Exploring the All-Magnetic Universe: Principles & Applications
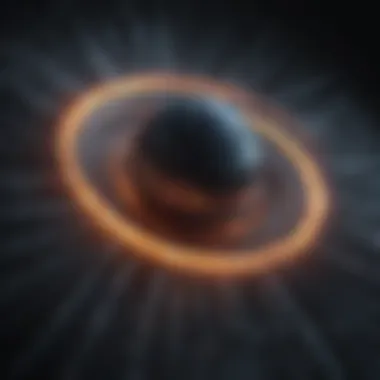
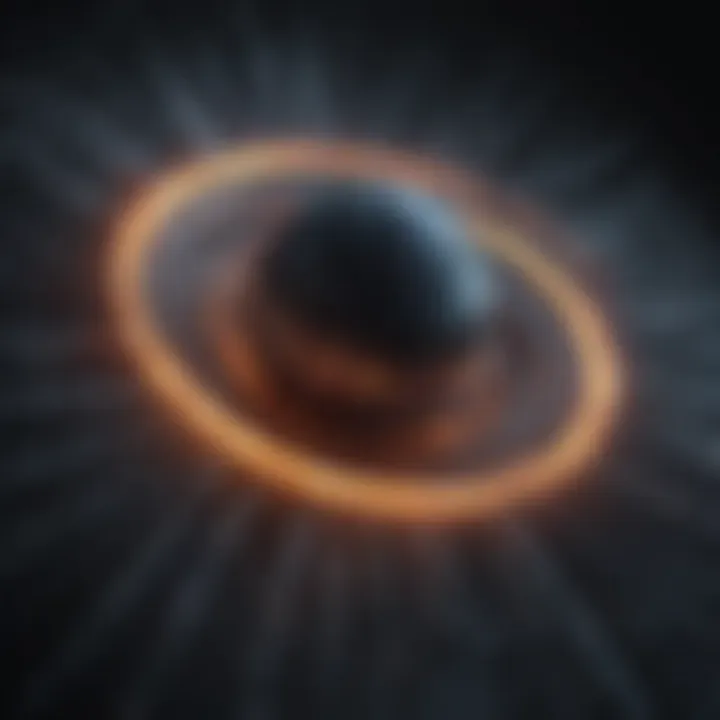
Intro
Magnetism is an essential force in our universe, a phenomenon that influences various aspects of life and technology. As we explore this topic, we will examine the fundamental principles of magnetism while delving into its applications across multiple fields. This article seeks to provide a well-rounded perspective on magnetism, from its classical roots in physics to its implications in emerging areas such as quantum mechanics and material science. Understanding the intricacies of magnetism can lead to significant advancements in technology and enhance scientific inquiry.
Key Research Findings
Overview of Recent Discoveries
Recent studies in magnetism have revealed groundbreaking insights that challenge traditional views and expand our understanding. For instance, researchers have made headway in the study of topological insulators, materials that exhibit unique magnetic properties due to their electronic structure. These findings not only contribute to theoretical physics but also have practical implications for developing advanced electronics, such as quantum computers and new battery technologies.
Another notable discovery is the identification of new magnetic materials, such as two-dimensional magnets, which present novel characteristics previously unseen in bulk materials. These materials hold promise for applications in spintronics, an area of technology that exploits electron spin to enhance performance in devices such as sensors and memory.
Significance of Findings in the Field
The recent advancements in magnetism play a crucial role in various scientific disciplines. They open avenues for innovation in efficient energy use and storage systems. The ability to manipulate magnetic properties on a nanoscale level allows for more precise control in electronic components.
Moreover, developments in magnetotherapy highlight the application of magnetic materials in healthcare, suggesting therapeutic benefits for conditions such as pain relief and inflammation reduction. These findings encourage interdisciplinary collaboration among physicists, engineers, and medical professionals to explore the potential of magnetism in health and technology.
"Magnetism is more than a physical force; it is an enabler of the future."
Breakdown of Complex Concepts
Simplification of Advanced Theories
To understand magnetism fully, it is imperative to break down complex theories into digestible parts. Classical magnetism, described by Maxwell's equations, focuses on the interaction between electric currents and magnetic fields. Understanding these relationships lays the groundwork for more advanced theories, such as quantum magnetism, where the behavior of particles at atomic scales becomes essential.
