Gravity in Space: Exploring Cosmic Forces and Impacts
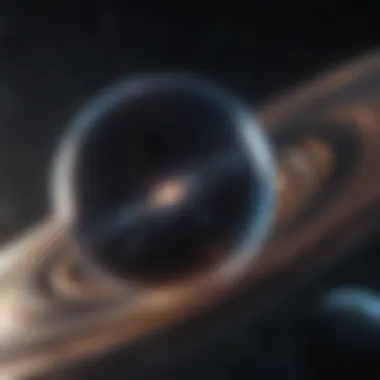
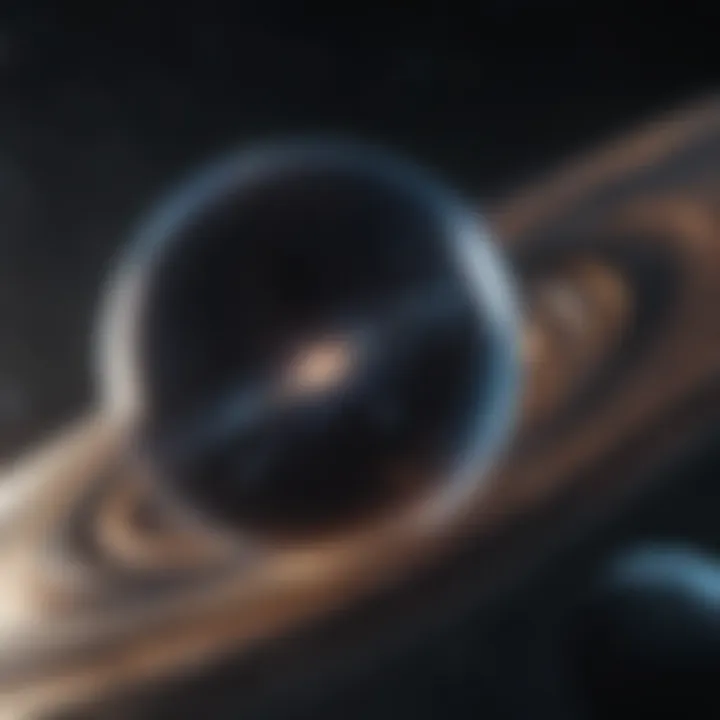
Intro
Gravity is one of the four fundamental forces known to humanity. In the context of space, it takes on additional significance, shaping the cosmos in ways that influence everything from the formation of stars to the behavior of galaxies. Understanding gravity is vital for students, researchers, and space enthusiasts alike, as it is a cornerstone of astrophysics and space exploration. This article delves into various aspects of gravity in space, from fundamental principles to their applications.
Key Research Findings
Overview of Recent Discoveries
Over the last few decades, numerous studies have advanced our understanding of gravitational forces. Notable discoveries include the detection of gravitational waves and the insights gained from observing black holes. These phenomena have reshaped theories surrounding general relativity.
Recent observations by the LIGO (Laser Interferometer Gravitational-Wave Observatory) have confirmed the existence of gravitational waves, which are ripples in spacetime. This finding supports predictions made by Albert Einstein over a hundred years ago. Understanding these waves may one day allow scientists to detect events such as colliding black holes or neutron stars, providing a new way of observing the universe.
Significance of Findings in the Field
The recent advances in gravitational research have profound implications. For instance:
- They enhance our understanding of the life cycle of celestial bodies.
- They inform models predicting the structure and evolution of the universe.
- They contribute knowledge that may lead to revolutionary technologies in space travel and satellite communication.
Gravitational phenomena impact everything from the motion of planets to the trajectory of spacecraft. Thus, the ongoing exploration of gravity is essential for future advancements in astrophysics and beyond.
Breakdown of Complex Concepts
Simplification of Advanced Theories
Gravitational physics often involves complex theories. Simplifying these concepts can help elucidate their significance. For instance, the principle of microgravityโan environment where gravity is significantly weakerโhas been studied in detail. In such conditions, objects behave differently than on Earth. Understanding this helps researchers prepare for long-duration space missions, affecting everything from human health to material science.
