Latest Insights and Developments in Dark Matter Research
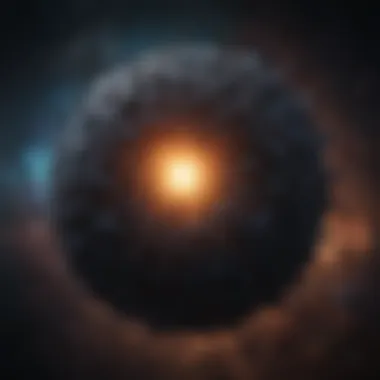
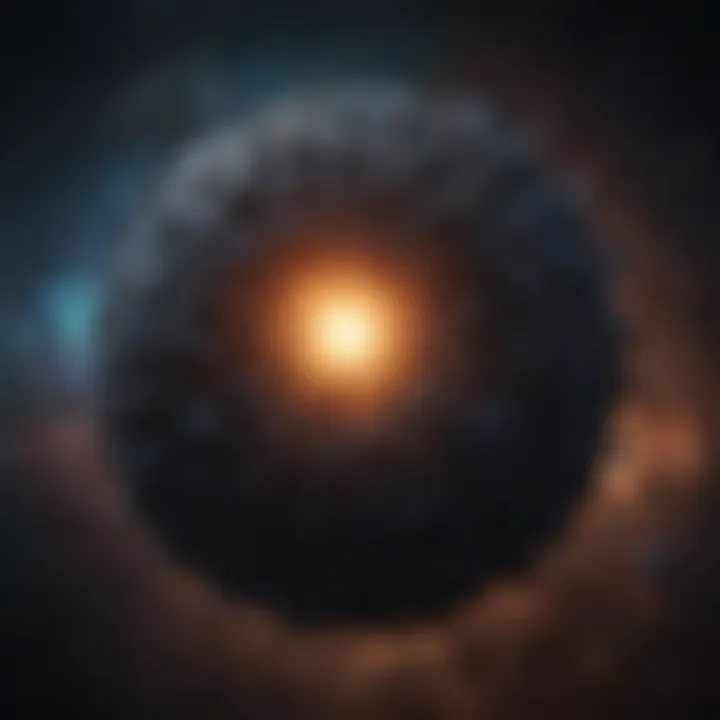
Intro
Dark matter remains one of the most captivating enigmas in the field of astrophysics. Its presence is inferred from various phenomena, yet it has not been directly observed. Recent research continues to unveil insights into dark matter's properties and its role in the cosmic framework. These discoveries are not only stimulating discussions in scientific circles, but they also challenge existing theories, pushing the boundaries of our understanding of the universe.
In this article, we will explore key research findings in dark matter studies, discuss the significance of those findings, and break down complex concepts to enhance comprehension. The implications of these studies stretch far beyond academic interest, as they may reshape how we perceive our universe.
Key Research Findings
Overview of Recent Discoveries
Recent explorations in dark matter research have led to several notable findings:
- Gravitational Lensing: Astronomers have utilized gravitational lensing effects to map dark matter distribution more accurately. This technique has provided insights into how dark matter interacts with visible matter.
- WIMP Candidates: Weakly Interacting Massive Particles, or WIMPs, have been a significant focus. New experiments, such as those conducted by the Large Hadron Collider, aim to detect these elusive particles, which could help explain the dark matter abundance in the universe.
- Galaxy Formation: Studies have shown that dark matter significantly influences the formation and evolution of galaxies. The Cosmic Microwave Background radiation has provided indirect evidence of dark matter's role in shaping the universe's large-scale structure.
These discoveries contribute to the ongoing quest to understand dark matter and its function in the universe.
Significance of Findings in the Field
The implications of these findings are profound:
- Understanding Cosmic Structure: Insights into dark matter help clarify how galaxies and galaxy clusters form and evolve over time. This understanding is crucial for cosmology.
- New Physics: Discovering the exact nature of dark matter could potentially lead to breakthroughs in physics beyond the Standard Model. This could change our perception of fundamental particles and forces.
- Technological Advancements: Research into dark matter detection drives technological innovation. Enhanced sensitivity in detection methods spurs developments that may have applications beyond astrophysics.
Breakdown of Complex Concepts
Simplification of Advanced Theories
Many theories regarding dark matter are intricate, making them challenging to grasp:
- Supersymmetry: Some theories suggest supersymmetry might offer candidates for dark matter particles. In this framework, each known particle has a heavier counterpart.
- Modified Gravity Theories: Some researchers propose modifications to Einstein's General Relativity to explain phenomena usually attributed to dark matter. These alternative theories may have significant implications on our understanding of gravity.
It is essential to distill these concepts into accessible explanations that resonate with both experts and newcomers in the field.
Visual Aids and Infographics
Using visual aids can greatly enhance understanding:
- Diagrams of Gravitational Lensing: Showing how light bends around massive objects can clarify how we infer dark matter's presence.
- Charts on Particle Detection Rates: Graphs demonstrating the results from labs, such as the Cryogenic Underground Observatory for Gravitational Waves, can help visualize the ongoing efforts in detection.
Such resources make the material more engaging and facilitate comprehension among various audiences.
"Understanding dark matter is crucial, not just for cosmology, but for the overarching narrative of our universe. Without it, many of our current theories lack cohesion."
Prologue to Dark Matter
Dark matter represents one of the most intriguing and complex aspects of modern astrophysics. Its study is fundamental to understanding the universe's structure and behavior. This dark, invisible substance, making up approximately 27% of the universe, plays a critical role in shaping galaxies and cosmic architecture. Therefore, grasping the intricacies of dark matter is vital for researchers, students, and educators alike.
