Exploring Contemporary Physics Theories and Discoveries
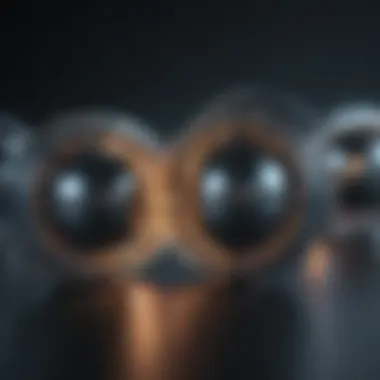
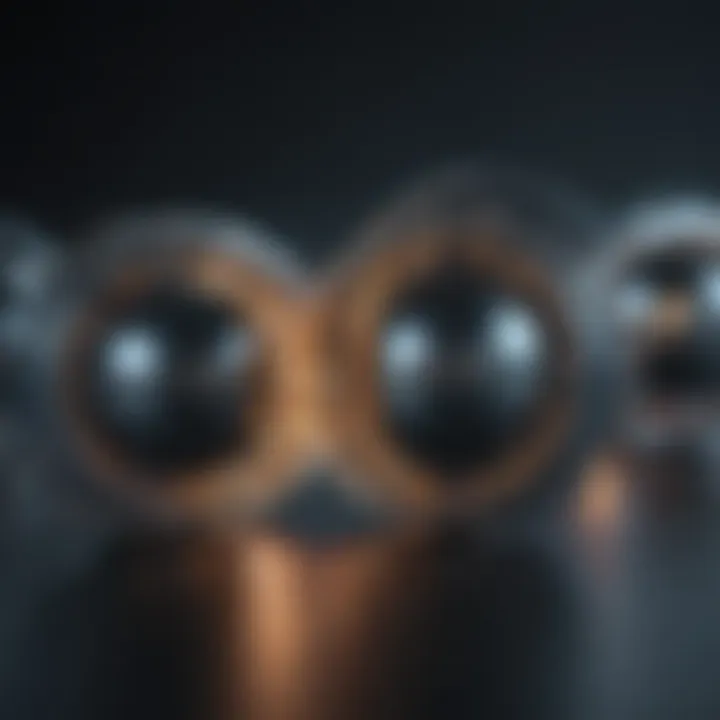
Intro
Physics, as a dynamic field, constantly evolves through rigorous research and innovative theories. The comprehension of the universe hinges on these theories, which not only shape scientific understanding but also influence technological advancements. In this exploration, we delve into contemporary physics, highlighting key research findings, simplifying complex concepts, and elucidating their implications. Our narrative targets students, researchers, and professionals looking for a deeper insight into recent advancements in the discipline.
Key Research Findings
Overview of Recent Discoveries
Recent years have seen notable progress in various areas of physics. A few key discoveries stand out:
- The detection of gravitational waves by the LIGO collaboration has confirmed a major prediction of general relativity.
- The discovery of the Higgs boson at CERN has provided answers about mass generation in particles.
- Research into dark matter and dark energy continues to unravel mysteries of cosmic composition and expansion.
- Quantum entanglement experiments are providing insights into the foundational aspects of reality, challenging classical interpretations of physics.
Each discovery not only sheds light on fundamental questions but also opens new avenues for research and technology.
Significance of Findings in the Field
The implications of these findings are profound. For instance, gravitational waves have paved the way for a new era of astrophysics. Scientists now possess a tool to observe cosmic events that were previously invisible. Similarly, understanding the Higgs boson deepens our grasp of particle physics and the Standard Model.
The search for dark matter is critical. Approximately 85% of the universe's mass remains unidentified. This fact drives numerous studies, and its resolution could revolutionize cosmology.
"Physics is not about finding the right answers; it's about asking the right questions."
Breakdown of Complex Concepts
Simplification of Advanced Theories
Many contemporary theories can seem intricate. However, breaking them down into simpler components aids comprehension. For example:
- String Theory: Asserts that fundamental particles are one-dimensional strings rather than point-like objects. This concept seeks to unify the multiple forces of nature.
- Quantum Mechanics: Explores the behavior of particles at the smallest scales, challenging intuitive notions about reality with principles like superposition and entanglement.
Visual Aids and Infographics
Utilizing visual aids enhances understanding of complex theories. Infographics can illustrate relationships and processes that text alone might find difficult to convey. For instance:
- Diagrams of the Standard Model convey how different particles interact.
- Infographics depicting black holes help visualize their profound effects on spacetime.
