Exploring Space-Time Ripple: The Universe's Fabric
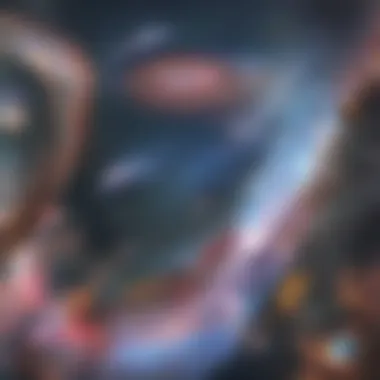
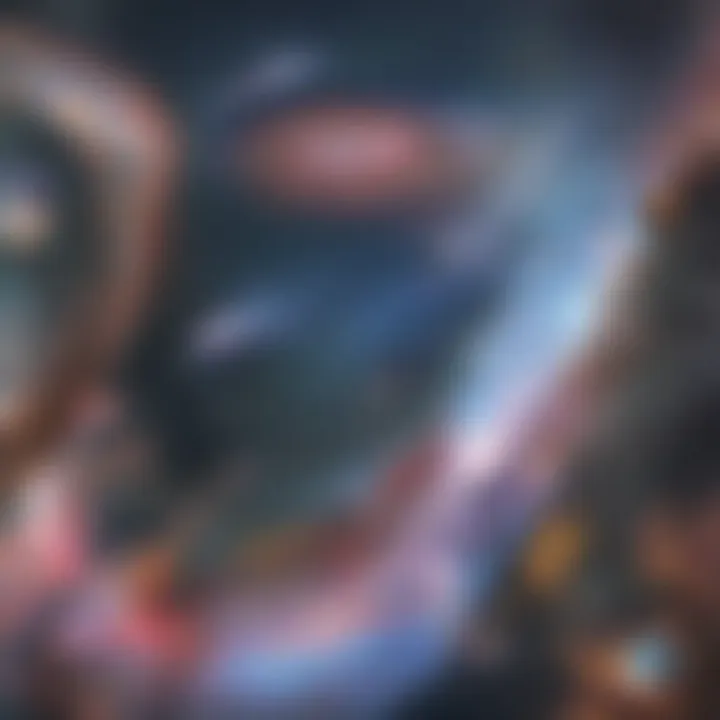
Intro
The concept of space-time ripples introduces a fascinating dimension to our understanding of the universe. These ripples, also known as gravitational waves, arise from some of the most violent and energetic processes in the cosmos. The study of these phenomena not only enriches the field of astrophysics but also intersects with mathematical and technological advancements. As researchers continue to explore these ripples, they uncover insights that challenge and expand our current models of the universe.
Key Research Findings
Overview of Recent Discoveries
In recent years, the detection of gravitational waves has shifted the paradigms within astrophysics. The first direct detection by the LIGO observatory in 2015 confirmed a long-held prediction from Einstein's general relativity. Since then, international collaborations like VIRGO and KAGRA have contributed to this momentum. These discoveries have led to the identification of events such as the merger of black holes and neutron stars.
Significance of Findings in the Field
The implications of these findings are profound. Gravitational waves offer a new means of observing the universe, complementing traditional electromagnetic observations. This multimodal approach allows scientists to gather more comprehensive information about cosmic events. For example, the observation of gravitational waves from neutron star mergers provided critical insights into heavy element synthesis, such as gold and platinum, further enriching our understanding of cosmic chemistry.
Breakdown of Complex Concepts
Simplification of Advanced Theories
Understanding gravitational waves requires familiarity with complex theories. At its core, Einstein's general relativity posits that massive objects warp space-time, creating waves as they move. Simplifying this concept involves viewing space-time not as an empty backdrop but as a dynamic fabric linked to mass and energy. When significant masses, like black holes, collide, they disturb this fabric, leading to the creation of space-time ripples.
Visual Aids and Infographics
Visual representations play a vital role in grasping the intricacies of gravitational waves. Infographics can illustrate how these waves propagate through the universe and how they are detected by instruments like LIGO and VIRGO. Specifically, diagrams showing waveforms can help delineate the differences between various types of gravitational wave signals, such as those from binary black hole mergers versus those from neutron star collisions.