Key concepts include:
- Magnetic Dipoles: Basic units of magnetism that determine how magnetic fields behave in various materials.
- Curie Temperature: The temperature at which a material loses its permanent magnetic properties, critical in the study of ferromagnetic materials.
Visual Aids and Infographics
Using visual aids is effective in simplifying complex information related to magnetism. Diagrams of magnetic field lines, charts depicting the magnetic properties of materials, and infographics that summarize the applications of magnetism can enhance comprehension.
These resources engage students and professionals alike, making it easier to grasp how theoretical principles apply in real-world scenarios. Graphical representations can help illustrate concepts such as hysteresis in magnetic materials or the arrangement of magnetic domains within a ferromagnet.
The End
The discourse surrounding magnetism is rich and varied, touching on classical physics while intersecting with modern technological advancements. As new materials and applications emerge, understanding magnetism becomes increasingly vital for innovation and exploration in both science and everyday life.
Understanding Magnetism
Magnetism is a fundamental force of nature that influences a vast array of physical phenomena. In this article, we explore its principles and applications, emphasizing the importance of understanding magnetism. Learning about this topic opens doors to innovations in science and technology. Not only does it enhance our grasp of classical physics, but it also helps unravel the complexities of quantum mechanics.
Understanding magnetism grants insight into how materials behave, which is vital for fields like electronics, transportation, and medicine. Knowledge in this area can lead to advancements in magnetic materials and their applications, providing benefits that range from improved data storage to life-saving medical imaging technologies.
Definition and Basics
Magnetism can be described as a force that arises from the motion of electric charges. It is a property of materials that can be observed when they interact with magnetic fields. Magnetism is one of the four fundamental forces of nature, alongside electric force, gravitational force, and nuclear force. Understanding its definition is crucial for grasping the principles that govern various magnetic phenomena.
The Role of Electrons
Electrons play a central role in magnetism. They are negatively charged particles that, when in motion, create a magnetic field. In materials, the arrangement of electrons in atoms determines the material's magnetic properties. For instance, in ferromagnetic substances, unpaired electrons align in the same direction, generating a strong magnetic field. This demonstrates how electron behavior is fundamental to understanding magnetic effects.
Types of Magnetism
Magnetism can be classified into several categories, each with unique characteristics:
Diamagnetism
Diamagnetism is a form of magnetism that occurs in all materials to some extent. It results from the induced magnetic fields that oppose applied magnetic fields. The key characteristic of diamagnetism is that the material creates a weak magnetic field in the opposite direction of an applied field, leading to a repulsion effect. This property is beneficial due to its non-invasive nature. However, its strength is very weak compared to other forms of magnetism, limiting its practical applications.
Paramagnetism
Paramagnetism is observed in materials that possess unpaired electrons. When exposed to an external magnetic field, these materials become weakly magnetized in the direction of the applied field. The unique feature of paramagnetism is that the magnetization is temporary and disappears when the external field is removed. Paramagnetic materials are widely used in various applications, such as magnetic sensors, due to their responsiveness, but their weakness compared to ferromagnetic materials can be a disadvantage.