Preface to Modern Physics
Modern physics represents a key transition in how we understand the fundamental principles of the universe. It encompasses theories developed in the late nineteenth and twentieth centuries, which challenge and expand upon classical physics. The importance of this field in academia and research cannot be overstated. As we embark on an exploration of the latest physics theories, it's crucial to highlight how these modern concepts provide insights into complex phenomena that classical theories struggled to explain.
Recent advancements in physics, particularly those occurring in quantum mechanics and relativity, allow scientists to address challenges that have puzzled humanity for centuries. Not only do they illuminate the behavior of matter and energy at microscopic scales, but they also deepen our understanding of cosmic events, such as black holes and gravitational waves. Here, we will discuss the foundational elements of modern physics, the historical evolution of theories, and their implications for contemporary scientific inquiry.
Defining Physics in the Twenty-First Century
In the twenty-first century, physics has evolved into a multi-disciplinary science that intersects with fields such as chemistry, biology, and engineering. The definition of physics has expanded to include areas like biophysics and quantum computing. This broadening of scope illustrates the relevance of physics in addressing real-world problems. Notably, concepts such as dark matter and quantum entanglement have emerged, promoting fresh perspectives on the nature of the universe.
Furthermore, physics today embraces technological advancements. Sophisticated instruments and computational methods enhance research capabilities. Concepts like artificial intelligence and machine learning are used to analyze massive data sets from particle collisions and astronomical observations, yielding new insights and accelerating the pace of discovery.
Historical Context and Evolution of Theories
The evolution of physics is not merely a linear progression but rather a complex tapestry of ideas and discoveries. Classical physics laid the groundwork. Sir Isaac Newton's laws of motion and gravitation provided a framework. However, as technology progressed, anomalies in classical theory prompted re-evaluations during the late nineteenth century.
The introduction of quantum mechanics shattered notions of determinism. Max Planck and Albert Einstein helped to shape a new paradigm, highlighting the quantized nature of energy. This transition was pivotal as it opened discussions about the probabilistic nature of subatomic particles.
In parallel, Einstein's theory of relativity redefined our understanding of space and time. The implications of relativity extend beyond theoretical physics to affect cosmology and astrophysics, reshaping interpretations of time, light, and the fabric of spacetime.
As we review modern physics, it becomes evident that the historical context is essential. Each theoretical advancement builds upon previous knowledge while simultaneously challenging established ideas. This dynamic interplay fosters a scientific environment conducive to innovation and discovery.
Quantum Mechanics and Its Recent Developments
Quantum mechanics serves as the bedrock of modern physics, fundamentally reshaping our understanding of the microscopic realm. It is crucial for explaining phenomena that classical physics cannot adequately address, such as the behavior of particles at the atomic and subatomic levels. As we venture deeper into the intricacies of quantum theory, new developments continue to surface, pushing the boundaries of scientific inquiry and technological advancement. This section explores the principles of quantum mechanics, the latest breakthroughs in quantum computing, and fresh perspectives on quantum entanglement.
Principles of Quantum Mechanics
The principles of quantum mechanics revolve around several core concepts that challenge traditional notions of reality. Key elements include:
- Wave-Particle Duality: This principle posits that particles, such as electrons, exhibit both wave-like and particle-like properties, depending on the type of measurement performed.
- Uncertainty Principle: Formulated by Werner Heisenberg, this principle states that one cannot simultaneously know both the position and momentum of a particle with arbitrary precision. This uncertainty is not due to limitations in measurement, but rather a fundamental property of nature.
- Superposition: A quantum system can exist in multiple states at once until it is observed or measured, at which point it collapses into one of the possible states.