"Gravitational waves are not just ripples in space-time; they are the melody of the universe, revealing secrets of cosmic events previously shrouded in silence."
Prolusion to Space-Time and its Importance
Space-time is a fundamental concept in the understanding of physics and the universe. It combines the three dimensions of space with one dimension of time into a single four-dimensional continuum. This integration has important implications for how we perceive movement, gravity, and the overall structure of the cosmos.
In this section, we aim to explore the significance of space-time in a scientific narrative. Understanding space-time is essential because it sets the framework for many physical phenomena. This includes not only the gravitational waves discussed later, but also the behavior of light, the expansion of the universe, and the nature of black holes.
Defining Space-Time
Space-time can be defined succinctly as the model that merges the dimensions of space with time. In simpler terms, it is the fabric within which all objects exist and all events occur. Maxwell's equations of electromagnetism and Einsteinโs theory of relativity are key foundations for defining this concept. When dramatic events occur in space, such as the collision of massive astronomical bodies, they create ripples. These ripples propagate through space-time, much like waves on a pond.
Moreover, the intertwining of time with space challenges our intuitive understanding of both concepts. Traditionally, space and time seemed separate, but experimental evidence suggests they are linked.
The Role of Gravity in Space-Time
Gravity is not merely a force; it's a curvature of space-time caused by mass. According to general relativity, massive objects like stars and planets distort the space-time around them. This curvature is what we experience as gravity. For example, the Earth circles the sun not just because it is drawn to it, but because the sun stretches the fabric of space-time in a way that guides Earth's path. This nuanced understanding of gravity reveals much about how celestial bodies move and interact.
Historical Background
The concept of space-time underwent transformation, particularly in the early 20th century. Before Einstein, Isaac Newton described gravity as a force between masses. However, Einstein's theories revolutionized how scientists think about space and time. His general theory of relativity, published in 1915, proposed that objects in space-time influence one another through their masses, leading to an integrated view of the universe. The ramifications of these ideas impacted not only theoretical physics but also practical applications in areas such as GPS technology.
Gravitational Waves: The Ripple in Space-Time
Gravitational waves represent a significant area of study in both theoretical and experimental physics. These ripples in space-time emerge from the acceleration of massive bodies, such as merging black holes or neutron stars. Understanding these waves not only enhances our grasp of the universe but also adds to our knowledge of fundamental physics. The discovery and detection of these waves has opened a new window into observing the cosmos.
Discovery of Gravitational Waves
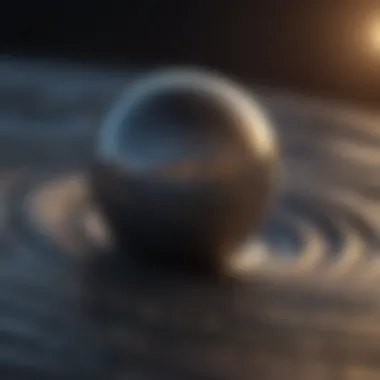
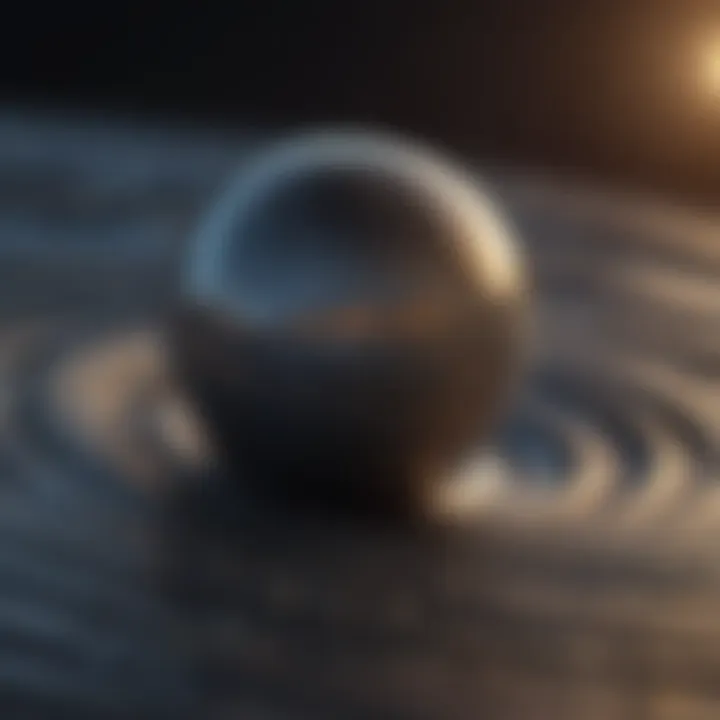
The concept of gravitational waves was predicted by Albert Einstein in 1915 as part of his general theory of relativity. In this theory, mass and energy can distort space-time, leading to the creation of waves when massive objects accelerate.
