Exploring the Future of Quantum Computing Innovations
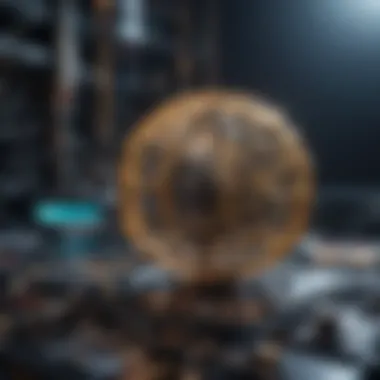
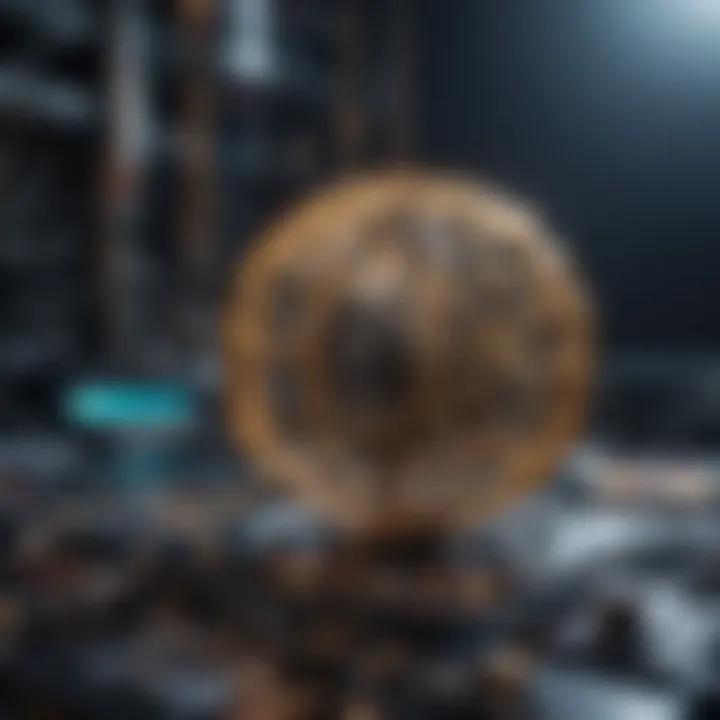
Intro
The landscape of computing is poised for dramatic transformation with the advent of quantum computers. While traditional computers process information in a linear fashion, quantum computing introduces a fundamentally different paradigm. This shift isn't just about increased speed; it's about redefining what's possible in various domains. From handling sizeable datasets with precision to tackling problems that were once deemed insurmountable, the implications are vast.
Understanding the potential of quantum computing goes beyond the realm of computer science. It intertwines itself with fields like mathematics, physics, and even philosophy, reshaping our approach to problem-solving. As we delve deeper into this topic, we will uncover critical findings from current research and discuss their relevance across diverse sectors.
To start with, let's look into the key research findings that are shaping the future of quantum technology.
Foreword to Quantum Computing
In this new era of technology, quantum computing stands at the frontier of what’s possible. Understanding quantum computing is essential, as it opens up a world of opportunities across various domains like cryptography and artificial intelligence. For students, researchers, and professionals, grasping the fundamentals of this complex field will shape how we approach challenging problems and even transform entire industries.
At its core, quantum computing harnesses the peculiar aspects of quantum mechanics. Traditional computing relies on bits as the smallest unit of data, representing either a 0 or a 1. In contrast, quantum computing uses quantum bits, or qubits, which can exist in multiple states simultaneously. This unique characteristic allows quantum computers to process information in ways that classical computers can’t, potentially solving complex problems at unprecedented speeds.
The implications of quantum computing extend far beyond faster calculations. As we delve into this subject, we’ll explore its potential to revolutionize data analysis, simulation technologies, and machine learning. The journey begins with a clear definition of quantum computing, followed by a historical overview that traces its development from theoretical concept to reality.
"Quantum computing isn't just a technological advance; it's a paradigm shift in how we understand and process information."