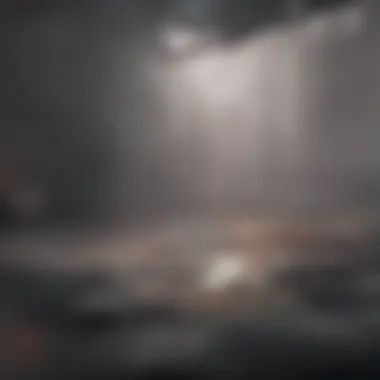
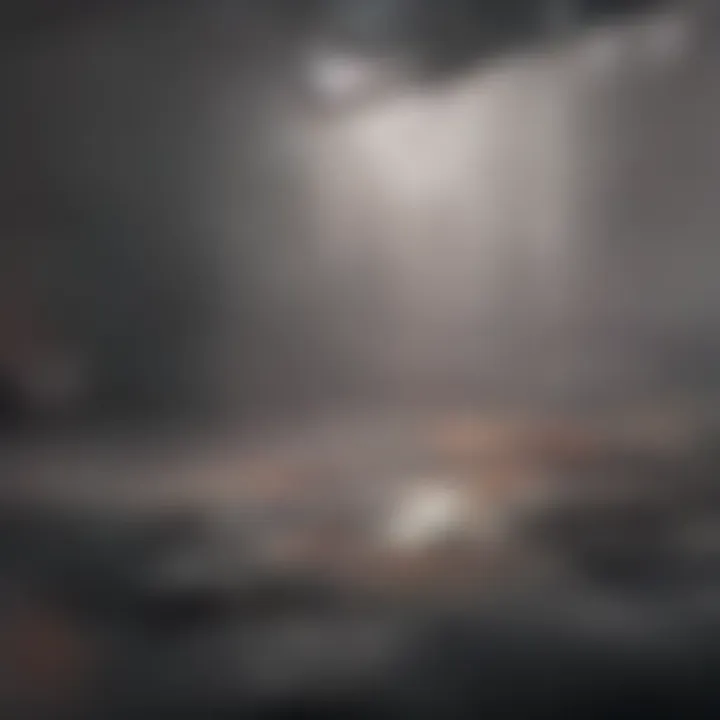
These principles fundamentally alter our understanding of reality, highlighting the probabilistic nature of quantum events and introducing an elegance that invites both philosophical and scientific reflection.
Latest Discoveries in Quantum Computing
In recent years, quantum computing has emerged as a field of immense potential, revolutionizing the ways we process information. Some noteworthy advancements include:
- Google's Quantum Supremacy: In 2019, Google announced it achieved quantum supremacy, demonstrating that their quantum processor, Sycamore, could solve a specific problem faster than the world's most advanced classical supercomputers.
- IBM's Quantum Initiative: IBM has been at the forefront of quantum computing research with its IBM Quantum Experience, offering cloud-based access to quantum processors for experimentation and development.
- Development of Quantum Algorithms: Recent focus on creating algorithms, like Shor's algorithm for factoring large numbers, showcases the potential advantages of quantum computation in cryptography and optimization problems.
These discoveries lay the groundwork for innovations that could transform industries ranging from cybersecurity to pharmaceuticals.
Quantum Entanglement: New Perspectives
Quantum entanglement is an intriguing phenomenon where particles become interconnected in such a way that the state of one instantaneously influences the state of another, regardless of the distance separating them. Recent perspectives in this field include:
- Applications in Quantum Communication: Entanglement has garnered attention for its potential to enhance secure communication methods through quantum key distribution, ensuring that any attempt at eavesdropping disrupts the quantum state.
- Entanglement in Quantum Teleportation: Researchers are exploring the feasibility of teleporting quantum states through entangled particles, raising possibilities for advanced computing and communication methods.
- Philosophical Implications: The peculiar nature of entanglement raises questions about locality and the nature of reality itself, inviting deeper investigations into the intersections of physics and philosophy.
"Quantum entanglement is not just a phenomenon of the quantum realm, but a gateway to understanding the deeper connections that govern the universe."
As we continue to unravel the complexities of quantum mechanics, it becomes clear that these recent developments are not merely academic. They offer a glimpse into a future where our understanding of the universe can fundamentally shift, influenced by the profound principles and discoveries arising within the quantum domain.
Relativity: Unraveling the Cosmos
Relativity, a groundbreaking theory put forth by Albert Einstein, fundamentally transformed our understanding of space, time, and gravity. This section delves into the core elements of relativity and its profound implications on modern physics. Understanding relativity is crucial for both students and professionals in the field, as it underlies numerous advanced theories and applications in contemporary physics. The insights gained from exploring relativity not only enhance our comprehension of the universe but also support the foundations of newer theories in scientific research.
The Fundamentals of General Relativity
General relativity, proposed by Einstein in 1915, extends the principles of special relativity by incorporating the effects of gravity. At its core, general relativity posits that gravity is the curvature of spacetime caused by mass. Essentially, massive objects like stars and planets warp the fabric of spacetime around them, which affects the motion of other objects. This concept can be illustrated through the analogy of a heavy ball placed on a stretched rubber sheet causing the sheet to sag.
The equations governing general relativity, known as the Einstein field equations, describe how matter interacts with spacetime. They have been pivotal in understanding phenomena such as planetary motions, light bending around massive bodies, and the expansion of the universe.
Through the lens of general relativity, the concept of time also becomes relative. Clocks in stronger gravitational fields, for example, tick slower than those in weaker fields. This has been experimentally validated through precise measurements of atomic clocks at different altitudes. Understanding these fundamentals provides a robust framework for discussing subsequent developments in the field of relativity.
