizing them becomes essential. A simplified infographic might help illustrate how a basic quantum algorithm functions, showing transitions between states and the flow of logic that influences outcomes. Since we are harnessing such novel ideas, tools like Lucidchart or Visme can be instrumental in creating such visuals.
By taking a closer look at these developments and theories, one begins to understand the massive waves quantum computing promises to send through our technological landscape. Distilling these complex ideas into digestible pieces not only aids comprehension but sparks curiosity—a necessary trait for anyone in academia or industry.
Foundations of Quantum Computing
Understanding the foundations of quantum computing is like unlocking a treasure chest of potential innovation and change. These foundations not only set the stage for all the astonishing capabilities of quantum mechanics but also distinguish it sharply from classical computing. A grasp of quantum principles helps clarify the unique advantages that this nascent technology can offer, such as breakthroughs in processing power and efficiency.
Basic Principles of Quantum Mechanics
At the crux of quantum computing lies quantum mechanics, which governs the behavior of matter and energy on a minute scale. Unlike classical physics, where objects exist in definite states, quantum mechanics reveals a world of probabilities. A fundamental concept here is superposition, where quantum bits, or qubits, can exist in multiple states simultaneously—working like a spinning coin rather than a simple heads or tails. Additionally, the principle of entanglement refers to the connection between qubits, where the state of one can instantaneously affect the state of another, no matter the distance between them. This defies our traditional understanding of locality and leads to a new realm of possibilities for data processing and transmission.
Moreover, it’s essential to consider quantum interference, another principle that enhances computational capacities. By manipulating the probabilities of qubit states, computation can be accelerated, leading to faster and more efficient outcomes.
Quantum Bits: The Building Blocks
When diving into quantum computing, qubits are the basic building blocks one must understand. Unlike classical bits, which can be either a 0 or a 1, qubits can embody a 0, 1, or both simultaneously due to superposition. This characteristic allows quantum computers to process an avalanche of data concurrently, making them exponentially more powerful for certain tasks.
Qubits are realized through various physical systems, ranging from photons to trapped ions. Each implementation comes with its perks and challenges, such as coherence time and error rates. Superconducting qubits used in platforms like IBM's quantum processors are particularly notable for their efficiency and scalability. On the flip side, achieving robust qubit interaction requires overcoming significant hurdles like decoherence—a problem that we’ll explore detail later.
Quantum Gates and Circuits
Quantum gates are the functional units that enable quantum programming. Much like classical logic gates, they manipulate qubits to perform operations. A key aspect of these gates is how they can create complex superpositions or entangled states, which deliver processing power that conventional computers can't even approach.
The arrangement of these gates forms a quantum circuit, which serves as the backbone of quantum algorithms. Each gate alters the state of qubits in a precise manner, and the optimal arrangement can lead to extraordinary results. The gates can perform simple operations such as the Hadamard gate, which places qubits into superposition, or the CNOT gate, which is critical for creating entangled states.
Notably, the design and optimization of quantum circuits are crucial areas of ongoing research. The efficiency of a quantum algorithm can hinge on how well these circuits are organized, akin to tuning an intricate machine for peak performance.
"Quantum computing represents a fundamental shift in how we approach computation and problem-solving. It challenges our understanding of what is possible in the realm of technology."
To summarize, the foundations of quantum computing reveal a fascinating world where basic principles diverge significantly from classical norms. As we progress deeper into this quantum realm, the implications become not just technical but transformative, influencing various sectors from cryptography to complex system simulations.
Comparative Analysis: Quantum vs Classical Computing
In the realm of computing, understanding the distinctions between quantum and classical systems is paramount. This comparative analysis is not merely a technical examination; it serves to illuminate how quantum computing stands to redefine standards in speed, efficiency, and problem-solving capabilities. The relevance of this analysis stems from the ongoing discourse in both academic and practical domains about where computational mechanics are headed.
Speed and Efficiency
When we talk about speed in computing, we usually mean how quickly a computer can solve a problem. Classical computers operate using bits, which can be either a zero or a one. This binary system limits the computational paths available. On the contrapoint, quantum computers leverage quantum bits, or qubits, which embody the principle of superposition. A qubit can exist in multiple states at once, allowing quantum computers to carry out numerous calculations simultaneously.
This property leads to exponential increases in computational power under certain conditions. For instance, Shor's algorithm, which is designed for factoring large integers, runs significantly faster on quantum systems compared to any known classical algorithm. The potential applications are wide-ranging: from efficiently solving complex optimization problems to decoding cryptographic encryptions that classical machines would find insurmountable.
- Multiple pathways: Unlike classical computers, which navigate a single pathway, quantum systems can explore many pathways at once.
- Problem-solving in record time: Some algorithms, such as Grover's search, show quadratic improvements in speed, offering promising implications for databases and search engines.
Resource Requirements
Resource implications are critical when comparing quantum and classical computers. Classical computers need physical components like transistors, which have been miniaturized over decades to vastly increase their performance. Quantum computers, however, rely on entirely different resources that are often harder to maintain. To operate qubits in a stable state, systems usually require cryogenic temperatures, sometimes close to absolute zero.
