The Enigma of Dark Matter: A Comprehensive Exploration
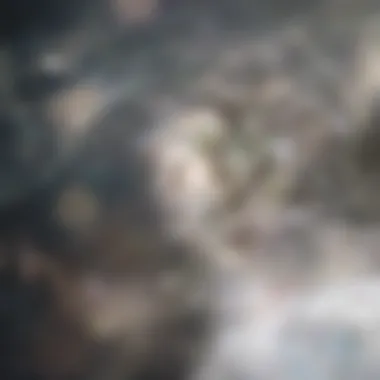
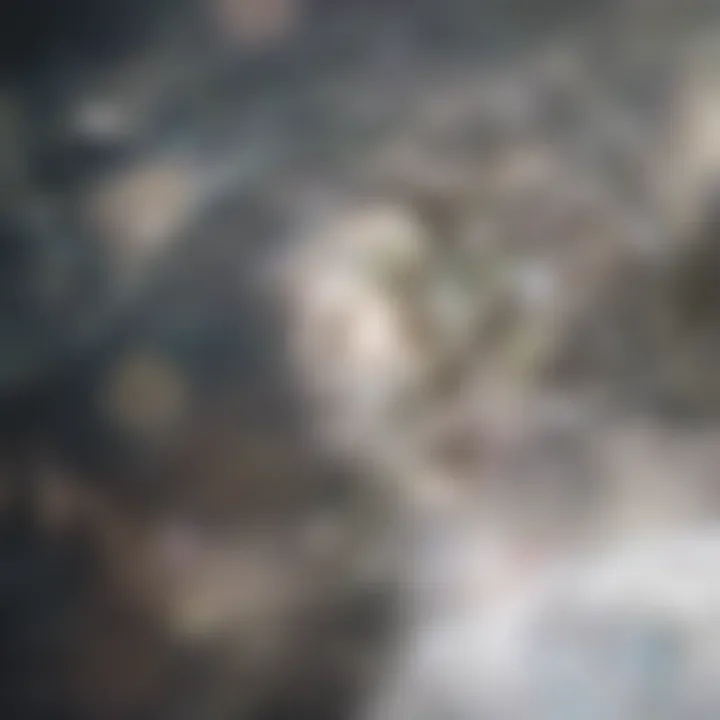
Intro
Dark matter represents one of the greatest conundrums in modern astrophysics. Constituting approximately 27% of the universe, its existence is inferred largely from gravitational effects on visible matter, radiation, and the large-scale structure of the universe. While it remains invisible and detectable only through its influence, recent advancements in observational techniques and theoretical frameworks are contributing to a better understanding of this elusive substance.
Researchers grapple with data that hint at the nature and distribution of dark matter. Its enigmatic characteristics pose fundamental questions about the universe's structure and evolution. This exploration is vital, as deciphering dark matter could unlock the mysteries surrounding galaxy formation and cosmic expansion.
Despite the complexities, the pursuit to unveil dark matter engages both seasoned scientists and curious minds alike. The journey into this obscure territory demands a blend of creativity, rigorous methodology, and a relentless quest for knowledge. This article aims to outline key research findings, simplify complex concepts, and detail the methodologies used in the search for dark matter.
Key Research Findings
Overview of Recent Discoveries
In the past few years, numerous discoveries have influenced our understanding of dark matter. Observations made by the Hubble Space Telescope and other advanced instruments have detected subtle gravitational effects that suggest the presence of dark matter in unexpected locations. For instance, studies involving gravitational lensing have revealed that dark matter behaves differently in various cosmic environments.
Prominent discoveries include:
- The Bullet Cluster: Observations of this collision between two galaxy clusters provided strong evidence for the existence of dark matter. The separation between visible matter and gravitational effects highlighted the role dark matter plays in cosmic interactions.
- Cosmic Microwave Background Radiation Studies: Observations from missions such as Planck have helped refine models of the universe, suggesting a higher density of dark matter than previously estimated.
- Galactic Rotation Curves: Studies of spiral galaxies reveal that the outer regions rotate at unexpectedly high speeds, indicating the presence of substantial unseen mass, attributed to dark matter.
