Examining Single Cell Organisms: Life's Building Blocks
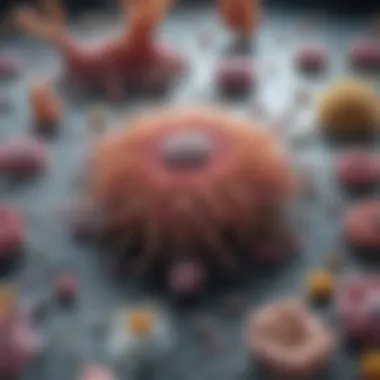
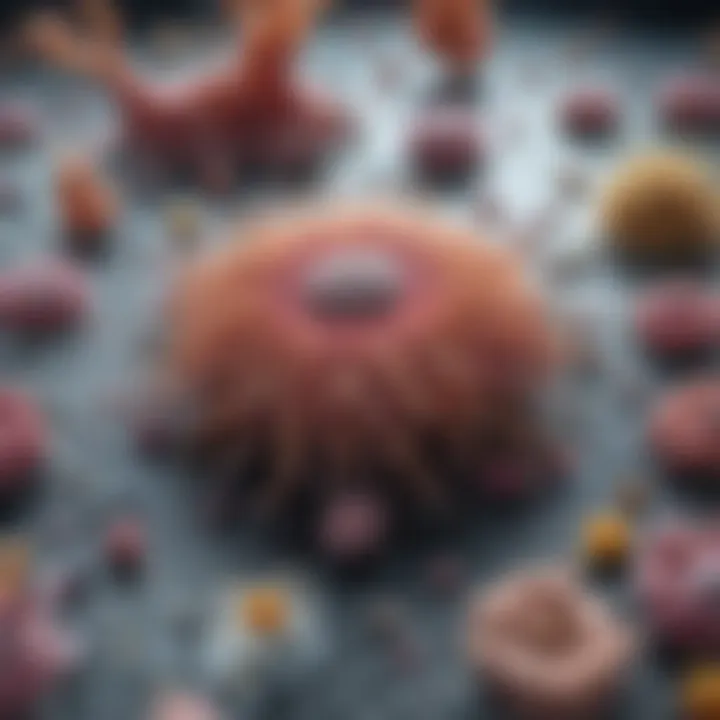
Intro
Single cell organisms are often overlooked, but they play a fundamental role in the web of life that sustains our planet. These minuscule entities, ranging from bacteria to protozoa, are the first steps in the grand narrative of biology. Understanding their unique characteristics, metabolic processes, and ecological significance offers a window into how life emerged and evolved.
As we embark on this exploration, we will uncover the compelling diversity and functionality that single cell organisms exhibit. By looking closely at their adaptations, we will see how these tiny life forms not only thrive in some of the harshest environments but also contribute to processes vital for the health of ecosystems and the advancement of scientific research.
This journey will span various aspects, from recent research breakthroughs to the implications of these organisms in biotechnology and environmental science. Each section will enrich our understanding of these remarkable entities. With that, let's dive deeper into the key research findings that illuminate the world of single cell organisms.
Preface to Single Cell Organisms
Single cell organisms might seem like tiny specks of life, almost negligible when viewed with the naked eye, but their significance is monumental. These microscopic entities serve as the building blocks of all life, shaping the world around us and possessing an astonishing range of diversity. Understanding single cell organisms paves the way for grasping the complexity of life itself, from the simplest bacteria to more intricate protozoa.
Their study opens a window into evolutionary biology, ecology, and even medicine, revealing the intricate relationships that sustain ecosystems and human health. This introduction lays the groundwork for exploring how these organisms not only survive but thrive in various environments, their defining traits, and their contributions to biological systems at large.
Definition and Characteristics
Single cell organisms, by definition, are living entities made up of a single cell. This simplicity in structure belies their complexity in function. They exist across different domains of life, primarily categorized into bacteria, archaea, and eukaryotes.