Ferromagnetism
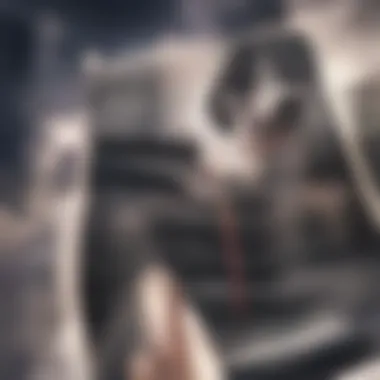
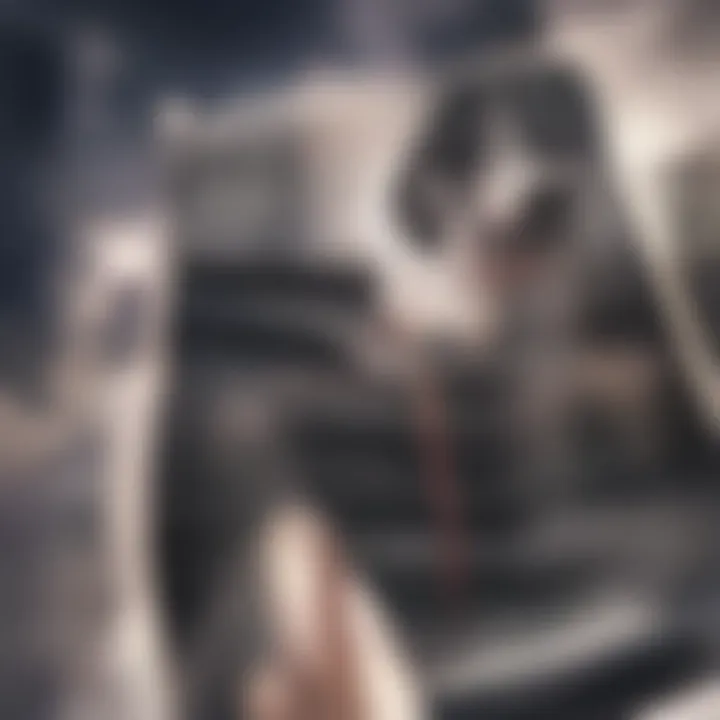
Ferromagnetism is a strong form of magnetism found in materials like iron, cobalt, and nickel. The key characteristic of ferromagnetism is the alignment of magnetic moments even in the absence of an external field. This behavior results in a significant magnetic field and permanence after magnetization. Ferromagnetic materials are essential for many applications, including electric motors and transformers. However, they may lose their magnetic properties at high temperaturesโa situation known as the Curie temperature.
Antiferromagnetism
Antiferromagnetism occurs in certain materials where adjacent magnetic moments align oppositely, canceling each other out. The key characteristic involves the magnetic moments having equal magnitude but opposite directions. This type of magnetism is significant because it helps us understand materials that exhibit unique electrical properties. While antiferromagnetic materials are often less useful in real-world applications due to their cancellation effects, they play a critical role in the study of complex magnetic systems.
Ferrimagnetism
Ferrimagnetism is similar to antiferromagnetism, but the magnetic moments are unequal, resulting in a net magnetic moment. The key characteristic is that these materials exhibit both strong magnetic properties and the complexity of interactions. Ferrimagnetic materials are commonly used in magnetic storage media and in the design of magnetic devices. However, like other magnetic types, they face challenges at varying temperatures, which can affect their performance.
Historical Perspective
The historical perspective on magnetism plays a crucial role in understanding its multifaceted nature. By examining past discoveries, we can appreciate how early scientific concepts paved the way for modern applications. This foundation is beneficial for contextualizing current research and future developments in the field. Understanding the evolution of magnetism helps us recognize the significance of key figures and their contributions, which have shaped the technological landscape today. This section highlights landmark moments and crucial individuals in the history of magnetism, providing insight into their impact on contemporary scientific practices and theories.
Early Discoveries
Early discoveries in magnetism inspired generations of scientists. Ancient civilizations were aware of magnetic materials, such as lodestones, which were naturally magnetized pieces of iron ore. The Greeks and Chinese noted their properties, but it wasn't until the Renaissance that serious investigation began.
Over time, scholars started to document experiments and observations. William Gilbert, in particular, is often regarded as the father of magnetism, thanks to his systematic approach. His work laid the groundwork for future experiments and theories. Understanding these early experiments helps us appreciate the simplicity yet complexity of magnetic forces.
Contributions of Key Figures
William Gilbert
William Gilbert is a pivotal figure in the history of magnetism. He published "De Magnete" in 1600, marking one of the first comprehensive studies of magnetism. Gilbert's observations on Earth's magnetism were revolutionary, challenging existing theories and establishing a systematic methodology for experimental science. His focus on empirical evidence demonstrates a critical shift from speculative philosophy to a more scientific approach.
Key characteristic: Gilbert's insistence on rigorous experimentation is a significant reason for his enduring influence. This method allowed future scientists to build upon his findings.