Significance of Findings in the Field
These findings are pivotal in the field of cosmology. They help reshape our theoretical frameworks, compelling scientists to rethink the role of dark matter in the universe's evolution. The implications stretch beyond astrophysics, influencing fundamental physics with potential consequences for our understanding of the laws governing the universe.
Researchers aim to develop and refine models that accommodate the observed phenomena, leading to a deeper comprehension of dark matterโs nature and possibly its constituents.
"Understanding dark matter is crucial to grasping the overall dynamics of the cosmos."
Breakdown of Complex Concepts
Simplification of Advanced Theories
To understand dark matter, it is essential to break down complex theories into manageable parts. These theories often involve advanced physics concepts, including supersymmetry and string theory. Not all researchers agree on a single explanation for dark matter. Some propose WIMPs (Weakly Interacting Massive Particles) as candidates, while others consider axions and sterile neutrinos as potential components of dark matter.
Visual Aids and Infographics
Utilizing visuals aids can greatly enhance the understanding of dark matter. Infographics depicting:
- The distribution of dark matter in galactic structures.
- The gravitational effects of dark matter on light from distant galaxies.
- Diagrams illustrating the differences between visible matter and dark matter properties.
These tools assist in grasping otherwise abstract concepts, making the science behind dark matter more accessible.
By presenting this narrative, the article seeks not only to document the present state of research but also to inspire further inquiry into the complex, intriguing subject of dark matter.
Foreword to Dark Matter
Understanding dark matter is crucial to grasp the complexities of the universe we inhabit. Dark matter influences the formation and structure of galaxies, yet it remains one of the most elusive topics in modern astrophysics. As we embark upon this comprehensive exploration, it is essential to recognize the multifaceted nature of dark matter. It serves as a bridge connecting different domains of scientific inquiry, from theoretical physics to observational astronomy.
Contrary to what its name suggests, dark matter does not signify a lack of substance. Rather, its presence is inferred from gravitational interactions that affect visible matter, radiation, and the large-scale structure of the universe. The significance of dark matter is underscored by its estimated contribution of about 27% to the total mass-energy content of the universe.
The investigation into dark matter opens numerous pathways of inquiry. This encompasses developing explanatory models that illuminate the properties and potential candidates for dark matter, as well as employing innovative experimental techniques for its detection. The implications of this research extend beyond mere understanding; they intertwine with fundamental questions surrounding the fabric of reality itself.
Definition and Significance
Dark matter is defined as a type of matter that does not emit, absorb, or reflect electromagnetic radiation. This inability is what renders it invisible and detectable only through its gravitational effects. The gravity of dark matter influences the motion of galaxies and other cosmic structures, leading scientists to confidently state that it must exist despite its elusive nature. Its significance resides not only in its mass contribution but also in the role it plays in cosmic evolution and structure formation.
The understanding of dark matter is pivotal for a complete picture of the universe. It affects the cosmic microwave background radiation and structures galaxies and galaxy clusters. Understanding dark matter can lead to profound insights about the universeโs formation, evolution, and ultimate fate.
Historical Context
The concept of dark matter emerged from the observations of astronomers who noted inconsistencies in the mass of galaxies versus the visible matter they contained. In the 1930s, Swiss astronomer Fritz Zwicky studied the Coma cluster of galaxies and noted that the visible matter alone could not account for the observed gravitational binding of the cluster. His findings suggested the presence of unseen mass, which he termed "dunkle Materie" or dark matter.
The 1970s further solidified the dark matter hypothesis, largely through the work of Vera Rubin. Her studies of galaxy rotation curves revealed a discrepancy between the mass indicated by visible stars and the mass required to keep galaxies gravitationally bound. The common understanding began to shift from visible matter alone to a model including dark matter.