One hallmark of single cell organisms is their metabolic versatility. They can extract energy from organic material, sunlight, or inorganic substances, showcasing a remarkable adaptability to their environments. For instance, certain bacteria thrive in the deepest ocean vents where conditions are harsh, while others inhabit the human gut, playing a pivotal role in digestion. Their cellular structures vary, with some possessing rigid cell walls while others are more flexible, allowing them to alter shape and even move.
Importance in Biological Systems
The role of single cell organisms extends beyond existence; they are indispensable in ecological balance.
- Decomposers: They break down organic matter, recycling nutrients back into ecosystems, which is critical for sustainability.
- Primary Producers: Certain single cell organisms, like cyanobacteria, engage in photosynthesis, generating oxygen and forming the base of many food webs.
- Symbiotic Relationships: Many organisms rely on single cell life forms for survival, such as the gut bacteria in humans that aid in digestion.
Their influence is also seen in medical science. Some bacterial species are harnessed in the production of antibiotics, while others can lead to illness, making the study of these entities crucial for public health.
Understanding single cell organisms thus provides a foundational insight into the delicate web of life, illustrating how even the smallest of beings can have significant impacts on larger systems.
Major Types of Single Cell Organisms
Understanding the various types of single cell organisms is vital for grasping the intricate web of life on Earth. Each type offers unique benefits and plays a significant role in both environmental dynamics and technological advances. From microorganisms that facilitate nutrient cycling in soils to those that serve as bioengineered tools in medical research, these organisms are foundational to our ecosystems and societal progress.
Bacteria
Characteristics of Bacteria
Bacteria are incredibly diverse, ranging from harmless strains that live in our gut to potent pathigens. A key characteristic of bacteria is their prokaryotic nature, meaning they lack a nucleus and other membrane-bound organelles. This gives them a versatility that allows them to thrive in varied environmentsโfrom the deep ocean trenches to the human body. Their simplicity also makes them attractive models for studying fundamental life processes. One advantage of bacteria is their rapid reproduction rate, which can be a double-edged sword: while it allows for quick studies, it also means they can develop resistance to antibiotics swiftly.
Role in Ecosystems
Bacteria serve as essential players in ecosystems, acting as decomposers that break down organic matter and recycle nutrients. This nutrient cycling is crucial for maintaining the health of various habitats. A notable feature of their role in ecosystems is their ability to fix nitrogen, a critical process for plant growth. The benefits of such bacterially mediated processes cannot be understated; they support agricultural productivity, influence climate patterns, and promote biodiversity. However, the mismanagement of bacterial populations, particularly through the overuse of fertilizers, can lead to harmful algal blooms that disrupt aquatic ecosystems.
Pathogenic vs. Non-Pathogenic Bacteria
When categorizing bacteria, one of the most significant divisions is between pathogenic and non-pathogenic types. Pathogenic bacteria are known for causing diseases, ranging from mild infections to life-threatening conditions. In contrast, non-pathogenic bacteria contribute positively to health, such as those in probiotics. Understanding this distinction is important, especially in clinical settings where antibiotic treatment choices rely on targeting harmful pathogens while preserving beneficial strains. The unique feature of pathogenic bacteria often lies in their mechanisms of resistance and virulence, which continuously challenge public health systems and research methodologies.
Protozoa
Classification of Protozoa
Protozoa are a diverse group of eukaryotic microorganisms that can be classified into various categories based on their movement and nutritional strategies. These classifications include flagellates, ciliates, amoeboids, and sporozoans. Each class contains unique representatives with distinct life cycles and ecological roles. Highlighting this classification is beneficial for understanding the complex interactions they have within ecosystems, such as their role in food webs, where they serve as both predators and prey. This diversity enables scientists to study them in various contexts, from environmental monitoring to biomedical research.