Recent Findings in Gravitational Waves
Gravitational waves, first predicted by Einstein, are ripples in spacetime created by massive accelerating objects, like merging black holes or neutron stars. The detection of gravitational waves is a watershed moment in physics. In 2015, the Laser Interferometer Gravitational-Wave Observatory (LIGO) made its first direct observation of these waves, confirming their existence. This discovery opened a new window into astrophysics and allowed scientists to observe events that are invisible using traditional electromagnetic approaches.
The implications of understanding gravitational waves reach far beyond mere detection. They offer insights into the very structure of spacetime itself and provide a new method for exploring the universe's mysteries. The exact characteristics of gravitational waves carry a wealth of information about their sources, including the masses and spins of black holes and neutron stars involved in massive cosmic events.
"The detection of gravitational waves is a testament to the power of human ingenuity in understanding the universe."
Black Holes and Their Mysteries
Black holes, one of the most captivating elements of general relativity, represent regions of spacetime where gravity is so intense that nothing, not even light, can escape. Their existence raises numerous questions regarding the nature of reality, time, and information.
There are several types of black holes: stellar black holes form when massive stars collapse at the end of their life cycles, and supermassive black holes, often found at the centers of galaxies, have masses equivalent to millions or even billions of suns. The study of black holes reveals fundamental truths about the universe, including how they affect their surrounding environments and the process of accretion.
In recent years, significant advancements in imaging technology allowed astronomers to capture the first image of a black hole's event horizon in 2019. This achievement marked a milestone, providing visual evidence of these enigmatic objects and igniting discussions about their formation and behavior.
Understanding black holes furthers not just our knowledge of the cosmos but also the fundamental laws of physics, compelling scientists to explore the boundaries between quantum mechanics and relativity. These investigations shape the future of theoretical physics and give insight into the nature of the universe itself.
The Search for a Theory of Everything
The quest for a Theory of Everything (ToE) holds significant relevance in the landscape of modern physics. This ultimate theory aims to unify the four fundamental forces of nature: gravitational, electromagnetic, weak nuclear, and strong nuclear forces. The importance of this topic lies in its potential to provide a cohesive framework that explains all physical phenomena under one umbrella. Like the Holy Grail of physics, a successful ToE would represent a monumental achievement, leading to profound implications on both theoretical and practical levels.
The benefits of finding a ToE are manifold. It would bridge the gap between quantum mechanics and general relativity, two pillars of physics that, at present, do not coexist harmoniously in certain realms, such as black holes and the early universe. Exploring the creation of a ToE can also influence the direction of technological advancements, such as in quantum computing, energy production, and even materials science.
However, the pursuit presents various challenges. The complexity of existing theories and the inherent difficulties of experimental validation pose substantial hurdles. Additionally, understanding the philosophical implications of a ToE can lead to debates on the nature of reality, causality, and determinism.
"The search for a Theory of Everything is not just a scientific endeavor; it is a fundamental philosophical question about how we understand our existence in the universe."
Thus, this section will examine current approaches toward a unified theory, delve into the intricate details of string theory, and scrutinize loop quantum gravity.
Unifying Forces: Current Approaches
The investigation of unifying forces is grounded in the attempts to create cohesive models of the fundamental interactions in nature. Current approaches to finding a ToE span several theoretical frameworks, including string theory, loop quantum gravity, and other advanced models that aim to integrate the four forces.
One significant method is to explore symmetries that underlie different forces. Physicists utilize concepts such as gauge theories, which describe how forces arise from symmetries associated with the fields. By unifying these concepts, researchers are hopeful to draw connection between quantum fields and gravity.
Furthermore, numerical simulations and advanced mathematics play pivotal roles in testing various models. Aligning theories with observations is critical for validation, whether through particle collider results or astronomical phenomena. Researchers are also investigating dark matter and dark energy and how these elusive components relate to the unification of forces.