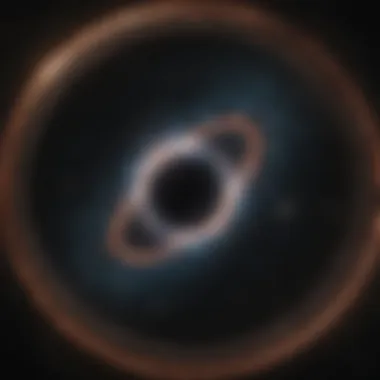
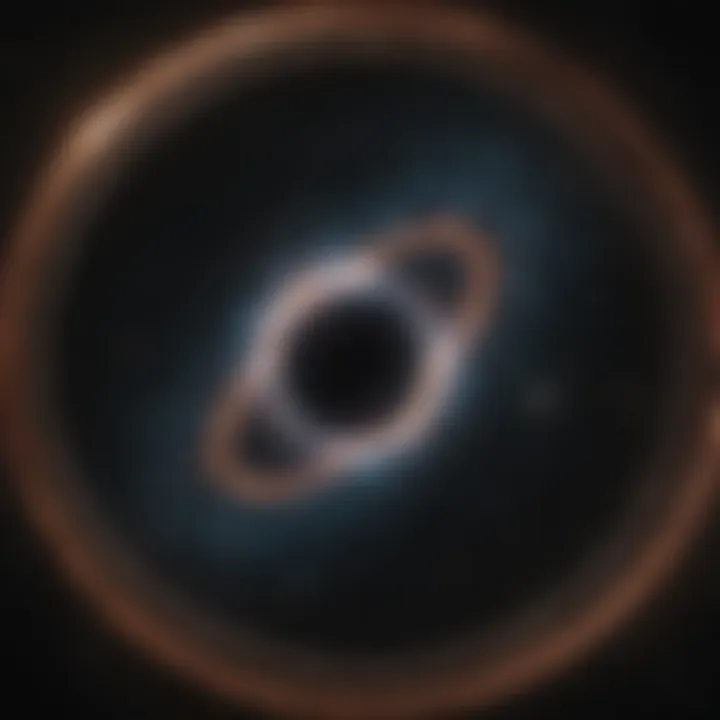
Through the decades, various theories and models have been proposed to explain dark matter, leading to a rich field of study that continues evolving. As research has progressed, the initial ideas have expanded, incorporating contemporary cosmology and particle physics to frame this significant cosmic mystery.
"Understanding dark matter is key to unraveling the greatest mysteries of the cosmos."
The historical context of dark matter sets the stage for contemporary research and the ongoing quest to uncover its true nature.
Theoretical Foundations
The exploration of dark matter begins with a solid understanding of its theoretical foundations. This section holds immense significance as it not only contextualizes dark matter within the realm of cosmology but also discusses how fundamental principles of physics shape our comprehension of this elusive component. By delving into early models, the vital role of gravity, and contemporary theories, we can appreciate the complexity and importance of dark matter research. This exploration invites readers to consider how these theoretical underpinnings guide current investigations and expand our understanding of the universe.
Early Models of Cosmology
In the development of cosmology, early models set the stage for the consideration of dark matter. Before the 20th century, the dominant view was that the universe was static and unchanging. Albert Einstein's theory of general relativity introduced a new framework by explaining gravity as a curvature of spacetime. However, it was not until Edwin Hubble's observations in the 1920s that the idea of an expanding universe gained traction.
Hubble's findings indicated that galaxies are moving away from each other, prompting a shift in thinking about the cosmos. This expansion raised essential questions about the overall mass and energy distribution in the universe. Initial models began to highlight discrepancies in the perceived motion of galaxies. Some galaxies seemed to be moving faster than could be explained by their visible mass alone. This phenomenon hinted at the existence of a hidden mass, later termed dark matter, lurking beyond observable limits.
The Role of Gravity
Gravity, a key force in the universe, plays a crucial role in understanding dark matter. It governs the interactions between celestial bodies and influences the structure formation in the cosmos. The expansion of the universe as noted by Hubble was not uniform. Instead, gravitational interactions suggested that unseen masses contributed to the effective gravitational pull on visible matter.
This gravitational lensing effect is one critical aspect that points to the existence of dark matter. Light from distant galaxies bends as it passes near massive objects due to gravity. The level of bending can be observed even if the mass creating the influence is undetectable. Thus, gravity serves as a bridge between the observable universe and the vast, invisible mass of dark matter that shapes it.
Current Theories Explaining Dark Matter
Today, several theories aim to explain dark matter and its properties. While the existence of dark matter is widely accepted, its exact nature remains elusive. The most prominent theory involves weakly interacting massive particles (WIMPs). WIMPs are hypothesized particles that interact through the weak nuclear force and gravity. Although they have not yet been detected directly, their presence is inferred through their gravitational influence on visible matter.