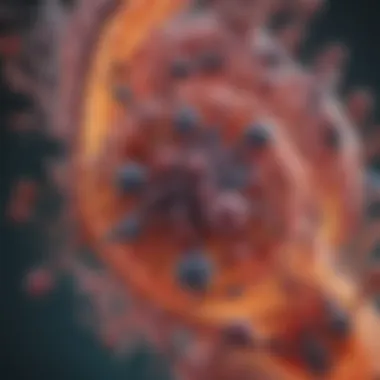
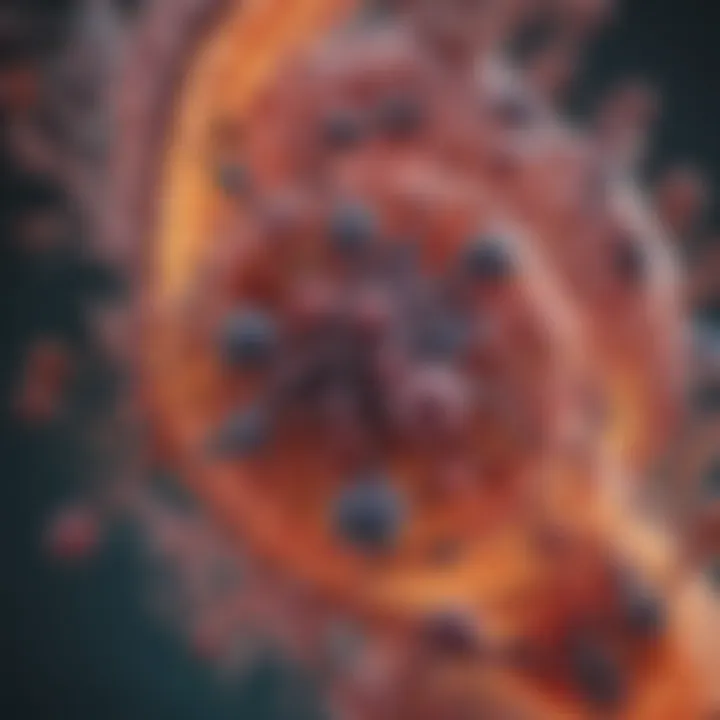
Ecological Importance
Protozoa play crucial roles in ecological balance, particularly in aquatic ecosystems. They help regulate bacterial populations, thus maintaining biodiversity by controlling microbial growth. Their consumption of bacteria and other microorganisms allows for nutrient cycling and energy transfer in food chains. One unique feature of protozoa is their capacity to exist in both free-living and symbiotic forms, which can either enhance or complicate ecological interactions. As researchers keep delving into their ecological importance, they uncover insights into environmental health and potential bioremediation applications.
Use in Research
Researchers utilize protozoa extensively as model organisms in studies focusing on cell biology, disease mechanisms, and evolutionary biology. Their relative simplicity yet complexity in behavior makes them ideal for laboratory experiments. A notable characteristic that stands out is their ability to adapt to environmental stresses, providing insights into survival strategies. The study of protozoa can lead to breakthroughs not only in understanding human diseases caused by pathogens like Plasmodium but also in developing new scientific tools and innovations.
Fungi
Yeasts and Their Applications
Yeasts, a group of single-cell fungi, are key players in both food production and biotechnology. Their fermentation capabilities are harnessed in the baking and brewing industries, where they convert sugars into alcohol and carbon dioxide. A significant feature of yeasts is their ability to undergo both aerobic and anaerobic respiration, making them versatile organisms for various industrial applications. The benefits of using yeasts include their relatively fast growth rates and the ability to genetically manipulate them for enhanced productivity or specific traits. However, care must be taken, as improper usage can lead to unwanted byproducts that alter the desired end products.
Role in Decomposition
Fungi, including yeasts, play a significant part in decomposition processes, breaking down complex organic matter and returning nutrients to the soil. This role is crucial for ecosystem health, allowing for the recycling of elements necessary for plant growth and maintaining soil quality. Fungi possess unique enzymes that can break down tough materials like lignin and cellulose, surpassing bacteria in speed and efficiency. This advantage enables fungi to thrive in habitats where other decomposers might struggle, although cases where invasive fungal species disrupt local ecosystems underscore the importance of balance.