Unique feature: Gilbert's distinction between magnetic and electric forces highlighted the complexity of physical interactions, underpinning the universality of electromagnetic theory. While he faced challenges due to the limitations of technology in his time, the insights gained have been foundational in physics.
Hans Christian รrsted
Hans Christian รrsted made momentous contributions to magnetism with his discovery in 1820 that electric currents create magnetic fields. This revolutionized the understanding of electromagnetism. รrsted's work showcased an interconnection between electricity and magnetism, leading to advancements in both fields.
Key characteristic: รrsted's interdisciplinary approach was crucial in the integration of scientific concepts. His work spurred significant research into electromagnetic phenomena, helping bridge the gap between physics and engineering.
Unique feature: รrsted's insights initiated the field of electrodynamics, impacting how we understand energy flow and magnetic behavior. However, he faced the challenge of limited communication and acceptance within the scientific community at the time.
James Clerk Maxwell
James Clerk Maxwell's contributions represent a higher synthesis of existing knowledge on electromagnetic phenomena. His formulation of Maxwell's equations in the mid-19th century unified electricity, magnetism, and optics, providing a comprehensive theoretical framework for understanding electromagnetic fields.
Key characteristic: The elegance and precision of Maxwell's equations solidified Maxwell's status as a leading figure in physics, influencing various scientific fields beyond magnetism.
Unique feature: Maxwell's equations are notable for their predictive power and have been essential in the development of technologies like radio, television, and radar. They exemplify how theoretical advancements can drive meaningful technological innovations.
Historical exploration of magnetism not only highlights past discoveries but also illustrates the continual advancement of scientific knowledge that shapes our technological reality today.
In summary, the contributions of these key figures frame our understanding of magnetism, illustrating the trajectory of scientific thought through history. Their efforts not only influenced the course of physics but also opened pathways for future discoveries and applications.
The Science of Magnetism
Understanding the science of magnetism is essential for grasping how magnetic phenomena influence a broad spectrum of disciplines. This section discusses foundational concepts, emphasizing how magnetic fields and forces operate, their mathematical formalization through Maxwell's equations, and crucial quantum aspects. The impact of magnetism is vast, affecting our technology, daily life, and the very frameworks of modern physics. Magnetic principles are not merely academic; they provide essential insights into practical applications such as electronics, communication, and medical imaging.
Magnetic Fields and Forces
Magnetic fields arise from the movement of electric charge and create forces that influence objects within their reach. The intensity and direction of a magnetic field are crucial determinants of its effect on various materials. Magnets can attract or repel based on their orientation, leading to practical applications in motors, generators, and magnetic storage devices.
The behavior of magnetic fields follows the laws of physics, primarily governed by Lorentz force, which indicates that charged particles experience a force when they move through a magnetic field. Understanding these interactions is vital for electrical engineering and physics.
Maxwell's Equations
Maxwell's equations are fundamental to the field of magnetism, describing how electric and magnetic fields propagate and interact. These four equations comprehensively depict the behavior of electrically charged particles and allow us to understand electromagnetism's principles in detail.
- Gauss's Law for Magnetism states that the magnetic field lines neither begin nor end but form closed loops or infinite extend; this indicates that magnetic monopoles do not exist.
- Faraday's Law of Induction explains how a changing magnetic field can induce an electric current in a conductor.
- Ampรจre's Law relates magnetic fields to the currents producing them.
- Maxwell's Addition introduces displacement current, affecting the formulation of electromagnetic waves.
Understanding Maxwell's equations is crucial, as they provide the theoretical foundation for technologies such as radio transmission and electrical circuits.