Another emerging candidate is the axion, a hypothetical particle proposed to resolve issues related to quantum chromodynamics. Unlike WIMPs, axions are thought to be much lighter and could help explain discrepancies observed in cosmic microwave background radiation.
Sterile neutrinos represent another interesting avenue of research. These are heavier cousins of ordinary neutrinos and may interact even weakly with normal matter. Each of these theories adds a layer to our understanding of dark matter, illustrating the complexity of the phenomena we are trying to investigate.
These theoretical foundations provide the necessary context for exploring dark matter in greater depth. As research continues, questions regarding the universe's composition, structure, and fate will remain interconnected with our understanding of dark matter.
Direct and Indirect Evidence
Understanding direct and indirect evidence is crucial in the study of dark matter. This section highlights the significance of these various forms of evidence in revealing insights about dark matter's nature and its role in the universe.
Gravitational Effects on Visible Matter
Gravitational effects provide some of the clearest evidence for dark matter. Observations that show the motion of galaxies reveal discrepancies that cannot be accounted for by visible matter alone. When scientists analyze the rotation speeds of galaxies, they notice that the outer stars rotate at speeds that contradict predictions based solely on the visible mass. This inconsistency suggests that additional unseen mass is exerting influence through gravity.
The concept of gravitational lensing further supports this notion. Light from distant objects gets bent when it passes near massive objects, including those made of dark matter. By measuring the extent of this bending, astrophysicists can infer the presence and distribution of dark matter surrounding galaxies or clusters. Therefore, gravitational effects serve as a critical piece of the puzzle in establishing evidence for dark matter.
Cosmic Microwave Background Radiation
The Cosmic Microwave Background Radiation (CMB) is another pivotal source of evidence. This radiation, a remnant from the early universe, provides a snapshot of its condition approximately 380,000 years after the Big Bang. Variations in temperature within the CMB reveal hints about the density fluctuations in the universe. Dark matter plays a significant role in shaping these fluctuations.
Through observations made by missions like the Wilkinson Microwave Anisotropy Probe (WMAP) and the Planck satellite, researchers have gathered data that predicts the effects of dark matter on the large-scale structure of the universe. The detailed measurements indicate that without dark matter, the observed CMB would differ substantially. Hence, the CMB serves as a crucial indirect evidence for the existence and effects of dark matter on cosmic scales.
Galaxy Rotation Curves
Galaxy rotation curves present yet another compelling line of evidence for dark matter. When observing how galaxies rotate, astronomers plot the orbital speeds of stars against their distance from the galactic center. In many cases, these curves reveal that stars on the outer regions of galaxies move much faster than would be expected if only visible matter were present.
Typically, one would expect the rotation speed to decline with distance from the center. However, the observed stable speeds suggest the presence of a large amount of unseen mass extending well beyond what we can detect. This mass is interpreted as dark matter. The surprising stability of rotation curves across different galaxies reinforces the notion that dark matter is a significant component of galactic structure.
"Gravitational effects, CMB data, and galaxy rotation curves collectively provide robust evidence for the existence of dark matter, shaping our understanding of cosmic structure and dynamics."
In summary, the various forms of direct and indirect evidence not only highlight the elusive nature of dark matter but also underscore its profound influence in shaping the universe. By carefully analyzing these sources, the scientific community continues to refine its understanding and approach toward the mysteries surrounding dark matter.
Search for Dark Matter
The quest for dark matter represents a pivotal endeavor in contemporary astrophysics and cosmology. This search is not merely an academic pursuit; it carries implications that touch on fundamental questions about the universe's structure and origins. Understanding dark matter is essential because it constitutes a significant portion of the universe's mass. Its presence influences the behavior of galaxies and clusters, and without it, our comprehension of cosmic dynamics would be fundamentally incomplete.