This presents additional challenges:
- High operational costs: The cooling systems and isolation from environmental noise necessitate significant investments in infrastructure.
- Limited availability of qubits: As of now, fewer qubits are practically achievable. Leading quantum machines, such as those developed by IBM and Google, have hundreds of qubits, but scaling this to thousands or millions for full efficiency remains elusive.
Despite these hurdles, the development of quantum technology is relentless. Researchers are not just looking at traditional methods but are exploring topological qubits and other novel approaches that could lead to advances in both efficiency and resource management.
The journey toward integrating quantum computing into mainstream applications is punctuated with hurdles but also ripe with possibilities that could extend far beyond current understanding.
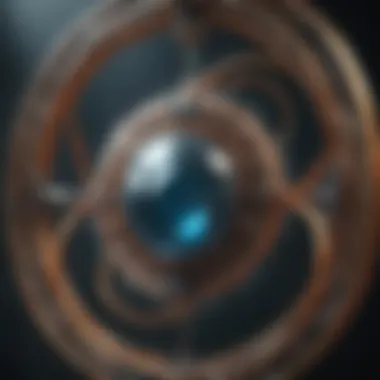
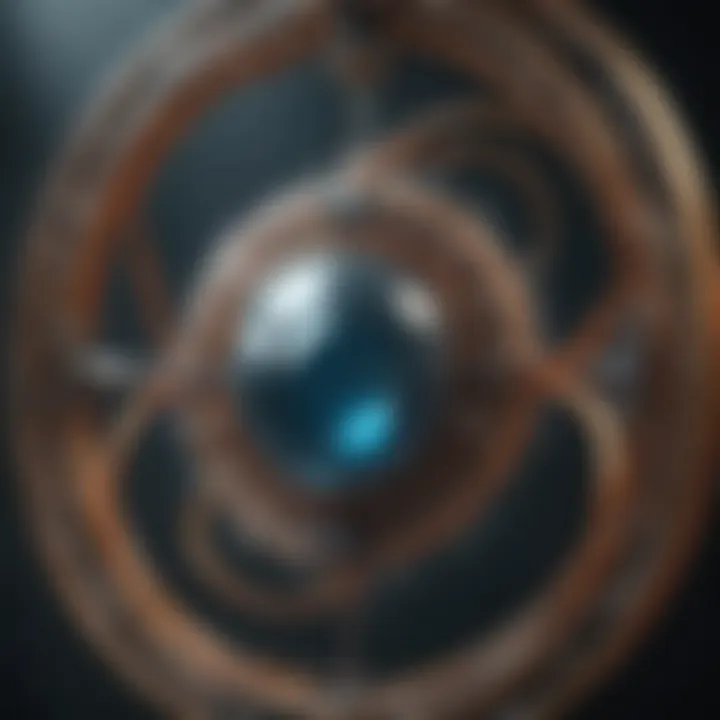
Understanding these key differences between quantum and classical computing isn't just an academic exercise; it's about anticipating a future where quantum systems may lead the way in fields ranging from medicine to finance. With advancements underway, there's a shared interest in the challenge of harnessing this incredible power responsibly and effectively.
Applications in Cryptography
In today’s world, where data breaches and cyber threats seem to lurk around every corner, cryptography stands as the first line of defense. The traditional systems have served their purpose, but with the rise of quantum computing, a revolution is brewing in the field of securing information. Quantum computing’s unique capabilities could reshape the landscape of cryptographic methods entirely, offering both opportunities and challenges. It’s not just about faster calculations; it’s about the potential to redefine the very foundations of security protocols.
Quantum Key Distribution
One of the most exciting applications of quantum computing in cryptography is Quantum Key Distribution (QKD). QKD leverages the principles of quantum mechanics to create secure communication channels. Unlike classical methods, QKD enables two parties to generate a shared, secret random key, which is secure against any potential eavesdroppers.
The beauty of QKD lies in its fundamental principle: it uses quantum bits that act differently when observed. If a third party tries to intercept the communication, the quantum state changes, alerting the original parties of a breach. This isn’t mere theory; it’s been tested and employed in various projects around the world.
Several techniques underpin QKD, including:
- BB84 Protocol: The first and most widely recognized QKD protocol, developed by Charles Bennett and Gilles Brassard in 1984.
- E91 Protocol: Based on the concept of entanglement, proposed by Artur Ekert in 1991.
The real-world implications of QKD are profound. In sectors like finance, healthcare, and defense, ensuring data integrity and privacy could be revolutionized. However, while quantum key distribution promises immense potential, it also paves the way for some logistical challenges that need to be addressed.
Challenges to Classical Encryption
As we stand on the brink of this quantum age, it’s vital to examine how quantum computing poses a threat to classical encryption methods. Current encryption systems, such as RSA and ECC, rely on mathematical problems that are computationally intensive for classical computers. Yet, quantum computers can tackle these problems using algorithms like Shor’s, rendering traditional encryption techniques vulnerable.
Some key challenges include:
- Factorization and Integer Decomposition: Shor's algorithm can efficiently factor large integers, endangering the RSA encryption that underpins much of today’s secure communications.