Visual Aids and Infographics
Using visual aids can clarify intricate ideas about gravity. Diagrams depicting gravitational fields or infographics explaining gravitational waves can provide context and enhance understanding.
"Visual representations of data can sometimes reveal insights that numbers alone cannot."
Equations related to gravitational force can also be represented visually. For example, the formula of Newton's law of universal gravitation offers a straightforward method to understand the relationship between mass and distance.
In summary, the exploration of gravity in space offers a rich tapestry of insights. As newer discoveries unfold, the influence of gravity will continue to be crucial in shaping our understanding of the universe.
Intro to Gravity in Space
Understanding gravity is crucial in the realm of space exploration and astrophysics. Our universe functions under the influence of gravitational forces, shaping the behavior of stars, planets, and galaxies. This section addresses the foundational elements of gravity in space, providing context on its significance and implications.
Gravitational forces dictate not only the orbits of celestial bodies but also the structure of the universe itself. Without gravity, the formation of planets, stars, and other heavenly bodies would be fundamentally affected, leading to a completely different cosmic landscape. Hence, grasping gravity's mechanics allows an insight into fundamental astrophysical phenomena.
The study of gravity in space promotes a deeper understanding of numerous complex concepts, from the life cycles of stars to the movement of galaxies. It impacts various fields, including physics, astronomy, and even biology. For instance, understanding how gravity operates in microgravity environments can help scientists comprehend physical changes in living organisms. Additionally, exploring the effects of gravity improves technological advancements for space missions, ultimately benefiting space agencies and researchers.
In summary, gravity in space is more than an academic topic; it is a gateway to understanding the universe. Through detailed exploration, we aim to highlight its influence not only on celestial formations but on our broader quest to comprehend the cosmos.
Defining Gravity
Gravity, at its core, can be defined as the force that attracts two bodies towards each other. Isaac Newton described this force in his law of universal gravitation. He formulated that every mass in the universe attracts every other mass with a force directly proportional to the product of their masses and inversely proportional to the square of the distance between their centers.
In simpler terms, this means that larger objects, like the Sun or Earth, exert a stronger gravitational pull than smaller objects, such as a car or a person. This interaction results in various phenomena, such as objects falling to the ground and planets orbiting stars.
Gravity is not only a constant of our earthly experience but also a fundamental force acting throughout the universe. Its universal nature reinforces the interconnectedness of all matter in space.
Historical Perspectives
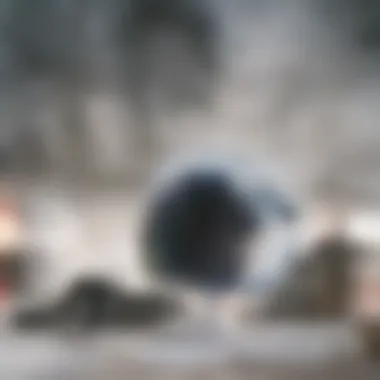
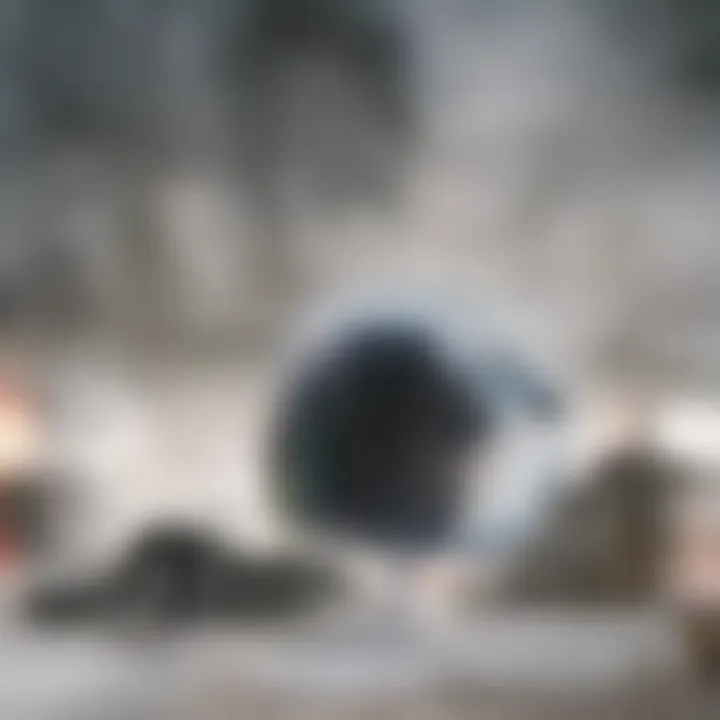
The history of gravity reflects humanity's evolving understanding of the universe. From early philosophers like Aristotle, who believed in natural places for elements, to the more structured theories introduced by Newton, significant shifts occurred.