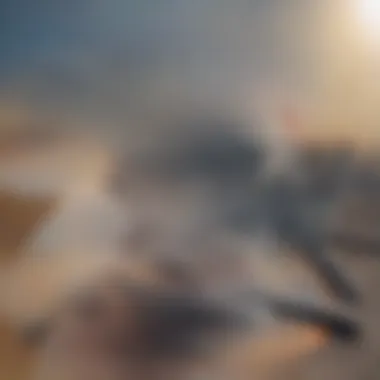
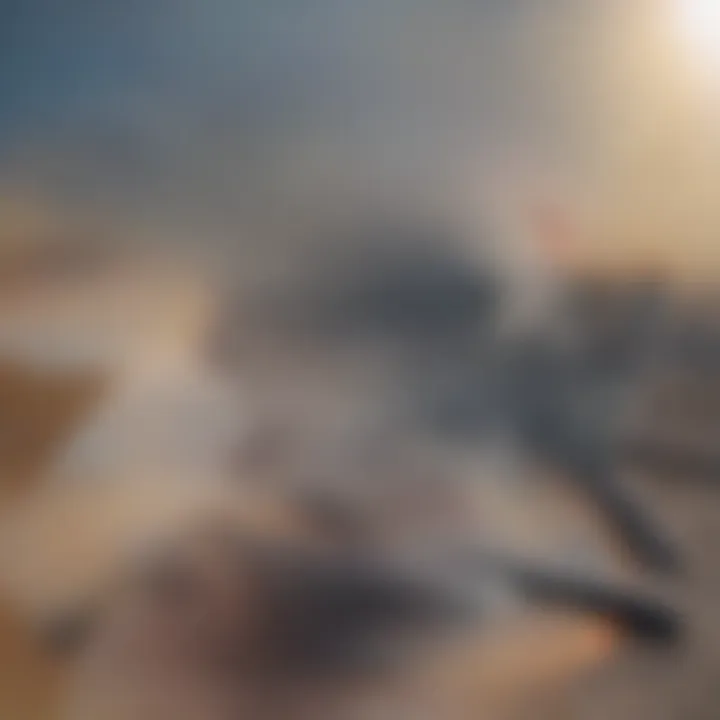
By investigating dark matter, researchers aim to uncover the unseen forces governing the cosmos. Identifying its properties may reveal new physics beyond established theories, potentially reshaping our understanding of fundamental interactions. The discovery of dark matter particles could explain numerous cosmic phenomena that current models cannot fully clarify. Thus, the search for dark matter is not just important for theoretical knowledge, but it also drives technological advancements in experimental physics.
Experimental Approaches
Experimental approaches to detect dark matter focus on direct and indirect methods. Direct detection aims to observe interactions between dark matter particles and ordinary matter. Sophisticated detectors are placed deep underground to shield them from cosmic rays and other background noise. These detectors often use materials such as liquid xenon or germanium, specifically designed to react with hypothetical dark matter particles. The primary goal is to capture the rare events where a dark matter particle collides with an atom, producing measurable signals.
In addition to direct methods, indirect detection strategies seek to identify signs of dark matter interactions or annihilations. This can occur in regions of high dark matter density, such as the center of galaxies. By observing gamma rays or neutrinos emanating from these regions, scientists can infer the presence and characteristics of dark matter. Researchers also utilize particle accelerators, like the Large Hadron Collider at CERN, to create conditions that may allow for the production of dark matter particles.
Observational Initiatives
Observational initiatives play a key role in understanding dark matter. Advanced astronomical instruments and observatories are crucial for gathering data on cosmic structures. Telescopes, such as the Hubble Space Telescope and the upcoming James Webb Space Telescope, allow scientists to observe the effects of dark matter on galaxy formation and distribution.
Moreover, initiatives like the Dark Energy Survey and the European Space Agency's Euclid mission aim to map the universe's large-scale structure. They collect extensive data on galaxy positions and movements, which helps deduce the influence of dark matter in cosmic evolution.
These initiatives not only focus on understanding dark matter's role in structure formation but also analyze its connection with dark energy. By observing distant structures and their behaviors, astronomers hope to gain insights into how these two mysterious components of the universe interact.
"The search for dark matter continually pushes the boundaries of our understanding of the universe, presenting both challenges and opportunities for discovery."
In summary, the search for dark matter encompasses a range of experimental and observational techniques. Each approach contributes to a deeper understanding of this enigmatic substance, which holds the key to unraveling the mysteries of the universe. As research progresses, the confluence of these efforts may finally lead to the resolution of dark matter's enigma.