- Discrete Logarithms: Similarly, the discrete logarithm problem, fundamental to ECC, succumbs to the capabilities of quantum algorithms.
- Data Harvesting and Future Decryption: Encouragingly, many organizations often store encrypted data for future analysis. If such data remains intact, theoretically, with the right quantum tools down the line, it could be decrypted even if it’s been stored for years.
In response to these vulnerabilities, the field of post-quantum cryptography (PQC) is emerging. PQC aims to develop new algorithms that remain secure against potential quantum attacks. This area represents an urgent front for researchers and developers alike, as the stakes have never been higher for data privacy.
"The only thing that’s certain about the future is uncertainty itself."
— This sentiment underscores the pressing need for robust security strategies in an unpredictable digital landscape.
Quantum Computing in Drug Discovery
Quantum computing stands to revolutionize the field of drug discovery, streamlining processes that have traditionally been both complex and time-consuming. This technology holds the potential to analyze vast datasets at unprecedented speeds, factors that are essential given the growing demand for new pharmaceuticals. A properly conducted drug discovery journey can span over a decade and involve significant financial investment; thus, harnessing quantum capabilities can substantially reduce timelines and costs.
Molecular Simulation Techniques
At the heart of drug discovery lies the essential phase of molecular simulation. This involves modeling molecular behaviors and interactions, which can illuminate pathways for developing effective compounds. Classical computers struggle with this task due to inherent limitations in resource allocation and processing speed, especially when simulating larger protein structures or diverse chemical environments.
Quantum computers, however, can effortlessly simulate intricate quantum interactions, enabling researchers to envisage how new drugs will behave within biological systems. Consider the following advantages:
- Accurate Models: Quantum systems can capture the nuances of molecular interactions far better than classical systems.
- Predictive Potential: Researchers can predict how a drug behaves in various environments before physically synthesizing it, reducing the trial-and-error phase.
- Speed: Tasks that would take classical models weeks or months to compute can often be reduced to hours, or even minutes, using quantum algorithms.
The ability to accurately predict molecular properties and behaviors accelerates the drug development process dramatically. Moreover, breakthroughs in algorithms developed for quantum simulations can lead to discovering entirely new therapeutic targets, which could change the landscape of medical treatments.
Accelerating Compounds Investigation
As the pharmaceutical industry faces a critical need for innovation, accelerating compounds investigation becomes key. This is all about identifying potential drug candidates from a myriad of possibilities. Traditional methods often rely on high-throughput screening where thousands of compounds are tested, consuming both time and resources. Quantum computing offers a more strategic approach to this process.
With quantum-enhanced optimization techniques, researchers can efficiently narrow down potential molecules much faster than before. Here are a few noteworthy points to consider:
- Enhanced Screening: By leveraging quantum entanglement, scientists can analyze multiple compounds simultaneously, vastly increasing the efficiency of the screening process.
- Informed Decisions: Each molecular model can provide insight into numerous compound properties at the same time, allowing for informed decision-making when selecting candidates for further testing.
- Cost Efficiency: Reducing the time required for the investigational process subsequently decreases costs associated with research and development, making the process not only faster but also economically viable.
"The ability to simulate and analyze drug compounds simultaneously with quantum technology might be the key to unlocking new treatment options faster than ever before."
Optimization Problems and Quantum Computing
Optimization problems are at the heart of countless decision-making processes, impacting fields as diverse as logistics, finance, and engineering. The challenge of finding efficient solutions to complex problems has occupied researchers and practitioners for decades, leading to significant costs and inefficiencies when using classical methods. Quantum computing emerges as a formidable contender to tackle these challenges, offering the potential for exponential speed-ups and enhanced performance on specific tasks. Understanding the intersection of optimization problems and quantum computing is crucial for unlocking future advancements that can reshape industries and academic disciplines alike.
Classical Challenges in Optimization
Classical optimization techniques often face hurdles that can prevent them from finding optimal solutions in a reasonable timeframe. Some of the most pressing challenges include:
- Dimensionality: As the number of variables increases, the solution space expands exponentially. This phenomenon, known as the "curse of dimensionality," can make even sophisticated techniques like gradient descent slow and inefficient.
- Local Minima: Many classical algorithms can easily get stuck in local minima. This entrapment can lead to suboptimal solutions that do not reflect the true optimal values.
- Constraint Handling: Real-world problems frequently contain constraints that must be respected, complicating the optimization process. Classical methods may struggle to efficiently navigate these constraints, leading to less satisfactory solutions.
- Computational Cost: Finding the best solutions often requires significant computational resources, especially for large-scale problems. This necessity can lead to prohibitive costs in terms of both time and money.
These issues pose significant barriers to achieving optimality in decision-making processes, and the reliance on classical techniques limits the potential for breakthroughs in various domains.
Potential Quantum Solutions
Quantum computing offers innovative approaches to address classical optimization challenges through unique principles of quantum mechanics. Some potential solutions and their implications include:
- Quantum Annealing: This technique leverages quantum bits, or qubits, to simulate the process of finding the global minimum of a cost function. Quantum annealers, like those developed by D-Wave, provide a platform that can explore vast solution spaces more efficiently than classical approaches.