Newton's work in the late 17th century laid the foundations for gravitational theory. His insights remained largely unchallenged until Albert Einstein presented general relativity in the early 20th century. This innovative approach redefined gravity, not merely as a force but as a curvature of spacetime caused by mass.
The acceptance of Einstein's theory marked a turning point in physics, impacting not just theoretical frameworks but also practical applications in navigation, astronomy, and space exploration.
This historical evolution emphasizes the importance of gravity in our understanding of the cosmos. It showcases a journey from simplistic ideas to complex, multi-dimensional frameworks that scientists continue to explore.
Fundamental Principles of Gravity
Understanding the fundamental principles of gravity is crucial for comprehending the intricate behaviors of celestial bodies and the universe at large. This section explores the cornerstone concepts of gravitational theory that have shaped our current scientific outlook. Each principle offers insights not only into how objects interact within the cosmos but also into the applications of these laws in various scientific fields.
- Clarity in Concepts: Clarity in the principles of gravity enables researchers and students to grasp the underlying mechanics of gravitational forces. This understanding is vital for advancements in astrophysics, engineering, and even philosophy as it delves into the nature of reality itself.
- Technological Applications: Modern technology relies heavily on gravitational principles. For instance, satellite navigation systems must account for gravitational variances to provide precise location data. Insights gained from gravity studies also inform the design of spacecraft.
- Interdisciplinary Relevance: Gravitational concepts are not confined to physics alone. They extend into fields like astronomy, geology, and environmental science. Grasping these principles fosters a holistic approach to scientific inquiry.
Newton's Law of Universal Gravitation
Isaac Newton's Law of Universal Gravitation states that every mass in the universe attracts every other mass with a force that is directly proportional to the product of their masses and inversely proportional to the square of the distance between their centers. This can be expressed with the formula:
[ F = G \fracm_1 m_2r^2 ]
Where:
- F is the gravitational force between two objects
- G is the gravitational constant
- m1 and m2 are the masses of the objects
- r is the distance between their centers
Newton's law provides a systematic way to calculate gravitational forces and is foundational for classical mechanics. It explains phenomena ranging from the orbits of planets around the sun to the motion of falling objects on Earth.
"Gravity explains the motions of the planets, but it cannot explain who sets the planets in motion."
โ Isaac Newton
Einstein's General Relativity
Einstein's General Relativity presents a more comprehensive framework for understanding gravity. It revolutionized the way we see gravitational forces by depicting gravity not just as a force but as a curvature of spacetime itself. According to this theory, massive objects like planets and stars warp the fabric of spacetime around them, and this curvature dictates how other objects move.
This perspective allows for the explanation of phenomena that Newton's laws cannot accurately address, such as:
- The bending of light around massive objects, known as gravitational lensing.
- The precise orbits of Mercury, which deviate from Newtonian predictions.
Einstein's ideas have been supported by numerous experiments and are a critical aspect of modern astrophysics, enabling advancements in our understanding of black holes, cosmic expansion, and more.
The Role of Spacetime
Spacetime is a four-dimensional continuum that combines the three dimensions of space with the dimension of time. Its role in gravity is essential. In this framework, time is as intertwined with the gravitational effects experienced by objects as space is.
Understanding spacetime leads to several significant insights:
- Time Dilation: Time passes at different rates in varying gravitational fields. For example, time moves slower close to a massive body compared to farther away.
- Black Holes and Events Horizon: The curvature of spacetime creates regions in space where the gravitational pull is so strong that nothing can escape, illustrating the profound implications of spacetime in defining cosmic structures.
The integration of time and space into the analysis of gravity leads to a deeper understanding of the universe's architecture and the fundamental forces that shape it.