However, it wasn't until 2015 that the Laser Interferometer Gravitational-Wave Observatory (LIGO) made a groundbreaking detection of these waves. This event confirmed Einstein's predictions and marked a milestone in astrophysics. Researchers detected waves resulting from the collision of two black holes, demonstrating how such events could produce detectable signals. This detection opened avenues for understanding various cosmic phenomena with unprecedented precision.
Detecting Gravitational Waves
Gravitational waves are incredibly subtle and require sensitive equipment to detect. LIGO and the Virgo Observatory are two key facilities dedicated to this task.
Laser Interferometer Gravitational-Wave Observatory (LIGO)
LIGO is a pioneering gravitational wave observatory located in the United States. It uses laser interferometry to measure tiny changes in distance caused by passing gravitational waves. The observatory consists of two long arms that form an "L" shape, each stretching four kilometers. When gravitational waves pass through, they cause minute changes in the lengths of these arms.
A major characteristic of LIGO is its sensitivity. It can detect waves with amplitude changes as small as one-thousandth the diameter of a proton. This high sensitivity makes LIGO an essential tool for cosmological research.
One unique feature of LIGO is its capability to operate in an environment where it can filter out noise caused by terrestrial vibrations. This allows for a clearer signal when analyzing gravitational waves. However, LIGO faces challenges in achieving this sensitivity, as environmental factors can still interfere with its measurements.
Virgo Observatory
Virgo is a European gravitational wave detector situated near Pisa, Italy. It complements LIGO's work by adding a third observatory in Europe, enhancing the detection of gravitational waves. Its design resembles that of LIGO, with a rectangular shape and long arms, measuring three kilometers each.
A key characteristic of the Virgo Observatory is its advanced technology. It employs similar laser interferometry techniques as LIGO but incorporates different design elements to enhance sensitivity and reduce noise levels. This allows Virgo to detect gravitational waves more effectively in certain frequency ranges.
The unique feature of Virgo is its ability to localize sources of gravitational waves better than LIGO alone. By collaborating with LIGO, Virgo can provide more precise information on the direction and origin of detected waves. Despite these advantages, Virgo still has limitations in terms of sensitivity compared to LIGO, which can affect its ability to detect weaker signals.
Understanding the Nature of the Waves
Gravitational waves carry information about their origins and the nature of gravity. They vary in amplitude and frequency, providing insights into the events that created them. By studying these waves, scientists can explore extreme cosmic events and further verify aspects of general relativity.
The ongoing advancements in gravitational wave astronomy not only promise new discoveries but also challenge existing frameworks in physics. The implications extend far beyond astrophysics, impacting our understanding of the universe's structure, evolution, and fundamental laws that govern it.
The Physics Behind Space-Time Ripples
Understanding the physics behind space-time ripples is fundamental to comprehending how the universe operates on both microscopic and macroscopic levels. The interactions between mass and the curvature of space-time not only explain gravity but also illuminate the dynamics of cosmic events such as black holes and neutron star collisions. These ripples, often referred to as gravitational waves, are a direct output of Einstein's framework of relativity. Exploring this section will elucidate both theoretical and mathematical aspects that govern these profound phenomena.