- Grover's Algorithm: Grover’s algorithm presents a paradigm shift in searching through unstructured data. It allows quantum computers to search for optimal solutions with quadratic speedup over classical algorithms, significantly reducing the number of queries needed to find satisfactory outcomes.
- Variational Quantum Eigensolver (VQE): VQE is deployed specifically to tackle complex optimization problems, particularly in quantum chemistry. By combining classical and quantum techniques, the method iteratively adjusts parameters to minimize the energy of quantum states, paving the way for breakthroughs in material science and drug discovery.
- Hybrid Approaches: The fusion of classical and quantum methods may provide optimal outcomes for complex problems. By utilizing quantum computing for the most challenging parts of optimization problems, classical methods can manage simpler constraints, creating a synergistic approach.
The potential for quantum computing to radically change optimization problem-solving is immense. By navigating vast solution spaces, bypassing local minima, and handling complex constraints more effectively, quantum computers could ultimately transform how society approaches and resolves critical issues.
Quantum Simulations of Physical Systems
Quantum simulations present a unique avenue to explore and understand physical systems in ways that classic computing struggles with. These simulations utilize the principles of quantum mechanics to model complex interactions, providing deeper insights into behaviors that classical models simply cannot replicate. As the field of quantum computing continues to mature, so too does our ability to accurately simulate intricate systems reliably.
One of the pivotal benefits of using quantum simulations is their ability to handle entangled states and superpositions effectively. Unlike traditional computers, where data is processed in binary, quantum computers can manage vast possibilities simultaneously. This makes them especially relevant for tasks where accuracy is paramount, such as understanding quantum materials.
Additionally, quantum simulations can significantly reduce the computational resources needed to predict behaviors in systems with massive degrees of freedom. This efficiency could lead to breakthroughs in materials science, climate modeling, and more.
"The applications of quantum simulation can lead to advancements we currently only dream about; it's a game changer for material discovery and even tackling climate change."
Modeling Quantum Materials
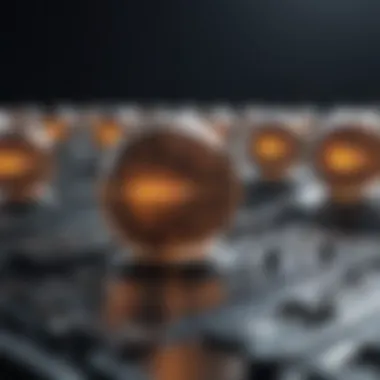
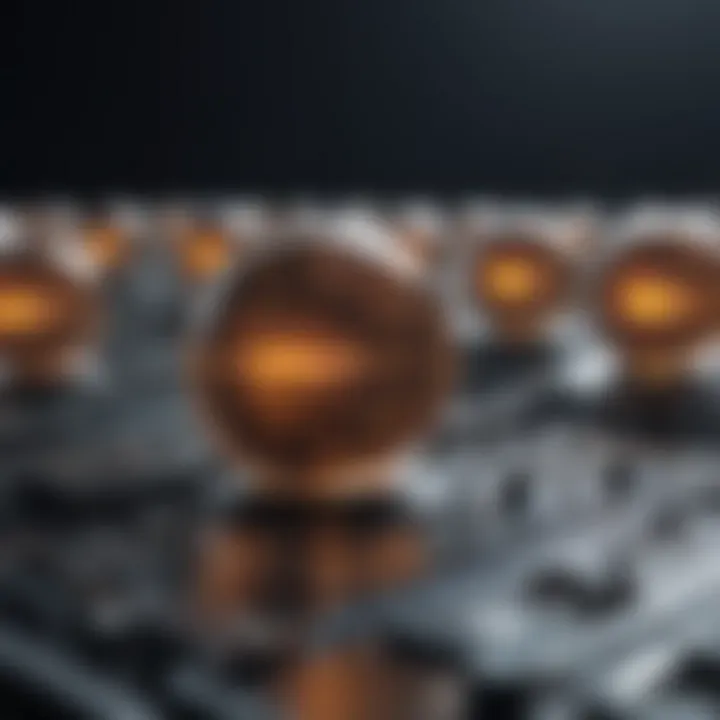
Modeling quantum materials through quantum simulations allows scientists to predict properties that are otherwise challenging to determine. Materials like superconductors and magnetic substances exhibit complex behaviors governed by quantum mechanics. Traditional methods often rely on approximations which might miss critical details. By directly simulating the quantum state of materials, researchers can gain insights into their behavior at atomic and subatomic levels.
A few key aspects of this modeling approach include:
- Accuracy: Quantum simulations can account for interactions between particles much more effectively than classical simulations. This results in a better understanding of fundamental properties.
- Design of New Materials: By predicting how materials will behave, scientists can tailor their designs for specific applications, such as creating more efficient solar panels or innovative batteries.
- Speed of Discovery: The accelerated pace at which we can explore material properties could lead to faster discovery cycles for new technologies.
Applications in Climate Science
The potential use of quantum simulations extends to vital applications in climate science. Understanding complex systems like our planet’s climate requires vast amounts of data and intricate modeling to predict changes accurately. Quantum computing can help simulate climate models with a higher degree of fidelity.