Defining Quantum Computing
Defining quantum computing involves understanding how it diverges fundamentally from classical computing. In classical systems, bits are linchpins that dictate binary processes. In quantum systems, qubits can exist in a state of superposition — think of it like being in two places at once. This means they can represent both 0 and 1 simultaneously, allowing quantum computers to conduct multiple calculations at once.
Furthermore, qubits can also be entangled. This property allows qubits that are paired to instantaneously influence one another, regardless of the distance separating them. This interplay enables quantum computers to perform calculations that would take classical computers impractically long to solve. Thus, quantum computing essentially redefines how we approach complex data processing and problem-solving.
Historical Context and Developments
The history of quantum computing is a tapestry woven with great intellect and innovation. It dates back to the early 1980s, when physicist Richard Feynman suggested that classical computers could not efficiently simulate quantum systems. In 1985, David Deutsch built upon this idea, providing the first coherent model of a quantum computer.
The journey continued with the introduction of algorithms like Shor's algorithm, designed to factor large numbers efficiently, offering profound implications for cryptography. In the subsequent decades, research in quantum mechanics and computing gained momentum, punctuated by both theoretical advancements and practical experiments.
Today, organizations like Google and IBM have made significant strides towards building functional quantum computers. Their efforts hint at a future where industries can harness quantum algorithms to solve real-world problems.
In summary, the introduction to quantum computing lays the groundwork for understanding how and why this technology is reshaping our world. As we continue, we’ll dissect its fundamental principles, key advancements, and potential applications, illuminating the exciting and unpredictable path ahead.
Fundamentals of Quantum Mechanics
Understanding the fundamentals of quantum mechanics is absolutely indispensable to grasp the essence and potential of quantum computing. This field serves as the backbone for all the advancements we are witnessing today in quantum technology. A firm understanding of quantum principles is crucial for those in academia and industry, as it influences how algorithms are developed, how hardware is designed, and how applications emerge. By delving into these fundamental topics, we can better appreciate not just how quantum computing works, but also why it offers revolutionary solutions to problems that classical computing struggles with.
Superposition and Entanglement
Superposition and entanglement are the twin pillars of quantum mechanics, providing the framework for quantum computing's potential. Superposition allows quantum bits, or qubits, to exist in multiple states at once, unlike classical bits, which are decidedly either on or off. Imagine flipping a coin—while it's spinning, it embodies both heads and tails simultaneously. This quality enables quantum computers to process vast amounts of information simultaneously, thereby increasing computational speed exponentially.
Entanglement, on the other hand, is the phenomenon where pairs or groups of qubits become intertwined such that the state of one qubit immediately influences the state of another, no matter how far apart they are. This spooky action at a distance not only leads to faster data exchange but also unlocks capabilities that classical systems can't match. To quote physicist Einstein, "God does not play dice with the universe," yet here quantum mechanics challenges that belief, suggesting that random outcomes can develop interconnected states.
Quantum Bits: Qubits Explained
At the core of quantum computing rests the qubit. Unlike a traditional binary bit, which can only be in one of two states (0 or 1), a qubit can realize a combination of both states simultaneously due to superposition. This characteristic fundamentally alters how computations are carried out, making quantum computers particularly efficient at specific tasks.