Gravity's Impact on Celestial Bodies
Gravity plays a crucial role in shaping the universe and influencing the behavior of celestial bodies. It governs the dynamics of planetary systems, dictates the life cycles of stars, and determines the structure of galaxies. Understanding gravity's impact on celestial bodies allows us to comprehend not only their formation and evolution but also their interactions with one another.
Planetary Formation and Orbits
During the early stages of the solar system, gravity facilitated the accretion of dust and gas into larger bodies. This process led to the birth of planets, moons, and asteroids. As objects collided and merged, gravity exerted attraction, drawing more material into the growing planetary bodies.
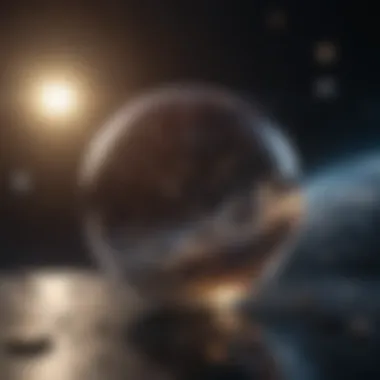
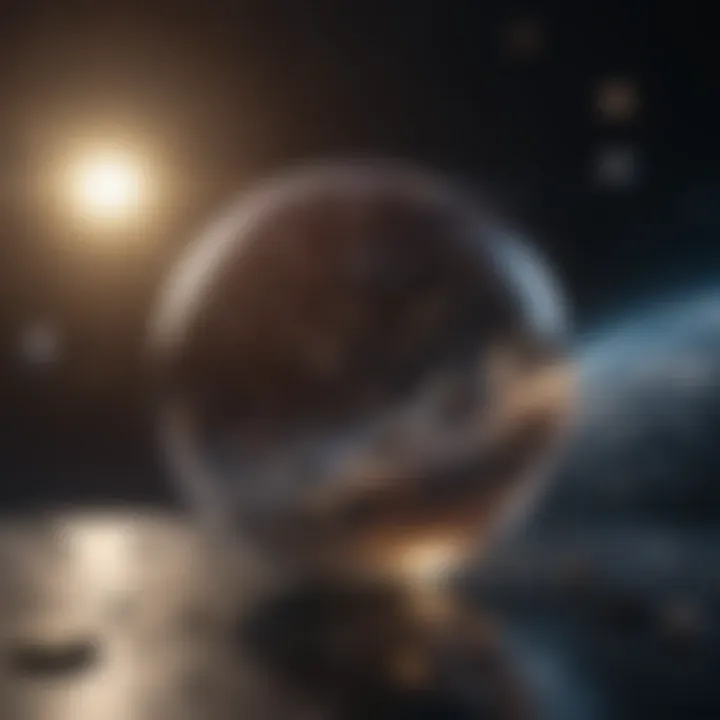
Once formed, celestial bodies entered into orbits defined by gravitational forces. The strength of gravity varies with mass and distance; thus, larger planets exert a stronger gravitational pull. For instance, Jupiter, the largest planet in our solar system, exhibits significant gravitational influence, affecting the orbits of nearby moons and even smaller asteroids.
Key points regarding planetary formation and orbits include:
- Material Accretion: Gravity pulls particles together to build larger celestial objects.
- Orbital Dynamics: The balance between gravitational pull and inertia keeps planets in stable orbits.
- Tidal Forces: Variations in gravity result in tidal effects, influencing environmental factors on planets, such as ocean levels.
Black Holes and Gravitational Singularity
Black holes represent one of the most enigmatic manifestations of gravity. Formed from the remnants of massive stars after they undergo supernova explosions, black holes possess gravitational fields so strong that nothingโnot even lightโcan escape their pull. This phenomenon is termed gravitational singularity, where density becomes infinite, and the known laws of physics cease to function adequately.
The study of black holes provides insight into the nature of gravity. According to Einstein's general relativity, the curvature of spacetime around a black hole shows how gravity bends the fabric of the universe itself. Understanding black holes helps scientists explore the limits of gravitational strength and the potential for phenomena such as gravitational waves.