Quantum Aspects of Magnetism

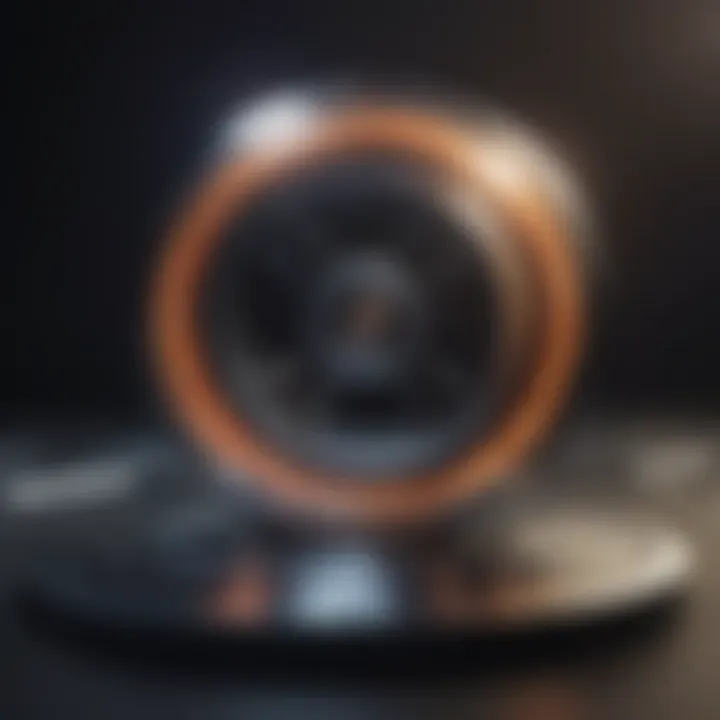
Magnetism also aligns with quantum physics principles, revealing phenomena not apparent in classical physics. This quantum nature is relevant for several technological advancements.
Spin and Magnetic Moments
At the quantum level, the concept of spin in particles, which can be understood as an intrinsic form of angular momentum, is pivotal for describing magnetic moments. The magnetic moment quantifies how a particle interacts with an external magnetic field. Electrons have a property called spin that can be thought of as spinning on their axes, leading to the emergence of their magnetic moment.
This element is significant because it is responsible for many magnetic behaviors seen in materials. In certain materials, electron spins can align, resulting in phenomena such as ferromagnetism. Its unique characteristic, where individual spins collectively influence the magnetic properties of a material, makes it a beneficial choice for studying magnetism.
However, managing these spins for practical applications can be complex and does present challenges such as thermal fluctuations affecting alignment. This aspect is critical when considering the stability of magnetic materials in various applications.
Quantum Magnetism Phenomena
Quantum magnetism phenomena explore magnetism in terms of quantum mechanics. Here, different interactions at the quantum level yield fascinating behaviors, such as antiferromagnetism and quantum entanglement.
One key characteristic of quantum magnetism is its non-classical behavior. This includes phenomena like spin-liquid states, where spins are disordered even at absolute zero temperature. This property has implications in advanced materials science and quantum computing. It demonstrates the potential for creating systems that can outperform classical systems due to enhanced performance in computations.
The advantages of studying quantum magnetism are significant. It allows researchers to develop new materials, including superconductors. But, understanding these phenomena is complex; the interactions can be unpredictable due to the intricate nature of quantum states, presenting challenges in practical applications.
"The behavior of magnetic fields and the principles governing them are not just theoretical constructs; they lie at the heart of technologies that shape our modern world."
In sum, the science of magnetism plays a crucial role in understanding fundamental physical principles that underlie both natural phenomena and technological advancements.
Magnetic Materials
Magnetic materials form the backbone of our understanding and application of magnetism. Their properties dictate how magnetic fields interact with matter, influencing numerous technolgical advancements. By classifying these materials, we can focus on their specific characteristics and potentials. This section will explore the various classifications of magnetic materials and their significance in both industrial applications and everyday usage.
Classification of Magnetic Materials
Soft Magnets
Soft magnets are characterized by their ability to be magnetized quickly yet can also be easily demagnetized. This makes them particularly useful in applications where the magnetic field needs to change frequently, such as in transformers and inductors. The key characteristic of soft magnets is their low coercivity, allowing for rapid magnetization and demagnetization.
One popular example of a soft magnet is silicon steel. This material captures magnetic fields efficiently, providing a strong magnetic response with minimal energy loss. The unique feature of soft magnets is their high permeability, making them effective in guiding magnetic fields. Therefore, their advantages include efficiency and versatility, which contribute greatly to electrical engineering and energy transmission processes.