Key applications include:
- Modeling Atmospheric Interactions: Quantum simulations can better capture the nuances of how different atmospheric elements interact, yielding more accurate weather forecasts and climate change models.
- Carbon Capture Mechanisms: Exploring materials capable of filtering greenhouse gases can be accelerated using quantum simulations, pushing us toward viable carbon capture solutions.
- Ecosystem Dynamics: The ability to model ecosystems dynamically offers a clearer picture of ecological interactions, essential for conservation efforts and sustainability planning.
As quantum technology progresses, its role in advancing our understanding of physical systems becomes increasingly crucial. The ability to simulate and model quantum materials and complex climate systems will not just enhance scientific knowledge; it can also inform policy decisions and guide technological innovations.
Artificial Intelligence and Machine Learning
Artificial intelligence (AI) and machine learning (ML) are playing pivotal roles in the evolution of quantum computing. As we stand at the crossroads of two of the most revolutionary technologies of our time, understanding their intersection is vital for grasping how they can reshape various sectors. The unique abilities of quantum systems could enhance machine learning algorithms, yielding faster processing speeds and the capability to work with more complex datasets. This possibility emphasizes the importance of these technologies in fields like finance, healthcare, and beyond, where making informed decisions swiftly can be the key to success.
Enhancing Machine Learning Algorithms
Quantum computing offers a suite of advantages that can significantly improve machine learning algorithms. Traditional algorithms often struggle with large datasets due to limitations in processing power and speed. In contrast, quantum algorithms, such as the Quantum Support Vector Machine, can handle these tasks more efficiently. By utilizing quantum superposition and entanglement, these algorithms can explore multiple solutions simultaneously, arriving at optimized outcomes much faster than their classical counterparts.
Here are some critical points about how quantum computing enhances machine learning:
- Speed: Quantum algorithms can theoretically evaluate multiple possibilities at once, drastically reducing the time required for training machine learning models.
- Complex Problem Solving: Quantum systems can navigate convoluted data landscapes more effectively, presenting solutions that classical systems might overlook.
- Improved Accuracy: By leveraging quantum mechanics, there’s potential for greater precision in predicting outcomes based on complex datasets.
This intersection of quantum computing and machine learning creates a fertile ground for innovation. As research continues, it is likely that we will see substantial breakthroughs that transform how machines learn from data.
Challenges and Limitations
While the potential gains are promising, the application of quantum computing to machine learning is not without its challenges. The technology is still in nascent stages, and several obstacles must be addressed before it can be fully realized:
- Decoherence: Quantum bits, or qubits, are delicate and can lose their quantum state due to environmental factors. This instability poses a challenge to maintaining coherent computations needed for machine learning applications.
- Scalability: Current quantum systems contain a limited number of qubits, which restricts the size and complexity of problems they can solve. As researchers work towards building larger, more reliable quantum machines, scalability remains a significant concern.
- Algorithm Development: There’s still much work to be done in developing quantum algorithms suited for specific machine learning tasks. Not all classical algorithms have direct quantum counterparts, which necessitates new approaches and frameworks.
"The integration of quantum computing into machine learning is an exciting journey full of possibilities and hurdles, where ingenuity is tested at every turn."
Quantum Computing and Engineering Challenges
Quantum computing promises to revolutionize various sectors, but it doesn’t come without a bundle of formidable challenges. These engineering hurdles are critical for realizing the full potential of quantum technology. Addressing issues like decoherence, error rates, and scalability is essential not only for the technical advancement of quantum systems but also for gaining trust from industries that could benefit from this cutting-edge technology.
In a world where speed and efficiency in computing matter more than ever, tackling these challenges represents a bittersweet journey towards the quantum age.
Decoherence and Error Rates
Decoherence occurs when a quantum system loses its quantum properties due to interaction with the surrounding environment. This interaction leads to errors in computations, particularly in qubit operations, creating significant limitations in current quantum computing models. Maintaining coherence allows qubits to function correctly, but external disturbances—from temperature variations to electromagnetic radiation—threaten this stability.
- Understanding the Significance of Decoherence:
Decoherence is an inherent challenge because it disrupts the delicate quantum states needed for efficient computing. For instance, consider two qubits in a computational process: if one reads interference from environmental noise, it can lead to a total breakdown of the calculations. As a result, quantum error correction schemes have gained attention, proposing methods to mitigate the effects of decoherence and correct errors. - Error Rates in Quantum Circuits:
Each qubit has a certain probability of making an error during operations. If this rate is too high, the vast speed advantage of quantum computing begins to diminish. Research seeks to reduce qubit error rates below a critical threshold. According to studies, maintaining error rates below one percent is generally deemed necessary for reliable quantum operations.
"The battle against decoherence and error rates is the linchpin in the quest for practical quantum computing."
Scalability Issues
Scalability refers to the ability of quantum computers to effectively increase their operational capacity. As the demand for computational power rises, creating larger and more complex quantum systems without compromising their performance becomes a prime objective. Existing systems face significant hurdles in achieving this scalability.