Important aspects of black holes and gravitational singularity include:
- Event Horizon: The boundary surrounding a black hole, beyond which no information can escape.
- Gravitational Waves: Ripples in spacetime produced during massive cosmic events, which can be detected by instruments like LIGO.
- Astrophysical Implications: Black holes challenge our understanding of physics, exploring conditions under extreme gravity.
Gravitational Lensing Effects
Gravitational lensing occurs when a massive object, like a galaxy or a black hole, bends the light from a distant object due to its strong gravitational field. This phenomenon allows astronomers to observe objects that are otherwise hidden behind massive structures. The effect has provided valuable information about the distribution of dark matter in the universe and the expansion of space.
The significance of gravitational lensing in modern astronomy cannot be overstated. It serves as a natural telescope, enhancing our capability to investigate the cosmos. Through gravitational lensing, scientists can examine the light from distant galaxies, leading to discoveries about their formation and evolution.
Key features of gravitational lensing include:
- Strong Lensing: When the light is significantly bent, creating multiple images of the same celestial object.
- Weak Lensing: Subtle distortions in shapes of distant galaxies, allowing measurements of mass distribution.
- Cosmic Surveys: Gravitational lensing aids in identifying and studying exoplanets, dark matter, and other cosmic phenomena.
Thus, gravity's impact on celestial bodies is profound, shaping the structure and dynamics of our universe. Understanding these interactions illuminates fundamental concepts in astrophysics and inspires future exploration.
Microgravity Environments
Understanding microgravity environments is essential for grasping the complexities of living and conducting research in space. Microgravity refers to a condition of continuous free fall, which creates an environment where the effects of gravity are drastically reduced. This situation arises in space, particularly in orbiting spacecraft, where objects experience weightlessness. The significance of microgravity extends beyond mere physical sensations; it influences biological, chemical, and physical processes in ways that differ remarkably from conditions on Earth.
Living Organisms in Space
The study of living organisms in microgravity environments unveils important insights into biological adaptations. When organisms are in space, they experience altered conditions that affect their growth, reproduction, and behavior. For example, bacteria can exhibit accelerated growth rates in space, and certain plant species may display changes in directionality of growth.
Research on the International Space Station (ISS) has provided significant data about how muscles and bones react under microgravity. Studies indicate that astronauts lose muscle mass and bone density during prolonged exposure. This knowledge is instrumental in developing countermeasures for maintaining health in long-duration missions, such as those planned for Mars.
Microgravity Effects on Human Physiology
The effects of microgravity on human physiology are profound and multifaceted. Astronauts face various physiological changes, including fluid redistribution, decreased cardiovascular fitness, and alterations in sensory perceptions. For instance, the heart tends to reshape and become more spherical in space due to the absence of gravitational force on its shape. Additionally, changes in vision have been documented, which detrimentally influence astronauts' performance in missions.
Research has shown that microgravity induces a state resembling aging. The immune system becomes less effective, increasing the risk of illness. Therefore, understanding these effects is critical for preparing astronauts for future explorations. This information helps form a comprehensive health management program, ensuring the safety and effectiveness of space personnel.
Applications in Scientific Research
Microgravity environments serve as unique laboratories for scientific research, allowing for experiments that cannot be conducted on Earth. The lack of gravity leads to precise control over variables in experiments, providing clearer results. Fields such as material science, fluid dynamics, and biotechnology benefit significantly from this research.
- Material Science: In microgravity, materials can be processed without the influence of gravity-driven events such as sedimentation or convection. This results in better quality materials with unique properties.
- Fluid Dynamics: The behavior of liquids differs significantly in microgravity, as surface tension becomes a dominating force. Understanding these principles can improve technologies on Earth.
- Biotechnology: Microgravity influences gene expression and protein crystallization, which are critical for drug development. Instruments designed for research on the ISS can lead to breakthroughs in pharmaceuticals.
"Microgravity environments are not just a challenge, but also a gateway for scientific innovation and discovery."
Gravitational Waves and Astrophysics
Gravitational waves represent one of the most significant advancements in the field of astrophysics. This section delves into the profound implications these waves hold for our understanding of the universe. Gravitational waves, which are ripples in spacetime caused by some of the most violent cosmic events, provide a unique perspective on the nature of gravity and its interactions. The ability to detect these waves opens up new avenues for observational astronomy and cosmology.