Symbiotic Relationships
Many fungi form symbiotic relationships with plants and other organisms, known as mycorrhizae. This partnership benefits both parties: fungi improve nutrient absorption for the plant, while receiving carbohydrates in return. Highlighting symbiotic relationships is significant for understanding plant ecology, agriculture, and even forest management. The unique aspect of these connections lies in their impact on soil health and biodiversity, enhancing resilience against environmental stressors. As we learn more about these relationships, the potential for agricultural improvements and ecological restoration becomes clearer.
Functionality of Single Cell Organisms
Understanding the functionality of single cell organisms reveals their vital roles within ecosystems and their intricate operational systems. These microscopic entities carry a wealth of mechanisms that govern life processes, from energy production to reproduction and environmental adaptations. Each functionality not only shows their remarkable adaptability but also highlights their significance in broader biological contexts. Integrating insights into these aspects helps us appreciate the foundational roles of these organisms in both evolution and biotechnology.
Metabolism and Energy Production
Aerobic and Anaerobic Processes
Aerobic processes require oxygen for metabolic functions, while anaerobic processes occur in the absence of oxygen. Both pathways are essential for energy production in single cell organisms. Knowing how these different modalities function allows us to appreciate their versatility in energy generation.
A major characteristic of aerobic processes is that they yield more energy per glucose molecule โ a significant advantage when conditions allow for oxygen presence. For instance, in environments rich with oxygen, bacteria like Escherichia coli thrive, effectively converting glucose into energy through oxidative phosphorylation. However, anaerobic pathways, as utilized by organisms such as Clostridium botulinum, display a unique feature by allowing survival and energy production in oxygen-poor conditions. This makes anaerobic processes incredibly beneficial in habitats where oxygen is scarce.
"Microorganisms don't just survive; they adapt meticulously to the environment at hand - they're true master of their craft."
The disadvantages of anaerobic processes include lower energy yields, which can limit metabolic effectiveness in fast-growing environments. Thus, understanding these energy production methods informs both ecological dynamics and potential industrial applications.
Fermentation Pathways
Fermentation pathways represent an anaerobic energy-generating process, critical for many single cell organisms. This method enables organisms to convert sugars into acids, gases, or alcohol, generating ATP without the need for oxygen. A notable aspect of fermentation is its rapid energy production when oxygen is limited, making it a favored choice for yeast, used extensively in baking and brewing.
A unique feature of fermentation is the variety of end products it can yield, from lactic acid, which is pivotal for our muscle function during exercise, to ethanol in brewing. However, this efficiency can come at the cost of generating toxic by-products, making it a double-edged sword. By grasping how these pathways operate, we glean insight into metabolic diversity and its applications in food production and biofuel synthesis.
Reproduction Mechanisms
Asexual Reproduction in Bacteria
Asexual reproduction is predominant among bacteria, typically occurring through binary fission. This process involves the duplication of genetic material and division of the cell into two identical organisms, presenting a rapid way to increase population size. This efficiency in replication underscores the success of bacteria in various ecosystems.
The key characteristic of asexual reproduction is its swift nature, enabling bacteria to respond quickly to environmental changes, making them adaptable. It's a straightforward reproductive strategy, allowing for rapid colonization of environments, especially in nutrient-rich conditions. Nevertheless, this method does not allow genetic diversity, which can be a disadvantage when faced with changing conditions, potentially leading to vulnerability against environmental stresses.
Sexual Reproduction in Protozoa
Protozoa often engage in sexual reproduction, a mechanism that contributes significantly to genetic variation. This can happen through conjugation, whereby two organisms exchange genetic material, enhancing genetic diversity. The benefit of this method lies in the potential resilience against diseases and changing environments, which can be crucial for survival. What's fascinating is the strategic nature of sexual reproduction, where protozoa can switch between asexual and sexual modes, providing flexibility depending on environmental conditions. However, this complexity can also present challenges, such as the necessity for compatible mates, which may limit reproductive success in sparse populations.