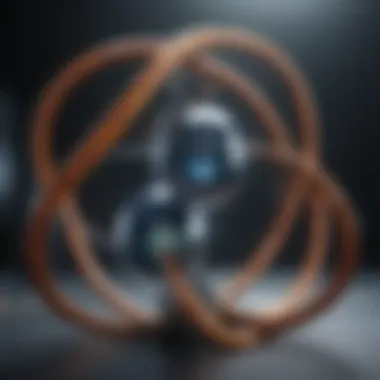
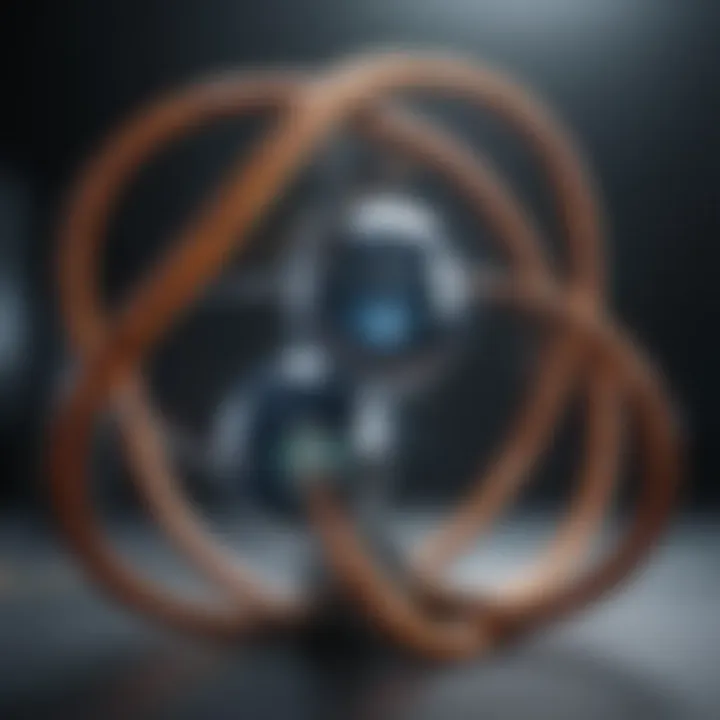
The implementation of qubits varies. Some common types include ion trap qubits, superconducting qubits, and topological qubits. Each comes with its own set of advantages and challenges, while researchers persist to refine the technology. For instance, superconducting qubits are promising due to their relatively fast operation speeds, yet they face issues with decoherence, which can often disrupt calculations. Understanding these complexities is crucial for anyone venturing into the field.
Quantum Gates and Circuits
Just as classical computers use logic gates to manipulate bits, quantum computers utilize quantum gates to operate on qubits. A quantum gate enables the control of qubit states through quantum operations. These gates can perform various transformations, facilitating the creation of quantum circuits that can execute complex algorithms in a fraction of the time needed by classical computers.
The beauty of quantum circuits lies in their ability to perform numerous calculations in parallel. This parallelism arises from the unique properties of qubits, producing potential solutions to problems involving multifaceted datasets or intricate calculations. For clarity, consider Grover's algorithm:
"Quantum mechanics is the science of the small, and its principles guide us into realms beyond our everyday comprehension."
By exploring these foundational concepts, students, researchers, educators, and professionals will find themselves better equipped to navigate the complexities and prospects in the exciting world of quantum computing.
Advancements in Computational Power
The rapid progression in quantum computing technology marks a turning point in how we approach complex calculations and data processing. This section unpacks the importance of advancements in computational power in the context of quantum computing, illuminating key elements, benefits, and pertinent considerations.
Solving Complex Problems
One of the standout features of quantum computing is its potential to tackle problems that are beyond the reach of classical computers. Take, for instance, the intricacies involved in drug discovery. Traditional methods may require months or even years to simulate molecular interactions. In quantum computing, the combination of superposition and entanglement allows for the simultaneous evaluation of countless possibilities. This means researchers can explore potential drug candidates in a fraction of the time.
Moreover, fields like climate modeling benefit immensely from quantum capabilities. By accurately handling vast datasets and complex variables, quantum systems can generate more precise predictions that inform better decision-making. Imagine trying to predict weather patterns for a whole region based on numerous factors—it’s like finding a needle in a haystack for classical systems, but quantum computing could shine a light right on that needle.
Quantum Supremacy and Its Implications
Quantum supremacy refers to the phase when a quantum computer can perform a computation that a classical computer cannot achieve in any feasible time frame. This milestone, once a theoretical aspiration, was achieved by Google in 2019. The implications of this breakthrough resonate far beyond academic circles.
When Google’s quantum computer solved a specific problem in 200 seconds—a task that would take the fastest supercomputers thousands of years—it showcased the practical applications that lie ahead. Many industries stand to gain tremendously from this leap, especially sectors like finance and logistics. For example, quantum algorithms could optimize trade routes in real time or enhance risk assessments in investment portfolios.