- Challenges in Increasing Qubit Count:
While researchers have made strides in adding more qubits to quantum systems, the interactions between these qubits can introduce additional error rates. Unlike traditional computers that can simply add hardware, quantum systems require not just more qubits but also effective interconnectivity and operational coherence among them. The challenge lies in ensuring that as more qubits are added, the overall functionality remains intact. - Infrastructure and Cost Considerations:
The infrastructure required for larger quantum systems often presents a financial barrier. Maintaining quantum states necessitates extensive cooling systems, shielding from interference, and more, all of which can inflate costs. In a market where return on investment is critical, determining the trade-off between the quality of quantum computing and its operational cost becomes increasingly important.
In summary, as quantum computing continues to march forward, tackling these engineering challenges becomes not just beneficial but essential. By addressing decoherence, error rates, and scalability, the potential of quantum computing will gradually shift from mere theory to practical application. This path, albeit fraught with obstacles, is where the real innovation lies in harnessing the next generation of computational power.
Quantum Technologies in Telecommunications
Quantum technologies are rapidly carving out their niche in the telecommunications landscape, marking a profound shift in how we conceive data transfer and security. With the relentless surge in data consumption, classic systems struggle to keep up with the ever-increasing demand for bandwidth and security. Quantum telecommunications promises not just faster speeds but also unparalleled security through its foundational principles.
Quantum Repeaters
At the heart of quantum telecommunications, the concept of quantum repeaters emerges as a crucial player in the quest for long-distance quantum communication. Unlike traditional repeaters that amplify signals, quantum repeaters utilize the fundamental properties of quantum mechanics to extend the range of quantum information and mitigate loss of coherence, enabling secure data transfer over longer distances than ever.
These repeaters rely on entanglement swapping—essentially a method to connect distant particles by entangling them through intermediaries. This process allows the quantum state to be preserved over vast distances, creating a bridge in quantum communication that maintains integrity where classical methods falter. The importance of quantum repeaters can't be overstated. They are the backbone of the proposed quantum internet, allowing for high-fidelity transmission of quantum bits, or qubits, which could transform how we share confidential information, ensuring that data remains untouchable by eavesdroppers.
Impact on Data Transfer Security
The implications of quantum technologies extend well beyond speed; they fundamentally redefine the security landscape in telecommunications. With the integration of quantum mechanics into data transfer, we’re witnessing the introduction of quantum key distribution (QKD)—a method where quantum properties are utilized to create secure communication channels.
- Unbreakable Security: QKD guarantees that any unauthorized attempt to intercept the keys will disturb the quantum information, alerting the parties involved of the breach. This feature stands in stark contrast to conventional encryption methods, which, while sophisticated, may still be compromised by advanced algorithms.
- Prevention of Data Manipulation: Through the principles of quantum mechanics, data is inherently tied to its carriers. This means any tampering or interception can be detected immediately. Therefore, users can enjoy a level of trust that was previously unattainable.
"Quantum technologies promise a significant leap in the realm of secure communication, where the very laws of physics act as the safeguard against breaches."
In a world where cyber threats loom large, bridging the gap between classical and quantum systems becomes imperative. As researchers continue to pave the way for these promising technologies, the telecommunications industry stands at a crossroads, poised to embrace a future where data transfer security is not just an aspiration but an assured reality. With quantum repeaters and security mechanisms in place, preparing for this brave new world is not just advisable—it’s essential.
The Role of Quantum Computing in Financial Modeling
In the rapidly evolving landscape of finance, the introduction of quantum computing holds tremendous promise. As traditional computational methods struggle with complex financial models, quantum technology offers a new lens through which to view risk assessment and portfolio management. This section explores how quantum mechanics influence modern finance, enabling improvements in speed and accuracy that were previously unattainable.
Quantum computing is expected to transform financial modeling by harnessing quantum bits, or qubits, which can exist in multiple states at once. This property enables a quantum computer to process enormous datasets and intricate algorithms simultaneously. Financial professionals can leverage this capability to refine their analyses and forecasts, ultimately leading to more informed decision-making.