Adaptations to Environment
Extremophiles
Extremophiles are a unique group of single cell organisms that thrive under extreme conditions, be it high temperatures, acidity, or salinity. The defining characteristic of extremophiles is their ability to possess specialized enzymes that function optimally under such harsh environments. For example, Thermus aquaticus, a thermophile, is essential in biotechnology, providing Taq polymerase used in PCR processes.
The advantage of extremophiles is their potential applications in industrial processes that require resilience to extreme conditions, yet they may struggle to adapt to more moderate environments, limiting their overall ecological roles.
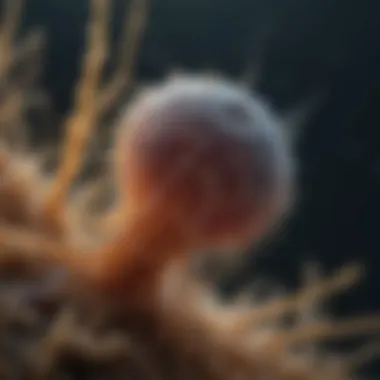
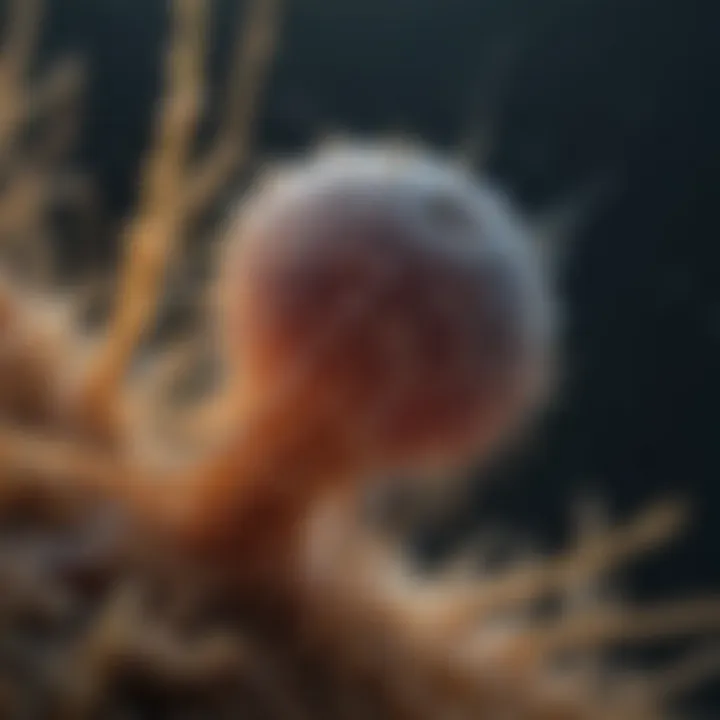
Biofilm Formation
Biofilm formation is another vital adaptation seen in many single cell organisms. This process entails the aggregation into a matrix that adheres to surfaces, providing a protective environment. A key characteristic of biofilms is their ability to resist environmental stresses, such as antimicrobials or competitive organisms.
The unique feature of biofilm formation not only promotes group survival but can also enhance nutrient acquisition. Yet, the downside includes the potential for chronic infections in medical contexts, illustrating the dual nature of biofilms. A deep understanding of biofilm dynamics may aid in developing strategies for both harnessing their benefits and mitigating their adverse effects in areas such as medicine and industry.
Scientific Research and Applications
The realm of single cell organisms isn't just a microscopic curiosity; it's a gateway to major advancements in various fields. From healthcare innovations to environmental conservation and biotechnological progress, these organisms fuel scientific inquiry and practical applications that often shift paradigms in our understanding and practice. The ability to manipulate and utilize these organisms allows researchers and professionals to tackle pressing issues facing humanity today.
Role in Biotechnological Advances
Genetic Engineering
Genetic engineering stands out as a powerful tool in the biotechnology arsenal, showing how single cell organisms serve as vectors for manipulating genetic material. By utilizing microbes like Escherichia coli as hosts, scientists can engineer new proteins or desirable traits in crops, essentially remixing nature's design.