However, the road to realizing the complete potential of quantum supremacy isn’t without its bumps. Questions arise about security and ethical concerns as quantum capability expands. How will existing encryption methods withstand the onslaught of quantum decryption powers? This need for thoughtful navigation is crucial as we move forward in the quantum era.
Quantum computing is no longer just an idea of future; it’s becoming reality in ways that will reshape industries.
The stakes are monumental, bearing the weight of responsibility for researchers and technologists globally. Thus, with advancements in computational power, particularly focusing on solving complex problems and paving ways to achieve quantum supremacy, we step into an era with vast unexplored territory and possibilities.
Applications of Quantum Computing
Understanding the applications of quantum computing is pivotal, especially when considering the future landscape of technology and industry. This section delves into the practical implementations of quantum computing and what can be gained from its unique principles. From cryptography to AI developments, the ramifications of harnessing quantum computing capabilities resonate through various sectors. Each segment highlights how quantum computing is not just theoretical; its potential is becoming increasingly tangible in solving real-world challenges.
Cryptography and Security
The world of cryptography is undergoing a seismic shift due to the advent of quantum computing. Traditional encryption methods rely heavily on complex algorithms that are difficult to break using classical computers. However, quantum systems utilize unique principles like superposition and entanglement, enabling them to solve these complex problems, often exponentially faster than their classical counterparts.
With quantum computers, the widely used RSA and ECC (Elliptic Curve Cryptography) could be rendered vulnerable. They depend on the factorization of large numbers and the discrete logarithm problem, both of which quantum computers can tackle efficiently using Shor's algorithm. As a response, researchers are racing to develop quantum-resistant algorithms. These new methods aim to safeguard our data against potential future threats from fully capable quantum devices.
"The arrival of quantum computing could redefine the security landscape, necessitating a reevaluation of data protection mechanisms across all sectors."
Quantum Simulation for Material Science
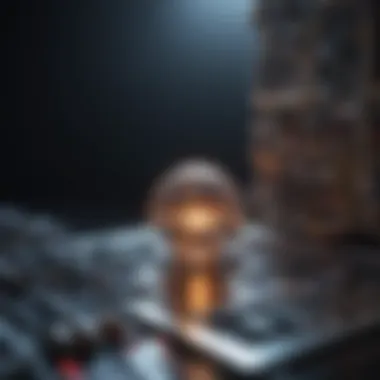
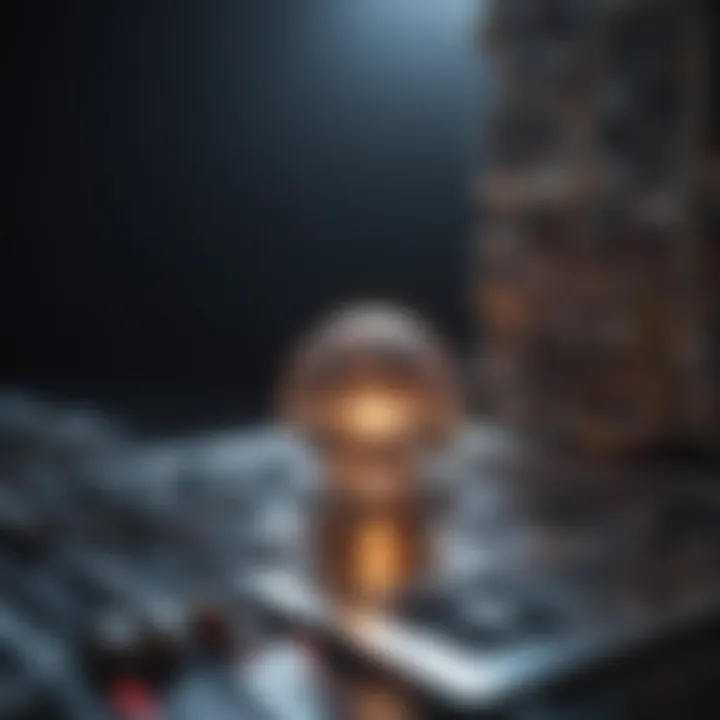
Quantum simulation plays a crucial role in advancing material science. The behavior of materials at the quantum level is inherently complex, governed by the principles of quantum mechanics. Classical computers struggle with simulating these states, particularly when dealing with many-body problems associated with quantum materials.