Hard Magnets
Hard magnets are known for their strong magnetic properties and the ability to maintain a stable magnetic field without external influences. The key characteristic here is their high coercivity. This means that once they are magnetized, they remain magnetized even when the external magnetic field is removed.
An example of this is the neodymium magnet, which exhibits remarkable strength and reliability. The unique feature of hard magnets is their sturdy magnetic retention, which translates into enduring performance in various applications like magnetic locks and motors. The disadvantages, however, include brittleness and higher costs associated with production, which may limit their use in some situations.
Permanent Magnets
Permanent magnets are a distinct category that emphasizes their ability to retain magnetism indefinitely once magnetized. They do not require an external power source to maintain their magnetic field. The key characteristic of permanent magnets is their consistent performance in various environments, making them reliable in diverse applications.
Materials such as ceramic and ferrite are common in permanent magnets. Their unique feature is their durability, allowing them to thrive in harsh conditions without losing their effectiveness. Among their advantages are their cost-effectiveness and wide availability. However, they may not provide the same strength levels as hard magnets, resulting in limitations in high-efficiency applications.
Applications in Technology
Magnetic materials play a role in many modern technologies. They are utilized in:
- Data storage devices such as hard drives and magnetic tapes
- Sensors that detect changes in magnetic fields
- Electric motors that drive various machinery
- Healthcare equipment like MRI machines
- Transportation systems, including maglev trains and electric vehicle motors
These applications highlight the integral role of magnetic materials in advancing technology, impacting industries and personal lives significantly. Each class of magnetic material has its optimal use, contributing effectively to the overall functionality of modern devices.
Applications of Magnetism
The applications of magnetism permeate various fields and are fundamental to modern technology. Understanding how magnetism is applied helps explain its significance in everyday life as well as in advanced scientific research. The exploration of magnetism yields tangible benefits, such as enhanced performance in electronics, medical technologies, and transportation systems. Each specific application showcases the versatility of magnetism and its ongoing evolution in response to contemporary challenges.
Electronics and Computing
Data Storage Solutions
Data storage solutions, like hard disk drives and magnetic tapes, use magnetism to read and write data. Their ability to retain vast amounts of information is crucial for both individuals and businesses. One key characteristic of these solutions is their long-lasting data retention. This makes them a reliable choice in a world where data loss can be catastrophic. The unique feature of hard disk drives is their spinning magnetic platters that align to store information. However, while they are cost-effective, they might not measure up to the speed of flash storage in some contexts.
Magnetic Sensors
Magnetic sensors play a vital role in detecting changes in magnetic fields, which can translate into various applications such as position sensing and speed detection. Their essential characteristic is sensitivity to magnetic fields, allowing them to operate efficiently in demanding environments. Magnetic sensors are popular choices due to their robustness and reliability in devices such as smartphones and automotive systems. Yet, they can be prone to interference from other electronic devices, which is a consideration when deploying them in complex systems.