One distinctive characteristic of genetic engineering is its precision. The ability to directly edit genes enhances the potential for outcomes that align closely with human needs, from producing insulin to creating crops resistant to pests. This targeted approach allows for a level of control and adaptability that traditional breeding methods simply can't match. While it presents tremendous opportunities for solving issues like food scarcity, it also raises questions about ethics and safety. Weighing the benefits against the potential risks becomes paramount in any discussion of this technology's future.
Industrial Applications
Industrial applications of single cell organisms encompass a vast array of processes, from fermentation to waste treatment. These microorganisms not only drive sustainable practices but also optimize production efficiencies.
A notable aspect of industrial applications is their scalability. For instance, yeast fermentation is employed in creating bread and beverages, showcasing how a single organism can contribute to diverse products. This adaptability makes such applications popular among manufacturers aiming for cost-effective solutions. However, the challenge remains in ensuring quality and consistency across large-scale production. Unique features, like the ability of certain bacteria to thrive in extreme conditions, provide advantages in certain industrial applications, but they also must be rigorously monitored to mitigate potential risks.
Medical Significance
Microbial Pathogenesis
Understanding microbial pathogenesis is crucial, given how many diseases stem from infections caused by single cell organisms. This aspect extends beyond basic health; it has far-reaching consequences in public health paradigms and treatment modalities.
One key characteristic of microbial pathogenesis is its variability. Different strains of bacteria can have diverse effects on human health, underlining the importance of tailored medical approaches. This variability can be a double-edged sword, as it allows for innovative treatment strategies but also complicates diagnostics and therapies due to evolving resistance patterns.
Use in Antibiotic Development
The role of single cell organisms in antibiotic development cannot be overstated. Essential antibiotics, like penicillin, derive from modifications of naturally occurring compounds produced by fungi and bacteria, highlighting the significant connection between nature and medicine.
A defining aspect of using these organisms in antibiotic development is the opportunity for discovering novel compounds. This search has historically yielded promising treatments for bacterial infections. While the prospect of developing new antibiotics presents exciting possibilities, it also entails navigating the challenges of resistance and the sustainability of antibiotic efficacy against ever-evolving pathogens. Understanding how single cell organisms contribute to this ongoing battle underscores their immense value in medical research.
Environmental Impact
Bioremediation Techniques
Bioremediation employs single cell organisms to clean up contaminated environments, such as oil spills or heavy metal pollution. This innovative approach leverages the natural capabilities of microbes to breakdown pollutants, thus achieving a cleaner environment through biological means.
A significant characteristic of bioremediation techniques is their sustainability. Unlike chemical methods that may harm ecosystems, biological treatments often promote long-term recovery and restore balance. The ability of certain bacteria to metabolize toxic compounds makes them beneficial for environmental rehabilitation, although itโs important to choose the right organisms and conditions to avoid unintended consequences.
Role in Nutrient Cycling
Single cell organisms are at the core of nutrient cycling. They facilitate the decomposition of organic matter and the recycling of nutrients back into ecosystems. Through processes like nitrogen fixation and carbon cycling, they contribute to the stability and productivity of ecosystems.
The unique feature of nutrient cycling involving these organisms is the speed at which they operate. Microorganisms can respond rapidly to changes in environmental conditions, ensuring that ecosystems remain resilient. Understanding this role offers insights into managing ecological health, but there are also concerns regarding how human activity disrupts these natural processes, emphasizing the need for conservation efforts and sustainable practices.
Challenges and Future Directions
The realm of single cell organisms is rich with discoveries but not without its hurdles. As we navigate the intricate relationships these organisms have within ecosystems and their vast implications in human health and biotechnology, one cannot overlook the challenges that arise. Addressing these challenges isn't merely an academic exercise; it serves as a crucial stepping stone toward advancing our understanding and beneficial applications of these organisms.