Einstein's General Theory of Relativity
Einstein's General Theory of Relativity fundamentally changed our understanding of gravity. Prior to this, gravity was explained as a force acting at a distance. Instead, Einstein proposed that mass warps the space-time fabric and that this curvature affects the movement of objects. The significant characteristic of this theory is its reliance on the geometry of space. It provides a mathematical structure to describe how mass deforms space-time. This leads to the prediction of phenomena like black holes and gravitational waves, marking a pivotal shift in physics. Einstein's work implies that the universe is interconnected in a way previously unimagined. Understanding this interconnectedness is critical for research into space-time ripples.
Mathematical Modeling of Space-Time
Mathematical modeling provides the tools necessary to quantify and predict the phenomena arising from general relativity. It allows researchers to visualize complex interactions in the universe and draw significant conclusions from data.
Tensors and Geometry
Tensors form the backbone of mathematical models used in relativity. They are essential for describing the properties of matter in a curved space-time. The key characteristic of tensors is their ability to encapsulate data in multiple dimensions. This makes them a powerful choice for modeling intricate relationships in the universe.
One unique feature of tensors is their coordinate independence. This means that equations involving tensors retain their form regardless of the choice of coordinate system. Such a property ensures consistency and universality in modeling the effects of gravity. In the context of space-time ripples, tensors allow us to detail how mass influences the curvature of space-time and subsequently propagates gravitational waves. However, working with tensors can be complex and computationally intensive, which might deter some researchers.
Field Equations
Field equations are another vital aspect of the mathematical framework of general relativity. They describe how matter and energy influence the geometry of space-time. The Einstein Field Equations (EFE), specifically, are a set of ten interrelated differential equations that form the core of the theory.
The primary feature of field equations is their depth, enabling detailed analysis of the universe's structure. They are beneficial because they provide a connection between physical phenomena and mathematical descriptions. Their unique aspect lies in the fact that they can be utilized to predict not only the behavior of gravitational waves but also other cosmological dynamics. The complexity of field equations, however, can complicate their direct application. Solutions to these equations often require sophisticated computational techniques and significant computational power, creating a barrier for some studies.
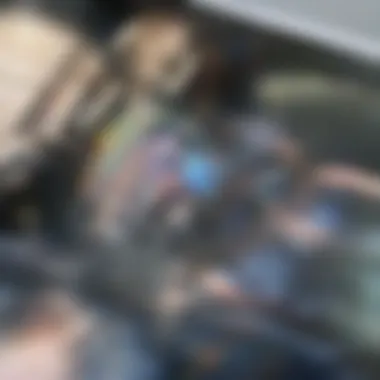
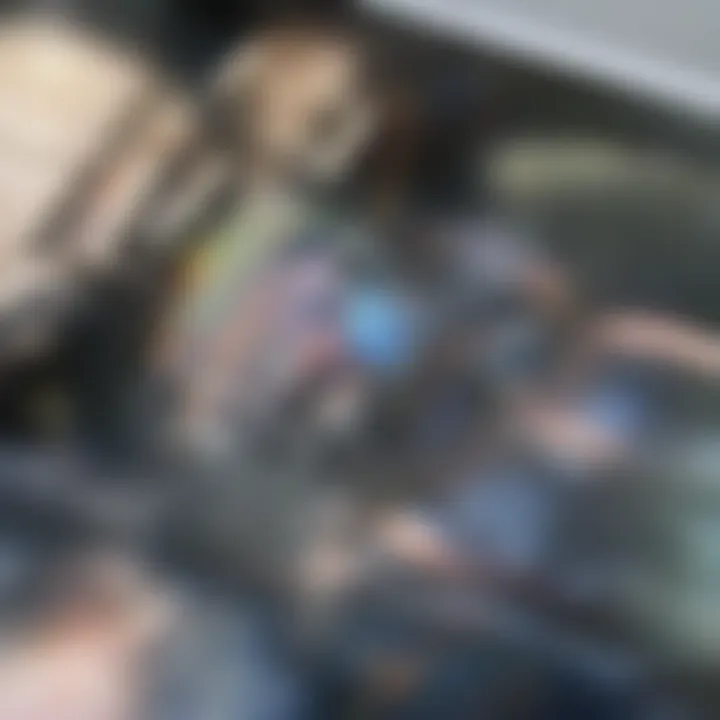
Energy and Momentum in Context
In the study of space-time ripples, understanding energy and momentum is crucial. These two concepts are linked intimately through the principles derived from Einstein's equations. Gravitational waves carry energy and momentum, which impacts the objects they encounter, causing measurable effects. For example, they can cause shifts in the positions of astronomical bodies and can potentially be detected through sensitive instruments.