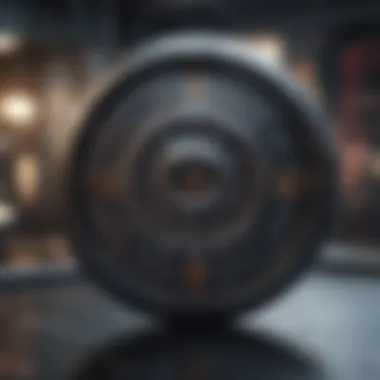
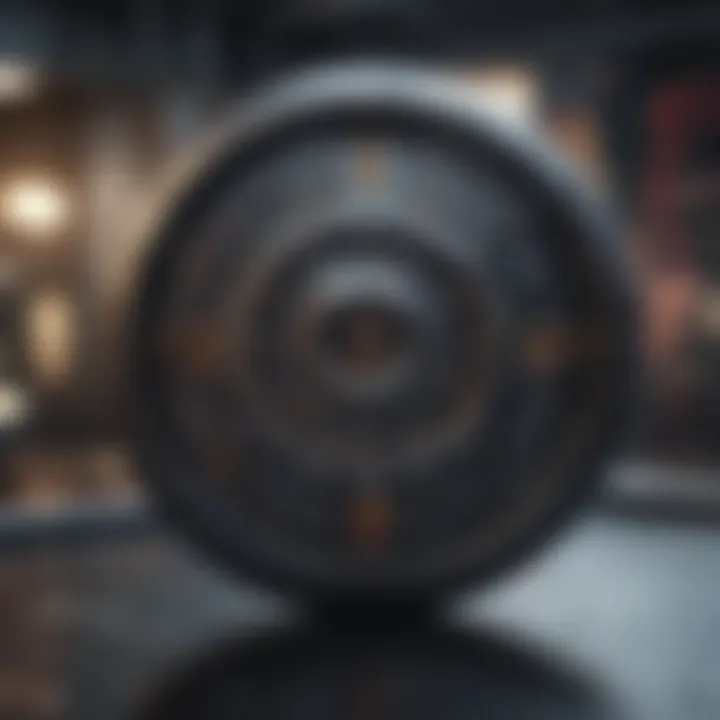
Risk Analysis and Portfolio Optimization
Risk analysis is a critical component in any financial framework. Traditional methods for assessing risk can often be cumbersome or inaccurate, heavily reliant on simplistic models that cannot capture the full complexity of market behavior.
Benefits of Quantum Risk Analysis
- Enhanced Simulations: Quantum computers can run extensive simulations of market conditions in far less time. This speed enables analysts to explore numerous scenarios and evaluate risk with greater granularity than is possible with classical computers.
- Improved Predictions: The ability of quantum computing to analyze vast amounts of data means that the predictions generated are based on more comprehensive datasets. This leads to better anticipation of market fluctuations and potential downturns.
For example, a hedge fund employing quantum algorithms may quickly create optimized portfolios that balance risk and reward profiles more effectively. These optimizations take into account vast datasets that traditional methods would require considerably longer to process.
"Risk management is not just about avoiding loss; it's about maximizing opportunities while knowing the potential pitfalls."
High-Frequency Trading Strategies
High-frequency trading (HFT) has revolutionized stock exchanges. Here, speed matters immensely; the ability to process transactions and analyze trends in real time dictates success. Quantum computing can enhance HFT by executing trades at lightning speed while analyzing multiple factors simultaneously.
The integration of quantum computing into HFT can lead to:
- Rapid Decision-Making: Quantum algorithms can process market data at an unprecedented rate, allowing traders to make informed decisions without delay. This results in performing thousands of trades in mere milliseconds.
- Dynamic Strategy Adaptation: Algorithms can rapidly adjust trading strategies based on constantly shifting market variables. This flexibility enables traders to capitalize on fleeting opportunities that classical algorithms might miss.
Quantum's edge in high-frequency trading does not come without challenges. Developing robust quantum algorithms that ensure reliability and act within the regulatory framework will be crucial. Time will tell if the theoretical speed advantage translates to sustainable market dominance.
Quantum Ethics and Societal Implications
As we cool down the fervor surrounding quantum computing, it is crucial to focus on Quantum Ethics and Societal Implications. This topic doesn’t just hover around the technical marvels of quantum systems; instead, it dives into the moral and societal waters stirred by these advancements. In a world increasingly driven by technology, the ethical implications and societal responsibilities tied to quantum computing are paramount for researchers, developers, and policymakers alike.
Quantum technology has the potential to flip the script across numerous domains—including computing, communication, and even biomedicine. However, with great power comes a hefty set of responsibilities. As quantum computing evolves, so must our understanding of the consequences that stem from its deployment. A thoughtful discourse on ethics can help navigate the nuance of potential benefits against the backdrop of societal risks.
Ethical Considerations in Quantum Developments
When we consider the ethical aspects, it’s not merely a matter of following good practices; it’s about shaping a framework that governs the use of quantum computing responsibly.
- Privacy Concerns: With the power to break classical encryption methods, quantum computing poses significant threats to individual privacy. For example, organizations could misuse quantum advancements to harvest vast amounts of sensitive personal data.
- Equity in Access: The rich potential of quantum technology isn’t evenly distributed. Who gets access to these groundbreaking tools can lead to inequalities that echo through society, creating a digital divide that parallels educational or financial disparities.
- Intellectual Property: The way quantum algorithms are developed raises questions about ownership and patent rights. If a quantum algorithm can outsmart its classical counterpart, does its creator rightfully own the outcome?
These are just a few threads of the ethical tapestry that must be woven into the development of quantum technologies. Ultimately, innovation should not trample over basic human rights and societal well-being.
Regulatory Challenges
Another layer to this ethical quandary is the challenge of regulation in the quantum realm. Many of the rules and regulations that govern technology are often caught in lag mode, struggling to keep pace with rapid advancements.
- Global Standards: In a globally interconnected world, establishing a set of universal regulations on quantum technologies proves complex. Different countries have different ethical frameworks and legal systems. Finding common ground on issues like security or privacy rights is often a matter of heated debates and negotiations.