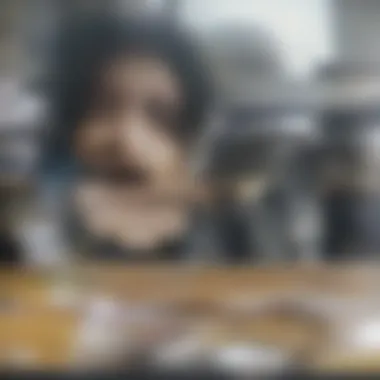
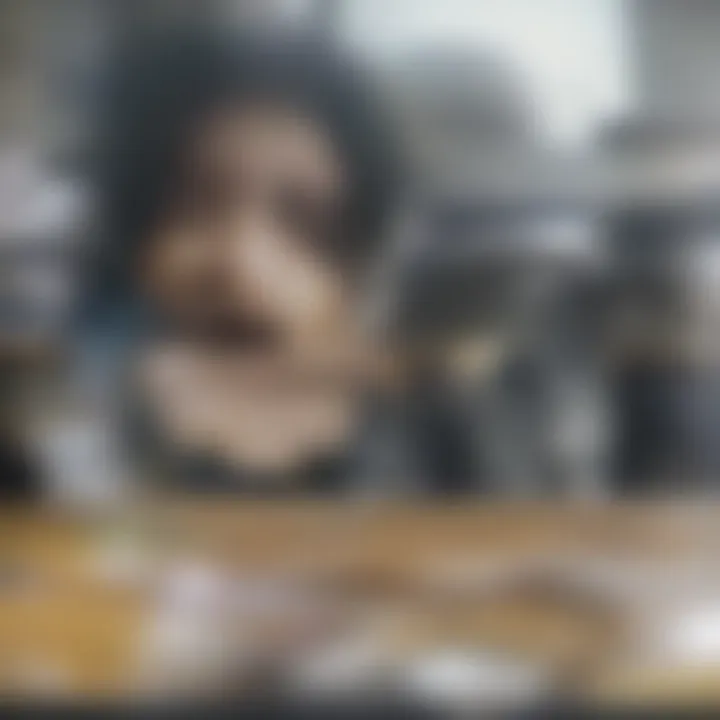
Medical Applications
Magnetic Resonance Imaging
Magnetic Resonance Imaging (MRI) utilizes powerful magnets and radio waves to create detailed images of organs and tissues. This technique is significant because it allows for non-invasive diagnosis, which can greatly enhance patient care. The fundamental characteristic of MRI is its ability to provide high-resolution images without using ionizing radiation. It is a beneficial choice for medical professionals, as it helps identify conditions that are hard to detect through other means. One downside is that it is relatively costly and requires specialized facilities.
Therapeutic Uses of Magnetism
Therapeutic uses of magnetism, ranging from magnet therapy to Localized Magnetic Field Therapy, involve applying magnetic fields to promote healing and reduce pain. This aspect of magnetism has gained attention in alternative medicine because of its potential benefits for pain management. A notable characteristic of these therapies is their simplicity and non-invasive nature, making them appealing for many patients. However, the scientific community is still exploring the efficacy of these treatments, and skepticism exists regarding their long-term benefits.
Transportation Technologies
Maglev Trains
Maglev trains utilize magnetic levitation to achieve high-speed travel with minimal friction. This advanced type of transportation has significant implications for reducing travel time and increasing efficiency. The primary characteristic of Maglev trains is their unique technology that allows them to glide above tracks using magnetic forces. This offers advantages such as reduced wear and maintenance costs compared to conventional trains. Nevertheless, the infrastructure required for Maglev systems can be extensive and costly to implement.
Electric Vehicles
Electric vehicles (EVs) employ magnetic components in various ways, such as in motors and regenerative braking systems. Their role in reducing carbon emissions aligns well with global sustainability goals. The key feature of electric vehicles is their efficiency and capability to provide instant torque, translating to enhanced driving experiences. They are popular because they contribute to a cleaner environment. However, the dependence on external charging infrastructures and battery limitations remain challenges that need ongoing attention.
Current Research Trends
Research in magnetism is at a pivotal turn in its evolution, bridging theoretical concepts with practical applications. This section will interrogate the significance of these current trends and their implications for various disciplines. Key areas such as emerging technologies and interdisciplinary research highlight the potential of magnetism in advancing our understanding of both fundamental and applied sciences. These trends not only provide insight into material science but also influence the development of future technologies that will shape our lives.
Emerging Technologies
Spintronics
Spintronics is a field that builds upon traditional electronics by utilizing the intrinsic spin of electrons alongside their charge. One notable aspect of spintronics is its ability to create devices that are faster and more energy-efficient. The key characteristic of spintronics is its reliance on the spin states, which can be manipulated to represent binary data. This feature makes it a promising option for next-generation computing and data storage systems.
Challenges remain, however. The complexity of creating and maintaining stable spin states in materials can be difficult. Moreover, there is significant research needed to implement these technologies in mainstream applications. Nonetheless, the advent of spintronics represents a significant shift towards more advanced, efficient technologies.
Magnetic Nanomaterials
Magnetic nanomaterials are materials engineered at the nanoscale to exhibit unique magnetic properties. These materials can be used in a variety of applications from biomedical imaging to high-density data storage. A standout characteristic of magnetic nanomaterials is their high surface area to volume ratio, which enhances their magnetic response. This feature contributes to their increasing popularity in various technological fields.