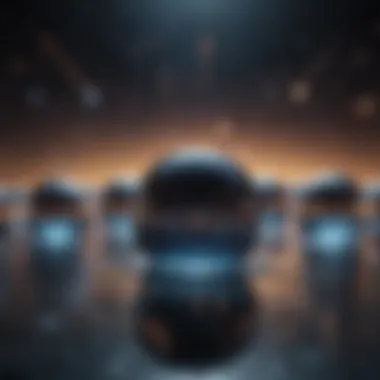
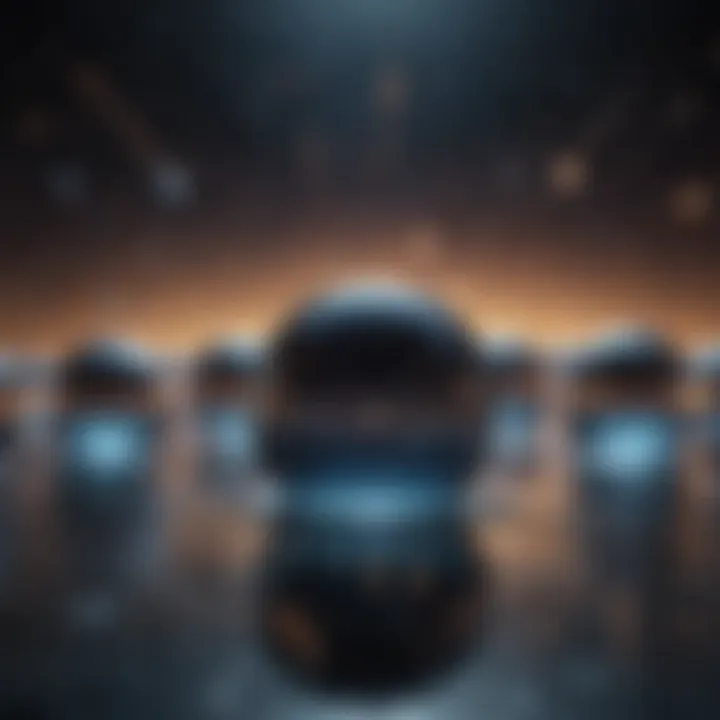
String Theory: A Comprehensive Overview
String theory posits that fundamental particles are not point-like objects but rather one-dimensional strings vibrating at different frequencies. This approach leads to the possibility of extra dimensions beyond the conventional three spatial and one temporal dimension.
There are several versions of string theory, including Type I, Type II, and heterotic string theories. Each version offers a unique take on how particles interact and how gravity can be incorporated within the quantum framework. A critical aspect of string theory is its ability to unify the four fundamental forces through the concept of dualities, where different physical theories can describe the same phenomena.
The implications of string theory extend far beyond theoretical physics. If validated, string theory may offer insights into the early universe, black holes, and the nature of spacetime itself. However, the challenge of deriving testable predictions remains a central debate in the scientific community.
Loop Quantum Gravity and Its Implications
Loop quantum gravity (LQG) presents a different perspective compared to string theory. It attempts to quantize spacetime directly by suggesting that spacetime has a discrete structure. This model endows spacetime with an atomic-like nature, where the geometry consists of quantized loops.
One of its implications is the resolution of singularities found in general relativity, such as those at the center of black holes. LQG proposes that space can be defined independently from matter, allowing for a more comprehensive understanding of the universe. This independence provides an intriguing layer of complexity to discussions of quantum mechanics, gravity, and cosmology.
However, LQG is not free from critique. The mathematical formulation and lack of empirical data test its robustness. Moreover, the intricacies involved in integrating LQG with existing frameworks continue to present theoretical challenges. This makes LQG an ongoing area of active research, potentially contributing to the overarching goal of formulating a Theory of Everything.
As we delve into these alternative approaches, it becomes evident that the search for a ToE is simultaneously an exploration of fundamental science and a reflection on our philosophical understanding of the universe.
The Role of Dark Matter and Dark Energy
The exploration of dark matter and dark energy is essential to current physics theories because these components account for a significant portion of the universe's unseen mass and energy. Understanding them is not just about filling gaps in knowledge; it is about redefining our grasp of the universe. These elements help explain why galaxies rotate as they do, the expansion of the universe, and overall cosmic evolution.
Understanding Dark Matter
Dark matter is a form of matter that does not emit, absorb, or reflect light, making it invisible and detectable only through its gravitational effects. Approximately 27% of the universe consists of dark matter, significantly influencing cosmic structures. It prevents galaxies from flying apart by providing the additional gravitational pull needed for their cohesion. Key evidence supporting dark matter includes:
- Galaxy Rotation Curves: Observations show stars at the edges of galaxies rotating more rapidly than expected. This discrepancy suggests that additional unseen mass exists.
- Gravitational Lensing: Massive objects bend light from distant sources, indicating more mass is present than observed.
- Cosmic Microwave Background: Measurements of the cosmic background radiation provide insights into the distribution of matter in the early universe, supporting the existence of dark matter.