The Role of Particle Physics
The exploration of dark matter is deeply intertwined with particle physics. This branch of physics investigates the fundamental constituents of matter and the forces governing their interactions. Understanding dark matter requires knowledge from particle physics to theorize what this mysterious substance could be. The lack of direct detection of dark matter necessitates theoretical frameworks that go beyond classical physics concepts.
Particle physics provides several insights into dark matter candidates. The most recognized framework for understanding particle interactions is the Standard Model. This model encompasses various elementary particles and their interactions, laying the groundwork for experimental approaches to dark matter.
Another significant aspect of the role of particle physics lies in its ability to predict new physics. If dark matter does not align with current understanding, it implies a need for models that extend past the Standard Model. This can lead to a deeper comprehension of the universe's fundamental fabric.
The Standard Model
The Standard Model of particle physics describes the electromagnetic, weak, and strong nuclear forces, along with the fundamental particles involved in these interactions. It consists of quarks, leptons, and bosons, which provide a framework for most known phenomena in particle physics.
However, the Standard Model has limitations. It excludes gravity and does not account for dark matter. Despite this, it is crucial in guiding researchers in the search for dark matter. For instance, many dark matter candidates, like Weakly Interacting Massive Particles (WIMPs), fit into the Standard Model structure. Research continues to focus on WIMPs as they could offer a solution to dark matter's elusive nature.
Beyond the Standard Model
Extensions of the Standard Model are essential to deepen our understanding of dark matter. Various theories propose new particles or interactions that might explain dark matter's properties. Some of these propositions include supersymmetry, which suggests that every particle has a heavier partner.
Moreover, axions and sterile neutrinos represent another class of theorized particles beyond the Standard Model. These hypotheses encourage experimental physicists to design detectors that can observe these new particles directly or infer their presence indirectly through their interactions with known particles.
In summary, the integration of particle physics enables researchers to better understand dark matter's role in the universe. It not only helps identify potential candidates but also challenges established theories, leading to broader implications in cosmology. This blend of particle physics and cosmology is essential for unraveling the mystery of dark matter.
Dark Matter Candidates
Understanding dark matter candidates is crucial in our exploration of the cosmos. These candidates help scientists hypothesize about the types of particles that could account for the missing mass in the universe. As we delve into this topic, we will cover three primary candidates: Weakly Interacting Massive Particles, axions, and sterile neutrinos. Each category presents unique features and implications for our understanding of the universe.
Weakly Interacting Massive Particles (WIMPs)
WIMPs are among the leading candidates for dark matter. They are predicted to have mass in the range of 10 to 1000 GeV/cยฒ. What makes WIMPs particularly intriguing is their weak interaction with ordinary matter, which makes them hard to detect. This feature also explains why dark matter has eluded direct observation despite making up a significant fraction of the universe's mass.
Experimental searches, such as those conducted by the Large Hadron Collider and underground detectors like the LUX-ZEPLIN, aim to create conditions where WIMPs could be produced or detected. The potential discovery of WIMPs would have far-reaching consequences for particle physics and cosmology, confirming a key aspect of the prevailing models of dark matter.
Axions
Axions are hypothetical elementary particles proposed to solve the strong CP problem in quantum chromodynamics. They are also considered a promising candidate for dark matter due to their predicted properties. Axions are light and do not interact much with normal matter, making them difficult to detect. Their existence would imply an invisible and stable component of the universe, potentially forming a significant portion of dark matter.
Current experimental efforts, such as the Axion Dark Matter Experiment (ADMX), aim to detect axions by looking for their conversion to photons in strong magnetic fields. The implications of axions extend beyond dark matter. Their discovery would open new avenues in both particle physics and cosmology.
Sterile Neutrinos
Sterile neutrinos are another intriguing class of dark matter candidates. Unlike active neutrinos, which interact via the weak force, sterile neutrinos do not couple with other particles. This makes them very elusive and hard to detect directly. Theoretical models suggest they could be heavier than active neutrinos, possibly contributing to the dark matter content of the universe.