The advantages of using magnetic nanomaterials include their ability to offer improved performance and versatility in applications. However, the synthesis and stability of these materials pose challenges. Additionally, their long-term effects on health and the environment require careful analysis. Despite these concerns, the potential benefits position magnetic nanomaterials as a key area of research moving forward.
Interdisciplinary Research
Magnetism in Biophysics
The study of magnetism in biophysics explores how magnetic fields interact with biological systems. This interdisciplinary research can shed light on critical processes in living organisms, such as cellular communication and molecular functions. The key characteristic of this field is its ability to integrate principles of magnetism with biological sciences, making it valuable for a deeper understanding of life at a molecular level.
One unique feature of magnetism in biophysics is its potential application in medical therapies and drug delivery systems. Such applications underscore the need for ongoing research to navigate the complexities of these interactions. However, the potential breach into untested biological territories can raise ethical and safety questions that must be addressed as research progresses.
Astrophysical Magnetism
Astrophysical magnetism covers the magnetic phenomena that occur on cosmic scales, influencing everything from stellar formation to the dynamics of galaxies. This area of research is particularly crucial for understanding the universe's behavior and structure. The key characteristic of astrophysical magnetism is the role that magnetic fields play in the evolution of the cosmos. Research in this area has considerable relevance to theoretical physics and cosmology.
A unique aspect of astrophysical magnetism is its potential to unify various astrophysical processes under the influence of magnetic fields, leading to new insights and models. However, observing and measuring these phenomena can be difficult, often requiring advanced techniques and technologies. The challenges involved in this research are significant, but breakthroughs in this area can lead to transformative insights into the universe.
Challenges and Future Directions
Addressing the challenges and future directions in the field of magnetism is critical for further advancement in both science and technology. This section highlights vital topics that must be considered for maximizing the potential applications of magnetism. From the sustainability of magnetic materials to the anticipated evolution in their usage, understanding these challenges lays the groundwork for effective approaches in overcoming them. Each element discussed not only emphasizes the importance of responsible practices but also suggests directions for innovative advancements in magnetic technologies.
Sustainability in Magnetic Material Production
Sustainable production of magnetic materials has become a pressing concern in recent years. With the intensifying demand for advanced materials in various industries, environmental considerations cannot be overlooked. The extraction and processing of rare earth elements and other critical materials often result in significant ecological impacts.
Efforts to improve sustainability focus on several key aspects:
- Recycling: Developing methods to recycle rare earth materials from end-of-life electronic devices can significantly reduce the need for new mining activities.
- Alternative Materials: Research is directed towards alternative materials that can replace traditional rare earth magnets. This search can lead to more abundant and environmentally friendly resources.
- Energy Efficiency: Innovations in manufacturing processes can enhance energy efficiency, reducing carbon footprints associated with magnet production.
By prioritizing sustainability, the industry can not only minimize its ecological impact but also secure a more stable supply chain for essential materials used in various magnetic applications.
The Future of Magnetic Applications
The future of magnetic applications is poised to expand markedly across multiple domains. As technologies develop, the use of magnetic principles is anticipated to transcend current boundaries. Here are some areas where future applications could play a transformative role:
- Electronics: The evolution of electronics will likely see closer integration of magnetism with quantum computing. Spintronics, for instance, holds potential for enhancing data processing speeds while reducing energy consumption.
- Healthcare: Advances in magnetic resonance imaging and other medical technologies can provide more precise diagnostics. Furthermore, specific targeted therapies using magnetic fields are being explored.
- Transportation: Magnetic technologies in transportation may evolve to include more widespread use of maglev trains, potentially redefining public transport efficiency and speed.
Significant innovations are on the horizon, keeping current challenges in mind. Investing in research, coupled with a commitment to sustainability, can unlock promising new applications in diverse fields.
In summary, facing the challenges in sustainability and the evolving landscape of magnetic applications will determine the trajectory of this field. Stakeholders in industry and academia must collaborate to ensure that advancements are not only technically feasible but also environmentally responsible.