This ongoing interaction underscores a deeper principle of conservation in physics whereby energy and momentum are preserved even amidst the complexities of space-time distortion. Understanding this context enriches the overall discourse surrounding space-time ripples and their far-reaching implications in cosmology and astrophysics.
Implications of Space-Time Ripples
Understanding the implications of space-time ripples is crucial for multiple fields within and beyond physics. Gravitational waves, as these ripples are often called, offer significant insights that extend our knowledge of the cosmos. They challenge existing theories and force scientists to rethink the structure of the universe.
Gravitational waves serve not merely as curiosities; they have real consequences in cosmology and astrophysics that help us better understand the universe's history and its ultimate fate. In addition, they lead to innovations in technology and computational methods, impacting society at large. Thus, the study of these waves is not limited to abstract concepts; it influences practical applications and interdisciplinary research.
Impacts on Cosmology
Gravitational waves significantly alter our approach to cosmology. Traditionally, cosmology has relied heavily on electromagnetic observationsโlight from distant galaxies and other celestial bodies. However, gravitational waves provide a different, complementary view of the universe. They allow scientists to observe events that are invisible through traditional methods, such as the merger of black holes or neutron stars.
This capability leads to a better understanding of the formation and evolution of cosmic structures. It supplies evidence for the existence of phenomena like black holes which do not emit light. As research progresses, gravitational waves could reshape our understanding of dark matter and dark energy, which are critical but not yet fully understood components of the universe.
Influence on Astrophysics
In the realm of astrophysics, gravitational waves have opened a new window for exploration. The ability to detect these waves allows researchers to study some of the most energetic events in the universe. These include supernovae, collisions between neutron stars, and the dynamics of black holes.
By analyzing the waves produced during these events, scientists can glean information about the mass, spin, and composition of the involved objects. This enriched understanding of astrophysical phenomena leads to better models of stellar evolution and the lifecycle of stars. Furthermore, it enables researchers to test the predictions of general relativity under extreme conditions.
Technological Innovations from Research
Gravitational wave research has spurred numerous technological innovations that reach far beyond the field of astrophysics. The pursuit of more sensitive detectors and advanced data analysis methods has led to progress in various areas.
Advanced detection technologies
Advanced detection technologies like Laser Interferometer Gravitational-Wave Observatory (LIGO) and Virgo Observatory are at the forefront. LIGO employs laser interferometry to measure minute changes in distance caused by passing gravitational waves. This capacity for high precision allows us to observe events billions of light-years away.
The unique feature of LIGO is its ability to detect waves from different angles, contributing to a better localization of events. However, the sophistication of this technology also presents challenges in environmental noise reduction and calibration. Overall, it offers a means to fundamentally change how we perceive cosmic events.
Data analysis algorithms
The development of data analysis algorithms is equally critical. These algorithms deal with large datasets captured by gravitational wave detectors. They help identify and classify waves, separating significant signals from noise. The key characteristic of these algorithms is their ability to learn and improve over time, thereby increasing their efficiency.
While they provide high accuracy, the complexity of these algorithms can lead to issues of interpretability. It is crucial to ensure their conclusions are reliable, impacting future research directions. In summary, innovations in detection and data analysis do not just advance our understanding of gravitational waves. They push technological boundaries that have broader applications within science and beyond.
Challenges in Space-Time Research
Research in space-time is not without its hurdles. Understanding the complex nature of space-time ripples requires tackling various challenges that affect both data collection and theoretical frameworks. Recognizing these challenges is vital, as they inform how scientists approach experiments and interpret results. Addressing these issues can lead to advancements in technology and a deeper understanding of the universe.
Environmental Interference
Environmental factors present considerable obstacles to space-time research. Instruments designed to detect gravitational waves are sensitive to minute changes, which can be caused by even minor vibrations or fluctuations in temperature. For example, seismic activity can generate noise that masks the signals researchers seek.