Understanding dark matter is crucial for theories such as the Lambda Cold Dark Matter (ΞCDM) model, which serves as a backbone for modern cosmology.
The Nature of Dark Energy
Dark energy is a mysterious force driving the accelerated expansion of the universe, making up about 68% of its total energy. Its nature remains one of the greatest challenges in modern physics. The discovery of dark energy arose from observations of distant supernovae, which suggested that the universe is not just expanding but doing so at an increasing rate. Important aspects of dark energy include:
- Cosmological Constant: Proposed by Albert Einstein, this concept suggests a constant energy density filling space homogeneously.
- Dynamic Energy Field: Some theories suggest dark energy could change over time, leading to various models explaining the universeβs acceleration.
- Equation of State: This term refers to the relationship between pressure and density in dark energy, influencing cosmic dynamics and expansion.
The implications of dark energy stretch far beyond current understanding, potentially altering concepts of gravity and structure in the cosmos.
Impact of Dark Components on Cosmic Structure
Both dark matter and dark energy play pivotal roles in shaping cosmic structures. Their interplay is fundamental to theories that attempt to explain the formation and evolution of galaxies. Key impacts include:
- Structure Formation: Dark matter acts as scaffolding for galaxies, allowing normal matter to coalesce and form stars and galaxies.
- Large-Scale Structure: The distribution of galaxies across the universe reflects the influence of dark matter clumps and voids created by dark energy.
- Fate of the Universe: The balance between dark matter and dark energy influences potential scenarios for the universeβs ultimate fate, be it continued expansion, a static universe, or even collapse.
"Understanding dark matter and dark energy is vital for unraveling the mysteries of cosmic evolution and the fundamental workings of the universe."
Exploring both dark matter and dark energy enhances our comprehension of fundamental physics, driving advancements in cosmological models. This examination not only helps clarify our existing theories but also evokes new questions, prompting fresh research initiatives.
Emerging Theories in Physics
Emerging theories in physics represent a vital part of modern scientific inquiry. They challenge established paradigms and offer new lenses through which to understand the universe. As our technology advances, so does our ability to test these theories, leading to significant implications for both fundamental research and practical applications.
The exploration of emerging theories can enhance our comprehension of complex physical phenomena. They incorporate insights from various fields, including quantum mechanics, relativity, and thermodynamics. A thorough understanding of these emerging theories not only enriches the academic landscape but also can drive innovations in technology and address unresolved problems in physics. This section will explore three profound emerging theories: post-quantum mechanics theories, multiverse hypothesis, and integrative approaches in theoretical physics.
Post-Quantum Mechanics Theories
Post-Quantum Mechanics theories aim to extend and refine the principles of quantum mechanics. They emerge from challenges faced in the standard model and the need for a more comprehensive framework that can address unsolved issues. These theories can include concepts like decoherence, which explains how quantum systems interact with their environment, leading to classical behavior.
One noteworthy post-quantum proposal is Pilot Wave Theory. This theory suggests that particles have definite positions and velocities, with non-local hidden variables guiding their motion. Such hypotheses strive to bridge the gap between the deterministic world of classical physics and probabilistic quantum mechanics. As researchers delve deeper, we observe increasing interest in alternative interpretations, enabling scientists to rethink the very principles of reality.
Multiverse Theory: Scientific Stance
The multiverse theory proposes that our universe is only one among many. Each universe may have different physical laws, constants, and initial conditions. This idea stems from interpretations of quantum mechanics, specifically the many-worlds interpretation, and cosmic inflation scenarios.
"The multiverse provides a compelling framework to understand why our universe appears fine-tuned for life."
Although the multiverse concept faces skepticism due to its speculative nature, proponents argue about its potential to explain certain cosmological observations, such as the fine-tuning of constants. Yet, the theory remains controversial within the scientific community, with debates surrounding its testability and implications for our philosophical understanding of existence. To date, there has been no empirical evidence supporting the existence of other universes, which raises critical questions about the boundaries and objectives of physics.