Antimicrobial Resistance
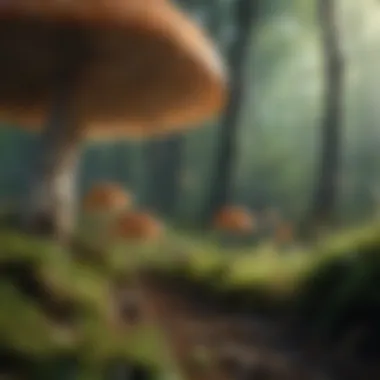
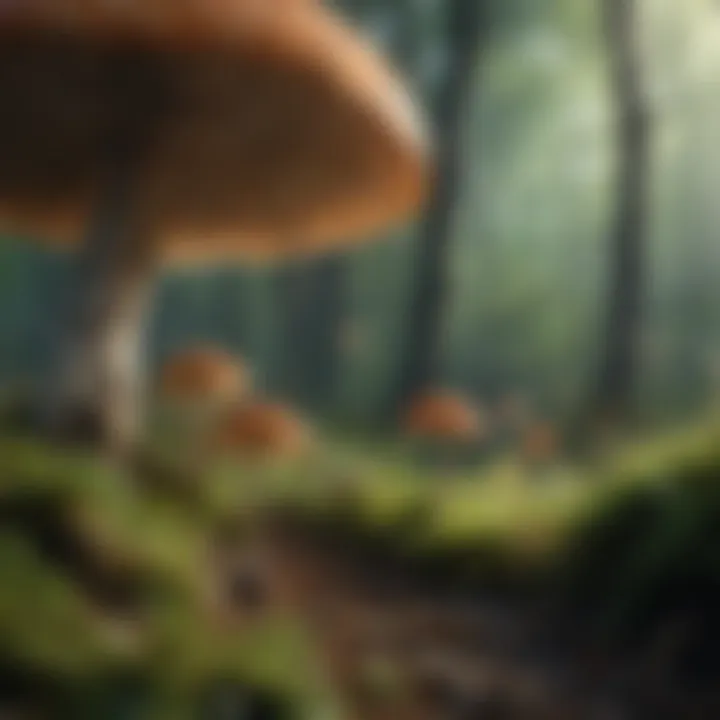
Mechanisms of Resistance
Antimicrobial resistance is a pressing concern that has emerged as we indiscriminately use antibiotics. Bacteria, in particular, possess unique mechanisms that allow them to survive in the presence of such medications. These strategies range from altering their cell walls to prevent drug entry to efflux pumps expelling the drug out. A well-known example is the ability of Staphylococcus aureus to produce beta-lactamase, an enzyme that attacks beta-lactam antibiotics. The importance of understanding these mechanisms cannot be overstated, as it provides insight into how we might combat resistant strains effectively.
The depth of knowledge surrounding mechanisms of resistance is vital in developing better treatment strategies and alternative antimicrobial agents. As an advantage, knowing these mechanisms can lead to tailored therapies, but on the flip side, it often means that treatment has to become more complex and individualized, thus increasing healthcare costs.
Implications for Public Health
The implications of antimicrobial resistance stretch globally, affecting public health systems and the efficacy of treatments. One key characteristic of these implications is the rise in infections that were once easily treatable, leading to longer hospital stays and higher mortality rates. This trend raises alarms about healthcare resources becoming strained, and in some cases, it leads to the re-emergence of diseases we thought eradicated. Addressing these implications is beneficial for maintaining public health standards and ensuring that common infections don't spiral out of control.
However, the harsh reality is that combating this resistance requires cooperation across various sectors โ healthcare, agriculture, and public policy. The unique challenge we face is balancing the urgency of developing new medications while implementing robust stewardship programs to limit inappropriate antibiotic use.
Impact of Climate Change
Effects on Ecosystems
Climate change stands to exert significant pressures on single cell organisms, many of which are already functioning within fragile ecosystems. Alterations in temperature and pH can impact metabolic rates and reproduction. For instance, higher temperatures can lead to increased bacterial growth, potentially resulting in harmful algal blooms.
Understanding the effects on ecosystems caused by climate change is essential for predicting future changes in biodiversity and the services ecosystems provide. The unique feature here is that single cell organisms form the backbone of many food webs. Any disruption at this level can have cascading effects throughout the ecosystem, leading to issues like nutrient imbalances or even loss of species.