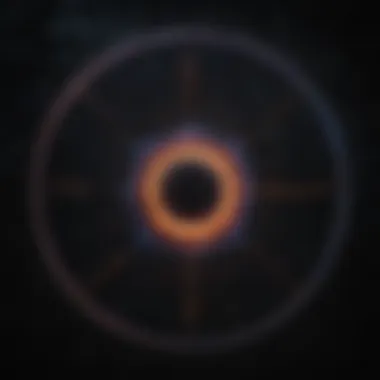
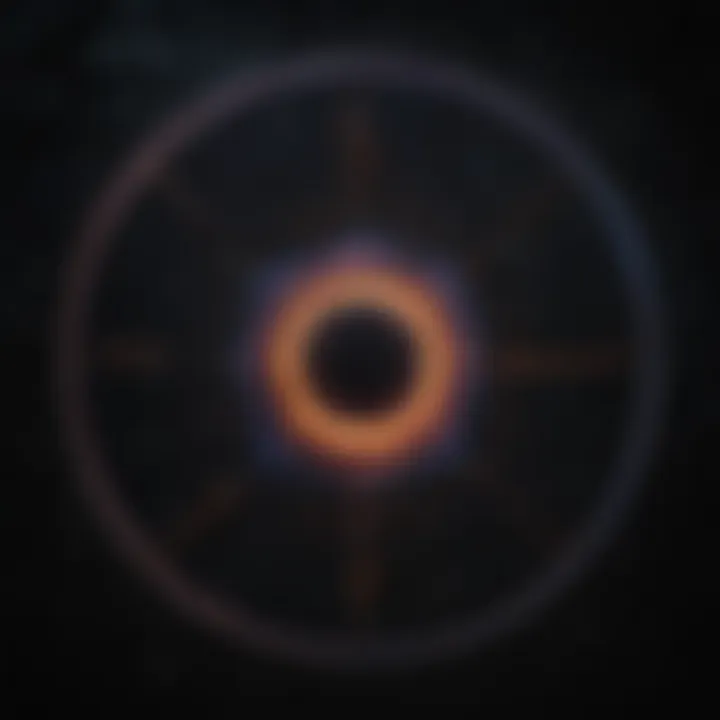
Sterile neutrinos may interact with active neutrinos and could have left observable signatures in the cosmic microwave background radiation. Furthermore, their existence could explain some anomalies in neutrino emission studies. As researchers continue to investigate all three candidates, the quest for understanding dark matter advances, enhancing our knowledge of the universe and its structure.
The ongoing study of dark matter candidates is not only about finding the missing mass but also unlocking the fundamental nature of the universe itself.
Implications for Cosmology
The topic of dark matter has vast implications for cosmology and our understanding of the universe. Its presence influences the structure, formation, and evolution of galaxies. While dark matter itself is invisible, its gravitational effects are visible throughout the cosmos. This section will cover how dark matter shapes cosmic structures and interacts with dark energy, underscoring its critical role in the framework of modern cosmological theories.
Structure Formation
Dark matter plays a fundamental role in the formation of cosmic structures. Initially present in the early universe, dark matter contributed significantly to how matter clumped together under the force of gravity. Without dark matter, the universe as we know it would not exist. The gravitational potential of dark matter creates wells in which ordinary matter can accumulate. These wells are where galaxies form and grow.
Studies like the Sloan Digital Sky Survey have shown how structures, from clusters of galaxies to filaments, tend to cluster in ways that support the existence of dark matter. Observations indicate that about 85% of the universe's mass is attributed to dark matter. This points to a gravitational framework that governs the behavior of galaxies.
Greater understanding of structure formation has been enhanced by simulations. These simulations often reveal that ordinary matter must be surrounded by dark matter to stabilize and form into larger structures. The popular , or Lambda Cold Dark Matter model, posits that dark matter is both cold and non-relativistic.
- Key Points:
- Dark matter influences galaxy formation and stability.
- Observations of cosmic structures support dark matter's existence.
- Simulations align with the Lambda Cold Dark Matter model.
Dark Energy and Its Interaction with Dark Matter
Dark energy is another central component of cosmology, associated with the universe's accelerated expansion. While dark matter pulls structures together through gravity, dark energy acts in opposition. This dual influence complicates our understanding of the universe's fate.
Recent findings suggest that dark matter may have a relationship with dark energy. Some models propose that dark matter could affect the properties of dark energy. For instance, the presence of dark matter might change the expansion dynamics. Moreover, interactions between dark matter and dark energy, although hypothetical, present intriguing avenues for exploration.