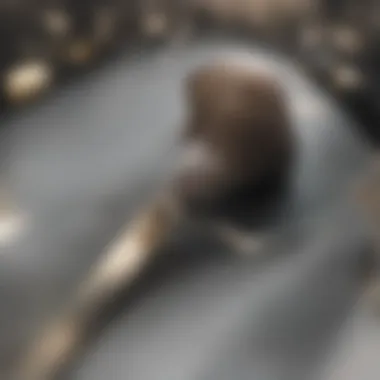
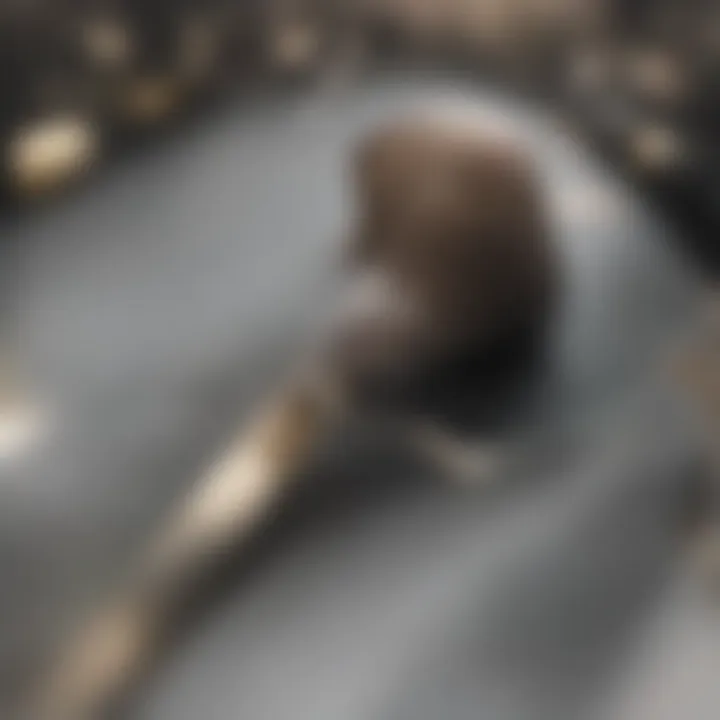
Integrative Approaches in Theoretical Physics
Integrative approaches involve blending concepts from distinct fields of physics to formulate a more unified understanding of natural phenomena. This may include collaboration between quantum mechanics and general relativity or merging statistical mechanics with thermodynamics.
Notable efforts include quantum gravity proposals, such as string theory and loop quantum gravity. These frameworks seek to reconcile the particle nature of matter with the geometric description of gravity in a cohesive manner. Such collaborations provide fresh insights into unresolved questions surrounding the fundamental forces and the fabric of spacetime.
Emerging theories in physics are critical. Understanding them challenges traditional ideas and can lead to groundbreaking discoveries. As technology evolves, the implications of these theories become increasingly significant for explaininig our universe. This ongoing research is crucial for students, researchers, and professionals aiming to deepen their comprehension of contemporary physics.
Influence of Technology on Physics Research
The impact of technology on the field of physics is profound and multifaceted. It serves as both a catalyst for new discoveries and a tool for validating established theories. In this section, we explore how modern advancements in technology contribute to physics research, focusing specifically on particle physics equipment and computational models.
Advancements in Particle Physics Equipment
Particle physics is at the forefront of exploring the fundamental building blocks of matter. Recent years have seen significant investments in advanced equipment that enhance our ability to investigate particles at unprecedented levels. Facilities like the Large Hadron Collider (LHC) at CERN exemplify this trend, where cutting-edge detectors and accelerators allow scientists to collide particles at near-light speeds. This not only enables the search for new particles but also tests the predictions of the Standard Model and beyond.
Some notable advancements include:
- High-Precision Detectors: These instruments can track particles with remarkable accuracy, allowing for better data collection and analysis.
- Superconducting Technologies: Used in magnets that steer particle beams, these technologies improve the stability and intensity of collisions.
- Cryogenic Systems: Help maintain particle detectors at extremely low temperatures, which is essential for their functionality.
By optimizing these technologies, researchers are able to push the boundaries of what is known about the fundamental forces. This enhances our understanding of phenomena such as the Higgs boson and neutrinos, with implications that extend into cosmology and beyond.
Role of Computational Models in Theory Testing
The development of sophisticated computational models has transformed physics research. These models simulate complex physical phenomena that are not feasible to observe directly. Through numerical methods, physicists can now predict outcomes and validate theories more efficiently.
Key aspects of computational models include:
- Simulations of Particle Interactions: These help visualize interactions at the quantum level, providing insights into particle behavior in various conditions.
- Data Analysis Tools: Modern algorithms process vast amounts of experimental data, extracting meaningful patterns that aid in hypothesis testing.
- Interdisciplinary Approaches: Collaborations with computer science and mathematics enhance the development of models, leading to more accurate predictions and discoveries.
"Computational models are as essential to modern physics as the laboratory apparatus was in the past. They allow us to explore scenarios we could never test physically."