By leveraging quantum computers, researchers can simulate molecular interactions with unprecedented accuracy. This has significant implications for fields such as drug discovery, where understanding protein folding or molecular binding can lead to breakthroughs in medicine. Additionally, the development of new materials—superconductors, for instance—could catapult advancements in energy storage and quantum computing hardware itself.
Optimization Problems in Various Industries
Optimization problems arise across various industries, from finance to logistics. Quantum computing offers a fresh angle to tackle these issues, facilitating solutions that classical computing often finds tedious or near impossible within a reasonable time frame.
For instance, in supply chain management, optimizing routes and inventory can lead to massive savings. Quantum algorithms, such as the Quantum Approximate Optimization Algorithm (QAOA), promise to find better solutions faster.
Key benefits include:
- Increased efficiency in resource allocation
- Enhanced predictive analytics
- Improved decision-making speed in dynamic environments
Industries that rely on optimization can expect transformative changes, allowing them to operate more effectively and competitively in an increasingly complex world.
Impact on Artificial Intelligence
AI and quantum computing are two cutting-edge domains that can synergistically advance innovations. Quantum computing can speed up machine learning tasks by processing large datasets faster than classical systems. Algorithms like Grover's search can substantially enhance the capabilities of AI technologies, making them smarter and more efficient.
Moreover, using quantum mechanics in AI often leads to exploring new avenues, such as quantum neural networks. These networks can represent complex relationships and patterns in data, potentially reaching insights that traditional AI methods might not uncover. This dual advancement could lead to breakthroughs in various areas, including natural language processing, computer vision, and beyond.
In summary, the applications of quantum computing span numerous fields, unlocking extraordinary potential and addressing challenges considered insurmountable until now. With ongoing research and collaboration, the vision for quantum computing continues to evolve, indicating a promising horizon ahead.
Challenges and Limitations
The realm of quantum computing teems with promise, yet significant hurdles can’t be overlooked. As with any emerging technology, the barriers to practical implementation pose both challenges and limitations that must be navigated. Appreciating these obstacles is crucial for a thorough understanding of just how far quantum computing has progressed and where it is headed. Addressing these issues is not merely an exercise in futility; it influences funding, research focus, and the trajectory of future advancements in the field.
Technological Hurdles in Quantum Hardware
When one dips into the nitty-gritty of quantum computing, it quickly becomes apparent that the hardware is not easily constructed. Developing robust and stable quantum bits—commonly called qubits—is a daunting task. Qubits can take various forms, such as ions trapped in electromagnetic fields or superconducting circuits, but each type presents unique complexities.
For instance, superconducting qubits require extremely low temperatures to maintain their quantum state. These frigid conditions demand expensive dilution refrigerators, driving up the cost and limiting the operational scale. Furthermore, creating systems that can interconnect multiple qubits effectively remains a perennial challenge. High-quality qubit interconnects are essential for building scalable quantum computers capable of running complex algorithms.
"Quantum hardware is a bit like trying to balance on a tightrope while juggling flaming swords. Failures can happen in the blink of an eye, and fixing them isn't always straightforward."
Moreover, integrating these set-ups into a functional quantum computer means addressing issues like physical limitations in materials and the intricacies of circuit design. A minor flaw in qubit manipulation can skew results and render lengthy computations futile.
Issues of Error Rates and Decoherence
In quantum computing, error rates and the phenomenon known as decoherence are often intertwined challenges. Decoherence occurs when quantum states lose their coherence due to interactions with the environment, resulting in the loss of the very quantum information we seek to harness. Essentially, this means that qubits can easily fall prey to noise and disturbances.
Error correction techniques exist, but they require additional qubits, which compounds the challenge. The effectiveness of these strategies can vary vastly among different quantum architectures. As a rule of thumb, the higher the level of error correction required, the more qubits are needed, which further complicates the already intricate hardware landscape.