The continued study of dark energy and its relationship with dark matter will be crucial. Understanding this interplay could reveal why the universeโs expansion is accelerating and what this means for the future.
"The dynamic balance between dark matter and dark energy dictates the universe's growth and expansion, raising pivotal questions about its ultimate fate."
The Future of Dark Matter Research
The exploration of dark matter stands at a pivotal juncture in modern astrophysics and cosmology. As researchers strive to unravel the enigmatic properties of this elusive substance, the implications for our understanding of the universe are vast. The future of dark matter research offers a wealth of possibilities, promising advancements that can reshape our foundational concepts in physics and cosmology.
Technological Innovations
Technological progress plays a crucial role in the quest to understand dark matter. The development and refinement of sophisticated instruments have become paramount in examining cosmic phenomena. Noteworthy innovations include:
- Large Hadron Collider (LHC): This facility at CERN investigates high-energy collisions, potentially revealing new particles that could constitute dark matter.
- Dark Matter Direct Detection Experiments: Projects like the LUX-ZEPLIN and XENONnT are designed to detect rare interactions between dark matter candidates and ordinary matter. They utilize ultra-sensitive technologies that can measure even minute energy deposits.
- Astrophysical Surveys: Surveys like the Vera C. Rubin Observatory are set to map the night sky in unprecedented detail. This data will enhance our understanding of galaxy formation and the distribution of dark matter in the universe.
Incorporating these technologies not only improves the precision of measurements but also expands the scope of what can be observed. As innovations continue to emerge, the tools available for probing the darker aspects of our universe will only become more advanced.
Predicted Discoveries and Their Impact
As research progresses, various predictions concerning dark matter and its components arise. Some potential discoveries include:
- The identification of WIMPs or other particles hypothesized to make up dark matter could fundamentally alter our understanding of particle physics.
- New insights into the interaction between dark matter and baryonic matter might explain phenomena currently surrounding galaxy dynamics and formation.
- The detection of axions or sterile neutrinos would provide evidence supporting specific theories beyond the Standard Model of particle physics.
These discoveries are poised to have significant repercussions. Not only would they enhance our knowledge of the universe's structure, but they might also provide answers to pressing questions regarding gravity, cosmological expansion, and the ultimate fate of the universe. As researchers continue to delve into the complexities of dark matter, their efforts promise to illuminate the profound mysteries that shape our cosmic existence.
The pursuit of dark matter research is not merely an academic inquiry; it is a quest that holds the potential to redefine our very understanding of reality.
Closure
In this article, the conclusion serves a crucial role in framing the ongoing dialogue about dark matter. It encapsulates the discoveries made throughout the exploration of this topic, while also recognizing the gaps that remain. Dark matter plays a foundational role in our understanding of cosmic structure and evolution, so addressing its mysteries remains vital.
Summary of Key Findings
The exploration of dark matter has revealed several key findings:
- Dark matter constitutes approximately 27% of the universeโs mass-energy content, profoundly influencing galactic formation and dynamics.
- Numerous indirect evidences, such as gravitational effects, rotation curves, and cosmic microwave background radiation, support the existence of dark matter despite its invisibility.
- Various candidates for dark matter, including Weakly Interacting Massive Particles (WIMPs) and axions, present diverse approaches to uncover its nature.
- Current research has increasingly leaned on technological innovations and collaborations to enhance detection methods in the quest for direct evidence.
- The implications of these findings are critical for cosmology, particularly regarding structure formation and the interplay with dark energy.
The Importance of Continued Investigation
The unexplained qualities of dark matter invite further investigation. Understanding it can reshape cosmological theories and our comprehension of physical laws. Continuing research also encourages:
- Development of more advanced detection techniques, which could lead to breakthroughs in revealing dark matter's nature.
- Collaboration among diverse scientific communities, bridging gaps between particle physics and astronomy.
- Insightful predictions that could refine our model of the cosmos and help clarify the universe's fate.
The complexity and mystery surrounding dark matter demand an ongoing commitment to exploration in this domain. As we draw closer to comprehending dark matter, we can achieve significant advancements across multiple fields of science, thereby enriching our overall understanding of the universe.