Overall, the influence of technology in physics research cannot be overstated. Innovations in equipment and computational modeling not only refine experimental techniques but also deepen the theoretical underpinnings of the field. As these technologies advance, so too will our understanding of the universe.
Philosophical Implications of Modern Physics
Inquiring into the philosophical implications of modern physics offers a profound understanding of the fundamental aspects governing our reality. Physics, especially in its modern forms, is more than a collection of theories; it raises deep questions about existence, knowledge, and morality. The ideas emerging from quantum mechanics and relativity can alter our perceptions of time, space, and even what it means to be conscious. This section delivers insight into how contemporary developments in physics influence philosophical thought and, conversely, how philosophical inquiry can guide physicists in their explorations.
Causality and Free Will in Contemporary Physics
Causality has long been a core principle underlying physics and, by extension, our understanding of free will. Classical physics saw the universe as deterministic, suggesting that every event had a preceding cause. However, the emergence of quantum mechanics calls this perspective into question. In quantum theory, certain events appear to happen without deterministic causes, proposing a model where probability plays a key role. This reality challenges traditional notions of free will.
The dialogue between physics and philosophy here is crucial. If events at the quantum level cannot be predicted with confidence, what does that mean for the concept of free will? Some argue that free will might be an illusion if our actions are influenced by fundamentally random processes. Others suggest that understanding the complexity of causality can lead to a richer understanding of free will itself, allowing for both determinism and indeterminism to coexist in a nuanced framework.
Impact of Quantum Theory on Philosophy
Quantum theory has significant repercussions not just in physics, but also in philosophy. Its principles challenge classical ideas about reality, knowledge, and observation. One philosophical implication arises from the concept of superposition, where a system can exist in multiple states simultaneously until observed. This leads to discussions on the role of the observer in defining reality. Some philosophers argue this changes the foundation upon which we build knowledgeβthat knowledge is not merely passive observation but actively shapes what is known.
Moreover, ideas like entanglement provoke philosophical consideration of locality and the nature of connections across spacetime. If entangled particles can affect each other instantaneously, regardless of distance, it raises questions about the limitations of human perception and knowledge.
The philosophical landscape is thus affected heavily by these advancements, prompting a reevaluation of truth, existence, and the limits of human understanding. The intersection between quantum mechanics and philosophy opens avenues for new theories, leading scholars to rethink what it means to exist and perceive in a world shaped by quantum mechanics.
"The philosophical implications of modern physics provide a fertile ground for exploring the nature of reality and our place within it."
This synthesis reveals how physics and philosophy can inform one another, presenting a richer dialogue that enhances both fields. In this interplay lies the potential for profound insights into our universe, ultimately enriching both scientific and philosophical perspectives.
Ending: The Future of Physics Theories
The study of physics is ever-evolving. As we approach the limits of our current understanding, the future of physics presents a panorama rich with potential. This section will highlight the significance of anticipating what lies ahead. By exploring this topic, readers can grasp both the implications of emerging theories and the necessity for ongoing inquiry in the field.
Predictions for Upcoming Discoveries
The landscape of physics promises to shift with the emergence of new discoveries. Physicists are currently investigating many domains, suggesting avenues for groundbreaking findings:
- Quantum Field Theories: Developments in understanding particle interactions may lead to newer frameworks. This can lead to significant enhancements in technology, including advancements in quantum computing and communication.
- Cosmological Observations: The deployment of advanced observational instruments reveals more about dark matter and dark energy. As technology improves, more accurate measurements may confirm or refute existing models of cosmic evolution.
- Novel Particles: The Large Hadron Collider and other particle accelerators may help discover new fundamental particles. This could reshape the Standard Model of particle physics and propel new theories.
"Emerging discoveries can redefine the foundations of physics, paving a pathway toward a unified understanding of our universe."
These discoveries may not only elucidate fundamental principles but also inspire interdisciplinary collaboration between physics, mathematics, and engineering.
Sustained Interest in Scientific Research
The importance of sustained scientific research cannot be overstated. Ongoing investment in theoretical and experimental physics fuels the industry's vitality.
- Interdisciplinary Approaches: Collaboration between disciplines garners new insights, especially in areas such as biophysics or quantum biology. These intersections can illuminate concepts that are otherwise elusive.
- Funding and Resources: Governments, private sectors, and educational institutions play pivotal roles in funding research initiatives. Financial backing enables scientists to pursue ambitious projects, such as those aimed at understanding the early universe or the fabric of spacetime.
- Public Engagement: Science communication plays a critical role in maintaining public interest and understanding. Engaging with non-experts can foster broader appreciation for the sciences, ensuring a healthy future for research endeavors.