This interference comes from natural sources such as earthquakes and man-made sources such as traffic and construction. The Laser Interferometer Gravitational-Wave Observatory (LIGO) incorporates various measures to mitigate these issues. It operates in isolated locations and employs advanced filtering techniques. However, the challenge persists, emphasizing the need for continued innovation in detection technology.
Data Interpretation Issues
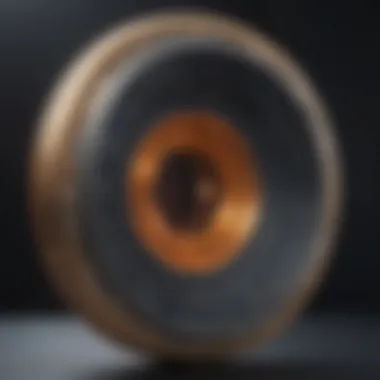
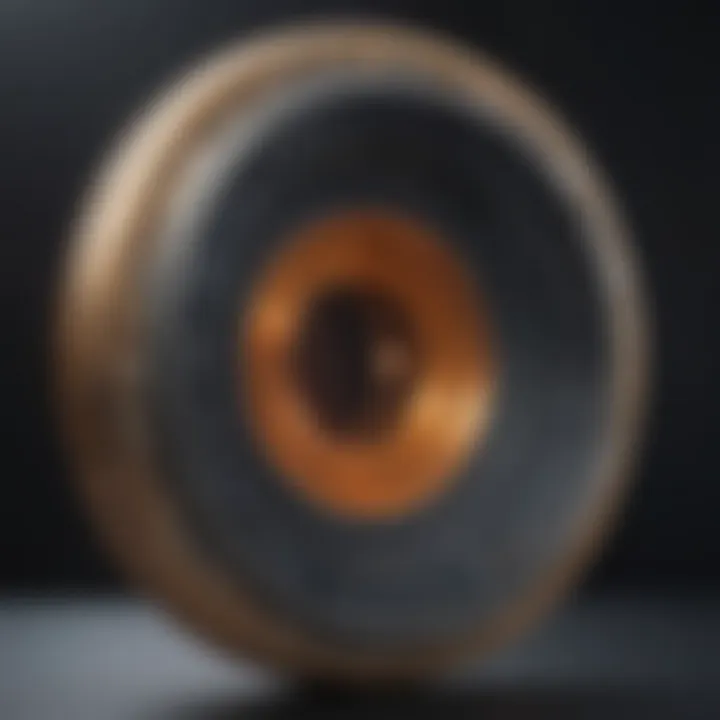
The complexity of the data retrieved from gravitational wave detectors creates its own set of challenges. Signals may be weak and easily misunderstood. Distinguishing genuine gravitational waves from noise is not straightforward.
Researchers often rely on algorithms and machine learning for data analysis. However, these methods can sometimes lead to incorrect assumptions. Statistical uncertainties complicate the interpretation of the data further. Ensuring that findings are accurate requires thorough testing and validation procedures, which can be time-consuming and resource-intensive.
Theoretical Limitations
The framework that manages gravitational waves still has theoretical gaps. Current models are based on Einstein's General Theory of Relativity, but there are aspects of gravitational phenomena that are not fully explained. For example, integrating quantum mechanics with general relativity poses significant mathematical challenges. These limitations constrain the capacity of scientists to make speculative predictions about the nature of space-time.
Additionally, simplifying assumptions made in modeling space-time can lead to incomplete or inaccurate representations of reality. As research evolves, scientists look toward more comprehensive theories that can reconcile existing frameworks. This reflects an ongoing quest to deepen our understanding without insisting on overly simplistic narratives.
Future advancements require an interdisciplinary approach that combines physics, mathematics, and computer science. Addressing these challenges will not only improve data collection methods but also refine the theoretical underpinnings of space-time research.
Future Directions in Space-Time Cosmology
Future directions in space-time cosmology are vital for pushing the boundaries of our current understanding of the universe. As research progresses and technology improves, many new possibilities emerge that could redefine our comprehension of space and time. This section explores upcoming observatories and projects, potential discoveries that lie ahead, and interdisciplinary research opportunities that could contribute to significant advancements in our field.