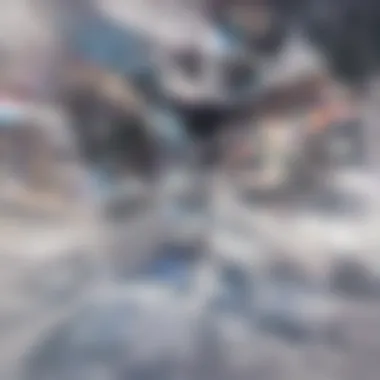
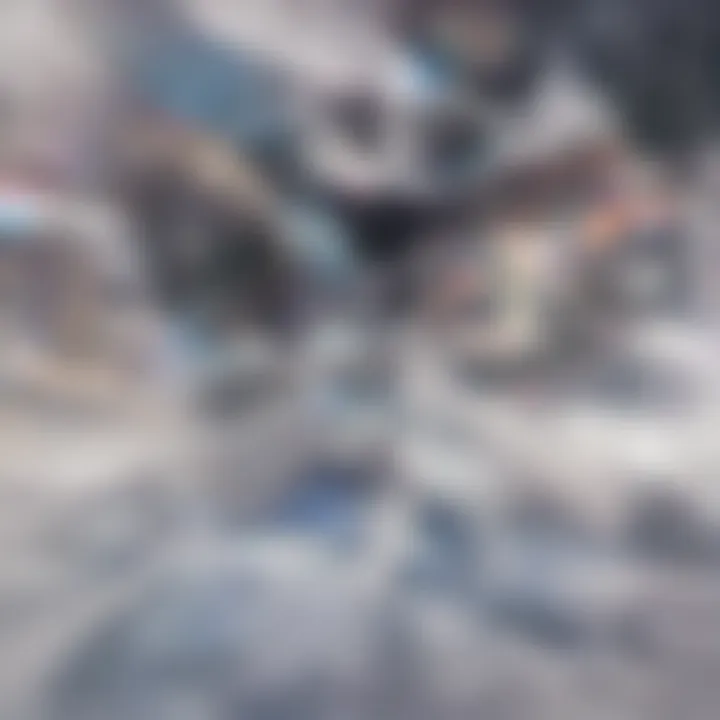
The detection of gravitational waves allows scientists to study phenomena that are otherwise invisible through traditional electromagnetic observations. Events such as black hole mergers, neutron star collisions, and supernova explosions generate gravitational waves that carry essential information about the processes involved. This information can enhance our comprehension of fundamental physical laws and challenge existing theories in gravity.
Theory and Detection of Gravitational Waves
The theoretical foundation for gravitational waves was laid by Albert Einstein in his General Theory of Relativity. Einstein proposed that massive objects warp spacetime around them. When these objects accelerateโlike when two black holes orbit each otherโthey generate ripples in the fabric of spacetime. These ripples propagate outward at the speed of light and can be detected on Earth by extremely sensitive instruments.
The LIGO (Laser Interferometer Gravitational-Wave Observatory) and Virgo observatories are pivotal in the detection of these waves. LIGO, in particular, made history in September 2015 by detecting gravitational waves from merging black holes. This monumental moment confirmed Einstein's predictions and opened a new era in astrophysics. The waves detected are incredibly faint, necessitating advances in technology to ensure precision in measurement.
"The discovery of gravitational waves is one of the most significant scientific achievements of the 21st century, reshaping our understanding of the universe."
Implications for Cosmology
The implications of gravitational waves are vast, particularly in the realm of cosmology. They offer a new method to explore the universe and gain insights into the early moments of the cosmos. By analyzing the signals from gravitational waves, researchers can infer properties about the objects that created them, such as mass, spin, and distance.
This information is crucial for addressing fundamental questions in cosmology:
- Understanding dark matter and dark energy: Gravitational wave observations can provide indirect evidence regarding the nature and distribution of dark matter and energy in the universe.
- Studying the expansion of the universe: By using gravitational waves, scientists can measure distances more accurately, thereby refining the Hubble constant and our understanding of the universe's expansion rate.