- Adapting Existing Laws: Current laws surrounding technology often don’t account for quantum-specific ramifications. Just like trying to use a square peg in a round hole, applying conventional rules to quantum computing may not yield effective governance.
- Incentivizing Responsible Use: Regulators face the uphill task of offering incentives for ethical behavior in a fast-moving technological environment. This isn’t as simple as putting a price tag on compliance; it needs a cultural shift within organizations to prioritize ethics alongside profit.
"The responsible development of quantum technology is not just an ethical imperative; it could shape the contours of our society for generations to come."
Navigating the social currents stirred up by quantum computing requires ongoing discussions around ethical standards and regulatory frameworks. By doing so, society can harness quantum’s vast potential while safeguarding fundamental principles of justice, equity, and respect for individual rights.
Future Directions of Quantum Computing
As we continue to probe the transformative landscape of technology, the focus on the future directions of quantum computing remains pivotal. Understanding where this field is heading offers insights into not just technological advancements but also the societal shifts intertwined with these innovations. The development of quantum computing promises a variety of potential benefits including enhanced computational abilities, diverse applications, and implications for regulations and ethics.
Emerging Technologies
The landscape of quantum computing is rife with emerging technologies that stand to redefine not just computation, but entire industries. Several technologies are making waves:
- Quantum Supremacy: This term refers to the point where quantum computers can perform tasks beyond the capability of classical computers. Google’s announcement regarding their quantum supremacy achievement was a significant milestone that brought attention to this frontier.
- Quantum Networking: Developing networks that leverage quantum mechanics could enhance data security and facilitate faster communication. Quantum key distribution systems are just the tip of the iceberg here.
- Topological Quantum Computing: This approach seeks to utilize topological states of matter to perform calculations. It aims to minimize errors compared to current paradigms, thus making computations more reliable.
These technologies pave the way for applications in various fields such as materials science, pharmaceuticals, and climate modeling. However, progress in these areas relies heavily on overcoming certain technical challenges which still linger.
Predictions for the Next Decade
Looking ahead, various predictions about the trajectory of quantum computing provide a framework for understanding possible developments:
- Increased Investment: Companies and governments are pouring resources into quantum research. This surge in investment could lead to breakthroughs in hardware and software, with organizations like IBM and Microsoft leading the charge.
- Widespread Adoption for Specific Applications: Areas like cryptography and optimization problems are likely to see more robust integration of quantum solutions in the next ten years. Industries may gradually adopt quantum techniques as they become more practical and efficient.
- Collaborative Ecosystems: A noteworthy trend might be the formation of collaborative platforms where researchers from various backgrounds come together to accelerate quantum research. This collaborative spirit could ignite innovation at an unprecedented pace.
As the world turns its attention to these upcoming advancements, the synergy between quantum computing and artificial intelligence could yield stark improvements in processing capabilities. This intersection may be another significant focus within the next decade.
"The most profound transformations in technology are often those that reshape our understanding of what is possible."
Understanding these future directions is more than an exercise in prediction; it’s about preparing for a landscape where quantum computing does not merely augment our computational power but fundamentally reshapes how we approach problem-solving across various domains.
Closure: The Path Ahead
The conclusion serves as a critical touchpoint in our exploration of quantum computing. It ties together not just the advancements but also the challenges and future avenues of this captivating field. Understanding where we stand now is important as it sets the stage for what lies ahead. The potential of quantum computing, while still in its infancy, promises to reshape various sectors, ranging from healthcare to finance.
Summary of Key Findings
Throughout the article, we delved into significant findings regarding quantum computing. Here are a few key takeaways:
- Accelerated Computational Speed: Quantum computers can process information at speeds unattainable by classical systems, potentially solving complex problems in seconds that might take traditional computers years.
- Applications Across Domains: From drug discovery to cryptography, the applications are vast and far-reaching. Quantum key distribution can enhance security protocols, while quantum simulations can mimic complex physical systems.
- Unprecedented Efficiency: Quantum algorithms can outperform classical algorithms in specific tasks, particularly in optimization and machine learning.
“The future of quantum computing holds promise yet demands rigorous inquiry and innovative thinking.”
These findings underscore the transformative impact that quantum technology will have, not just in theoretical contexts, but in real-world applications.
The Importance of Continued Research
As we think about the future direction of quantum computing, it is imperative to underscore the importance of ongoing research. Here are some reasons why:
- Addressing Current Limitations: Unlike classical computers, quantum systems face unique challenges like decoherence and error rates. Research is needed to develop better qubits and error correction mechanisms to make these systems stable and reliable.
- Ethical Considerations: With great power comes great responsibility. The impact of quantum computing on fields like cryptography raises ethical questions that require thorough examination to avoid misuse.
- Educational Opportunities: There is a growing need for skilled professionals in quantum computing. Continued research can drive academic programs, fostering the next generation of innovators.
- Interdisciplinary Collaboration: By encouraging collaborations across various disciplines, we can tap into diverse expertise that will propel quantum technology forward.
In summary, without continuous inquiry and funding, the ambitious goals of quantum computing may remain beyond our reach. Staying ahead in this rapidly advancing field requires a commitment to knowledge, innovation, and ethical consideration.