To give some perspective, current quantum computers may boast error rates above 1%, which might seem small—until you consider the amount of calculations required in complex quantum algorithms. Just think about it: a string of a thousand operations, even with a 1% error rate, likely means at least ten errors, creating a formidable challenge for quantum processors.
In summary, while the ambitions and promises of quantum computing shine brightly in the horizon, the technological hurdles along the way are substantial. Understanding these challenges, particularly regarding hardware limitations and error management, not only lays bare what’s at stake but also shapes the very future of this groundbreaking technology.
Future Prospects of Quantum Computing
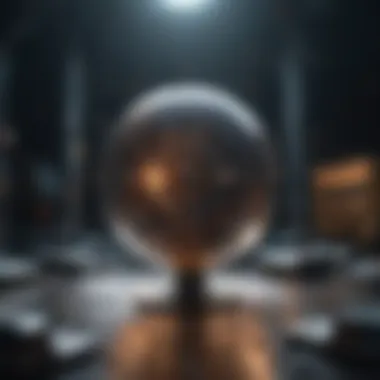
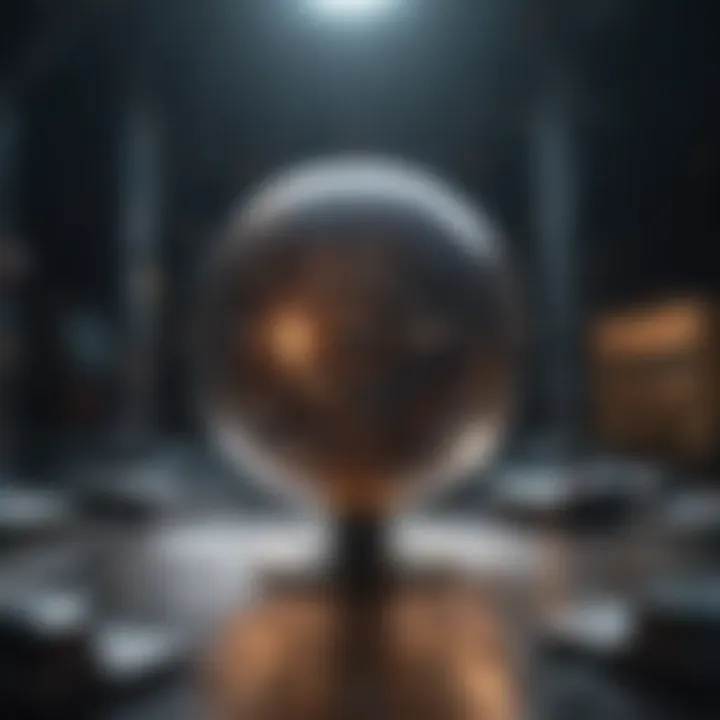
The future of quantum computing stands as a beacon of promise and intrigue, suggesting possibilities that could reshape industries and innovate approaches to longstanding problems. The advancements in this area aren’t just about boosting processing power; they encompass the collaboration among diverse fields, educational growth, and ethical frameworks necessary to navigate this exciting frontier. As we move forward, the convergence of these elements becomes crucial in harnessing the full potential of quantum technology.
Interdisciplinary Collaborations
One of the most pivotal aspects of quantum computing is its ability to bring together different disciplines, working hand-in-hand toward shared goals. Fields like computer science, physics, engineering, and even biology are starting to synchronize their methods and approaches to tackle the complex challenges posed by quantum systems.
The collaboration often leads to revolutionary ideas. For instance, physicists apply principles of quantum mechanics while engineers translate these theories into tangible innovations. When researchers from different backgrounds come together, they not only elevate their understanding but also generate solutions that transcend conventional boundaries.
- Example: Consider the project that involves quantum computing in drug design. Here, chemists, computer scientists, and pharmacologists unite to accurately simulate molecular interactions. It’s like pulling the right pieces from a puzzle to unveil the bigger picture of how new medicines can be developed.