Upcoming Observatories and Projects
The next wave of observations in space-time research promises to illuminate countless aspects of the cosmos. Notable projects include the James Webb Space Telescope, which aims to observe distant celestial bodies and phenomena. Its enhanced capabilities will allow for deep insights into the formation of galaxies and the behavior of black holes.
Another significant project is the European Space Agency's LISA (Laser Interferometer Space Antenna), designed to detect gravitational waves from the cosmos. LISA will enhance our capacity to understand these waves and their sources, making it an essential tool in space-time research.
There are also many emerging initiatives in various stages of planning, such as the Square Kilometre Array, which is set to study the early universe and dark matter. By combining both advanced technology and large-scale collaboration, these observatories will provide unprecedented data and help refine existing theories.
Potential Discoveries on the Horizon
As new telescopes and detection methods become available, the potential discoveries in space-time are both vast and profound. One promising area of exploration involves understanding dark energy and its influence on the fabric of space-time. Insights gained could provide answers to pressing questions about the universe's expansion and ultimate fate.
Furthermore, the ongoing detection of gravitational waves opens pathways to new astrophysical phenomena. For instance, detecting waves from merging neutron stars can reveal more information about their internal structure and nuclear physics. Continued advancements in faint signal detection diets could lead to more groundbreaking discoveries.
"The future of space-time research is not just about finding answers, but also about exploring questions we have yet to imagine."
Interdisciplinary Research Opportunities
The study of space-time ripples connects various disciplines and encourages collaboration among scientists, mathematicians, and computer experts. Great advancements are possible when different fields merge to address complex questions about the universe. For example, mathematicians can refine methods of modeling space-time, while computer scientists can develop new algorithms to process large datasets from observatories.
There are also opportunities for partnerships with technology companies to enhance detection instruments and improve measurement precision. This synergy can lead to innovative solutions that drive further research and discovery.
In addition, educational institutions can promote interdisciplinary studies, fostering a new generation of researchers equipped with versatile skills. Such collaboration is essential for addressing new challenges and unlocking the mysteries of the universe.
In summary, future directions in space-time cosmology hold immense potential. By embracing advanced technology, collaborative research, and fresh methodologies, we can reshape our understanding of the universe and its intricate workings.
End
In concluding this exploration of space-time ripples, it is essential to underscore the multifaceted importance of this topic. The reactions to these cosmic phenomena significantly advanced physics and provided a framework for understanding the universe at a deeper level. Gravitational waves do not simply represent the echoes of violent cosmic events; they offer tangible evidence of the fundamental nature of space and time.
Summary of Key Findings
The examination throughout this article revealed several key findings:
- Nature of Gravitational Waves: These disturbances exemplify the dynamic nature of space-time as described by Einstein's general theory of relativity. They originate from significant astronomical events, such as colliding black holes and neutron stars.
- Detection Advances: Innovations in technology, particularly the development of instruments like LIGO and Virgo, have achieved incredible sensitivity, allowing scientists to capture minuscule changes in gravitational waves.
- Impacts on Cosmology and Astrophysics: The study of these waves has provided insights into the nature of the universe's expansion, formation of galaxies, and fundamental physics, deepening our knowledge about the cosmos.
The Broader Significance of Space-Time Ripples
The implications of space-time ripples extend beyond academic curiosity. Understanding these phenomena allows scientists to test prevailing physical theories and explore fundamental questions about existence. The knowledge gained influences multiple disciplines, merging physics, mathematics, and even technological innovation:
- Testing Theories: Each detection of gravitational waves serves as a real-world test of theoretical predictions that have long been assumed.
- Technological Innovation: The methodologies developed for detecting gravitational waves have repercussions for data analysis in various fields, paving the way for advancements in computational techniques and algorithms.
- Interdisciplinary Research Opportunities: The pursuit of understanding space-time ripples fosters collaboration between astrophysicists, mathematicians, and computer scientists, creating a rich tapestry of innovation and discovery that can lead to unforeseen breakthroughs.
The study of space-time ripples ultimately serves as a reminder of the intricate and interwoven nature of physics, technology, and our broader quest for knowledge about the universe.