- Probing the very fabric of spacetime: Gravitational wave studies may lead to insights into quantum gravity and the underlying structure of spacetime beyond the current theoretical frameworks.
As research progresses, gravitational waves are set to enhance our comprehension of both the cosmos' grand structure and the minute interactions of particle physics.
Future Directions in Gravitational Research
Understanding gravity in space is not just about its current implications; it is also about anticipating future advancements in the field. The study of gravity is entering an exciting era with the potential for groundbreaking discoveries. As we enhance our technological capabilities, we can explore phenomena that were once thought to be purely theoretical. Researchers seek to probe deeper into gravitational physics, which can yield insights not just into gravity itself, but also into the foundational mechanics of the universe.
Advancements in Technology and Methodology
Recent developments in technology play a crucial role in gravitational research. Instruments designed to detect gravitational waves, such as LIGO (Laser Interferometer Gravitational-Wave Observatory), have revolutionized our ability to observe cosmic events. These detectors measure ripples in spacetime, created by massive actions such as black hole mergers or neutron star collisions. The precision of these instruments allows scientists to gather data that was previously inaccessible.
Moreover, the rise of advanced computational models enables researchers to simulate complex gravitational interactions accurately. This enables the analysis of scenarios that occur on cosmic scales and provides a deeper understanding of gravitational dynamics. Collaboration with data science and artificial intelligence is proving beneficial for data interpretation and enhances model accuracy.
Advancements in space missions also contribute significantly. For instance, the European Space Agency's (ESA) LISA (Laser Interferometer Space Antenna) project aims to detect gravitational waves in space. This development can complement Earth-based detectors and provide insights into the early universe's gravitational landscape.
Interdisciplinary Approaches
Future gravitational research increasingly benefits from interdisciplinary approaches. Fields such as astrophysics, quantum mechanics, and cosmology converge, leading to a more comprehensive understanding of gravitational phenomena. Collaboration across disciplines fosters innovative research methodologies, unearthing complex relationships that might otherwise go unnoticed.
For instance, understanding dark matter and dark energy is crucial for grasping gravitational influences on cosmic scales. This requires input from both particle physics and gravitational theory. Joint research efforts enable the creation of unified models, enhancing our overall comprehension of the universe.
Additionally, community engagement and openness to various academic perspectives enrich research outcomes. Knowledge-sharing platforms, forums, and collaborations worldwide help cultivate an environment where innovative approaches to gravitational research can thrive.
Culmination
The exploration of gravity in space is a vital aspect of understanding the universe. Analyzing the fundamental principles of gravity enhances our comprehension of celestial mechanics and phenomena. This article has highlighted not only the traditional views from Newton and Einstein but also the implications of these theories in modern astrophysics. The insights gathered here demonstrate how gravity affects the formation and evolution of galaxies, stars, and planets, shaping them through gravitational forces.
Summary of Key Insights
The key takeaways from this article emphasize the following:
- Fundamental Theories: Newton's Law and Einstein's General Relativity provide a comprehensive framework for understanding gravity.
- Celestial Impact: Gravitational forces dictate orbits and interactions between celestial bodies.
- Microgravity Studies: Understanding how microgravity impacts life can lead to advances in medicine and biology.
- Gravitational Waves: Detection of these waves opens new avenues in cosmology, providing insight into powerful cosmic events.
The Role of Public Understanding of Gravity
The publicโs grasp of gravitational concepts is essential for fostering interest in science and technology. It helps bridge gaps between complex theories and everyday understanding. An informed public can appreciate innovations stemming from gravitational research, which can enhance STEM education and inspire future generations of scientists.
- Engagement through Education: Institutions can promote workshops and lectures on gravitational concepts.
- Accessible Resources: Simplifying academic jargon in literature makes science more relatable.
- Public Interest in Research: Community involvement in space research initiatives can boost funding and support.
Ultimately, understanding gravity not just as a physical phenomenon but as a fundamental aspect of our universe can inspire curiosity and advancement in scientific exploration.
"Knowledge of gravity opens pathways to understanding the universe's grandeur and complexity."
Through continuous research and public engagement, gravity will remain a cornerstone of our quest to decode the universe.