This interdisciplinary approach also fuels innovation in educational strategies, enhancing the learning environment for students and professionals alike.
Educational Implications and Skill Development
As quantum computing continues to evolve, so does the need for a workforce skilled in this emerging technology. Education programs must adapt to include quantum theory, programming, and hardware design. This isn't just a passing phase; the demand for knowledgeable individuals in quantum tech will more than likely stabilize in the long run.
- Skill Areas to Focus On:
- Quantum algorithms and programming languages (like Q# or Cirq)
- Understanding quantum entanglement and superposition
- Problem-solving using quantum mechanics principles
Educational institutions have a unique opportunity to tailor their curriculums. Through offering specialized courses and encouraging hands-on experiments, schools can strike a balance between theory and practical application. This will not only help produce adept professionals but also foster a culture ripe for innovation and discovery.
Potential Ethical Considerations
As with any formidable technology, the rise of quantum computing is not without its ethical quandaries. Questions around privacy, security, and access become paramount as this technology matures. Since quantum computers could potentially break current encryption standards, organizations and governments must remain vigilant regarding cyber threats.
- Key Considerations:
- Privacy: How will data protection evolve under quantum influences?
- Equity: Will powerful tools be concentrated in the hands of a few?
- Transparency: How do we ensure the responsible use of quantum technology?
Approaching these ethical questions requires input from not just technologists, but philosophers, sociologists, and policymakers as well. A multidisciplinary response will ensure that quantum computing advances society rather than disrupt it.
In summary, the future prospects of quantum computing come wrapped in layers of collaboration, educational shifts, and ethical reflections. The landscape ahead is both challenging and exhilarating, and how we navigate these waters can determine the extent of the technology's benefits for generations to come.
Ending
In wrapping up our exploration of quantum computing, it’s essential to grasp the significance of the insights we've covered. Quantum computing represents not just a shift in computational capabilities, but a transformation across multiple sectors, extending from scientific research to everyday applications.
Recapitulating Key Insights
This journey through quantum computing allowed us to highlight several pivotal aspects:
- Interdisciplinary Nature: The development of quantum technologies relies heavily on collaborations across physics, engineering, and computer science. This fusion of disciplines fuels innovation and speeds up advancements.
- Real-World Impacts: From revolutionizing cryptography to enhancing artificial intelligence, the practical applications outlined throughout our article showcase just how profound this technology can be. Case studies demonstrated how specific industries are gearing up to leverage quantum solutions.
- Challenges Ahead: Despite its potential, the road forward is riddled with technological hurdles. We explored the significance of addressing issues such as error correction and decoherence, which are pivotal for the successful implementation of quantum computing.
By recapping these insights, we establish a foundation for understanding not just where quantum computing stands today, but where it could lead us in the future.
The Continuing Journey of Quantum Computing
The future of quantum computing is as promising as it is uncertain. It’s akin to planting a seed in fertile ground—there is potential for growth, yet many factors influence how far those roots can spread. As researchers delve deeper into quantum algorithms and error correction techniques, new breakthroughs are anticipated.
"Quantum computing is like a compass guiding us through uncharted territory, where algorithms yet to be conceived could reshape our understanding of complex systems."
- Innovation in Education: As quantum computing technologies mature, it’s crucial to adapt educational frameworks to prepare upcoming generations. Integrating quantum physics and computational theory into curricula ensures that the next crop of researchers and engineers are well-equipped.
- Ethical Considerations: With great power comes responsibility. The discussion surrounding ethical implications in quantum computing is gaining momentum. As algorithms that can break traditional security models emerge, the conversation about protecting data integrity and privacy becomes imperative.
- Continued Research and Development: The ecosystem of quantum computing is ever-changing. Engaging in open-source projects or communities, like those found on Reddit or through collaborations highlighted on platforms such as Facebook, can foster a culture of innovation and shared knowledge.
As the boundaries of quantum computing stretch ever forward, it’s the concerted efforts of scholars, professionals, and technologists that will shape this field's destiny. Understanding this trajectory is not merely academic; it’s foundational for harnessing the forthcoming advancements that quantum computing offers.