Adaptation Strategies
To enable survival, many single cell organisms have developed adaptation strategies. For instance, some can form spores or go into a dormant state until conditions improve. Others adjust their metabolic processes to utilize available resources more effectively. This adaptability not only allows these organisms to persist but also shapes ecosystem resilience.
The notable benefit of such adaptation strategies is their ability to inform us about biological response mechanisms in diverse environments. However, the challenge remains that not all organisms will adapt equally, leading to potential losses in biodiversity as certain species may outcompete others.
Expanding the Microbiome Research
Importance of Human Microbiome
The human microbiome holds a pivotal role in health and disease, influencing everything from metabolism to immune responses. One striking aspect is the sheer diversity of microorganisms residing within us, which affects our bodily functions. Understanding the importance of human microbiome contributes to medical advancements, including personalized medicine and novel treatments. It elevates microbiology from a simple observational science to a field of immense significance in health.
However, the complexity of the interactions within the human microbiome also poses a challenge. Not all microbial interactions are beneficial, and understanding the balance is crucial to harnessing their potential positively in healthcare.
Future Research Avenues
As we pave pathways for microbial studies, future research avenues will emerge, focusing on the roles of individual microbial species and their interactions. Investigating how these microorganisms affect human health could lead to breakthroughs in treating metabolic disorders or autoimmune diseases. The prospect of utilizing advanced technologies, such as CRISPR, further adds to this potential.
While there are promising directions, hurdles remain, particularly regarding ethical guidelines and the translation of bench research into clinical practice. Thus, while the future seems brimming with promise, it is essential to tread carefully in the exploration of these uncharted territories.
The journey through challenges highlights the intricacies of single cell organisms, and it is through addressing these obstacles that we may find the keys to unlock their potential for bettering our world.
Closure
In wrapping up the exploration of single cell organisms, we must highlight the importance of their role in our understanding of life itself. This article has journeyed through the remarkable diversity of these organisms, showcasing how they exhibit unique adaptations, metabolic processes, and ecological functions that affect every corner of our biosphere.
Summary of Key Insights
Single cell organisms are not just microscopic agents; they are the bedrock of all life. Here are some of the pivotal points discussed in this article:
- Diversity and Classification: Ranging from bacteria to protozoa and fungi, these organisms showcase an astonishing variety. Each category comes with distinctive traits that allow them to thrive in various environments.
- Metabolic Roles: The unique metabolic pathways found in single cell organisms enable them to produce energy in diverse manners, from anaerobic processes to complex fermentation activities.
- Ecosystem Impact: They are essential players in nutrient cycling and maintaining the health of ecosystems. Their interactions with other life forms, like from the microbes to larger organisms, illustrate their significance in sustaining life.
- Research Applications: Advances in biotechnology and medicine hinge on harnessing these organisms, from antibiotic production to genetic engineering.
This holistic understanding allows scientists and researchers to grasp how these microscopic entities influence broader biological networks.
The Significance of Ongoing Research
Continued research into single cell organisms holds vast potential. The implications stretch far beyond academia into practical applications that can benefit society as a whole. Here are some critical considerations:
- Addressing Emerging Health Challenges: As antibiotic resistance becomes more prevalent, understanding the mechanisms of resistance in bacteria is crucial. Research could lead to new treatment strategies that effectively combat resistant strains.
- Understanding Environmental Shifts: Climate change threatens to alter ecosystems fundamentally. By studying how single cell organisms adapt, researchers can predict potential impacts on food webs and nutrient cycles, further enabling effective mitigation strategies.
- Expanding the Human Microbiome: The human microbiome is a treasure trove of insights. Ongoing studies may unravel links to health conditions and pave the way for personalized medicine.
In summing up, single cell organisms are the foundation of life, and understanding them grants us keys not only to current biological challenges but future opportunities to improve human health and environmental sustainability. Research in this field is not merely an academic pursuit; it is instrumental in shaping a better, more informed world.