Definition and Importance
The term "dark matter" refers to a type of matter that does not emit, absorb, or reflect light. As a consequence, it cannot be observed directly using traditional astronomical methods. Instead, its existence is inferred through its gravitational effects on visible matter, such as stars and galaxies. This unobserved mass is pivotal in explaining phenomena that visible matter alone fails to account for, such as the rotational speeds of galaxies and the cosmic background radiation.
Understanding dark matter is essential for several reasons:
- Cosmological Significance: It influences the formation and evolution of galaxies, directly impacting cosmological models.
- Scientific Inquiry: Investigating dark matter leads to potential new physics beyond the Standard Model.
- Technological Development: The efforts to detect dark matter have spurred advancements in technology and methodology across various scientific disciplines, enhancing overall research capabilities.
In light of these factors, the definition of dark matter goes beyond mere classification. It encompasses a significant avenue of inquiry in both theoretical and experimental physics, shaping our understanding of the universe's nature.
Historical Context
The quest to understand dark matter is not recent; it spans several decades. The initial hints of dark matter emerged in the 1930s when Swiss astrophysicist Fritz Zwicky studied galaxy clusters in the Coma cluster. He noticed that the mass derived from the visible matter was insufficient to account for the observed gravitational binding of these galaxies. He proposed that an unseen mass was present, coining the term "dark matter."
Later in the 1970s, Vera Rubin's work on galaxy rotation curves offered additional evidence. Rubin noted that the outer regions of galaxies were rotating at speeds that contradicted expectations based on visible mass alone. This discrepancy pointed strongly to the existence of unseen mass concentrated in and around galaxies.
Since then, various theoretical frameworks, such as the Lambda Cold Dark Matter model, have emerged to explain dark matter's properties and effects. The historical progression of dark matter research reveals a fascinating journey through scientific discovery, fueled by curiosity and innovation. Each step has paved the way for the advanced theories and research highlighted in this article.
Current Theories
The study of dark matter is fundamentally shaped by the prevailing theories that attempt to explain its nature and behavior. Understanding these theories is crucial, as they form the backbone of ongoing research and experimentation. Current theories not only seek to identify the constituent particles of dark matter but also attempt to explain the effects observed in the universe that can be attributed to its existence. Each theory brings its own set of implications, challenges, and experimental avenues to explore. Therefore, an analysis of these contemporary concepts is essential for anyone engaged in cosmology or particle physics.
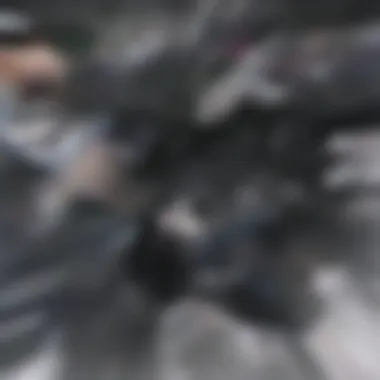
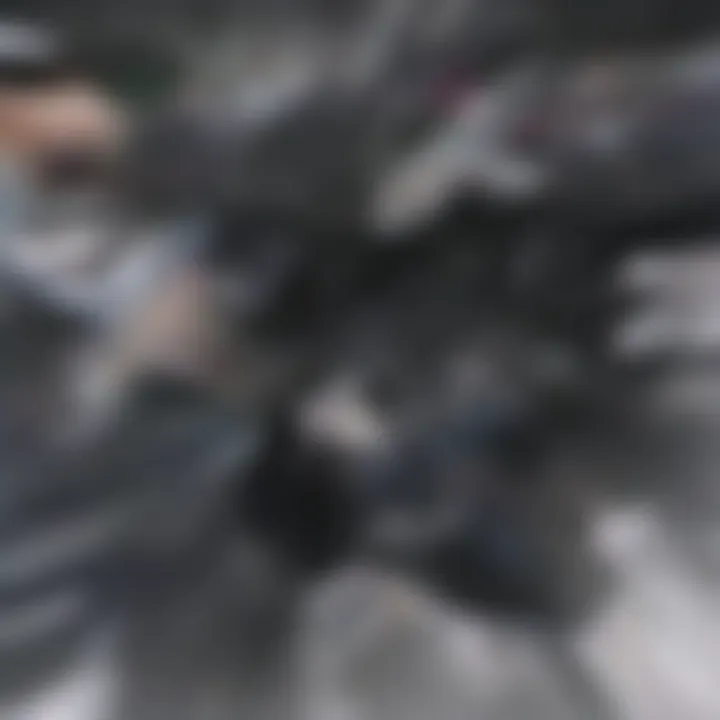
WIMPs (Weakly Interacting Massive Particles)
WIMPs are one of the leading candidates for dark matter. They are hypothetical particles that are predicted to interact via the weak nuclear force and gravity. This makes them less detectable than ordinary matter but still significant in understanding the universe's structure and formation. Being massive, WIMPs could account for a substantial fraction of dark matter. Their interactions, although weak, could eventually lead to detectable signals in various experiments.
Several experiments are currently underway to detect WIMPs directly, such as the LUX-ZEPLIN project and the PandaX experiment. These projects aim to observe WIMP interactions with nuclei in ultra-pure environments. The significance of WIMPs lies not only in their potential detection but also in the clues they can provide about the fundamental nature of the universe. As physicists continue to probe deeper into these interactions, we may find critical insights regarding particle physics and the behavior of the cosmos.
Axions and Other Candidates
Axions present another intriguing possibility in the quest to understand dark matter. They are hypothetical particles proposed to resolve the strong CP problem in quantum chromodynamics. Similar to WIMPs, axions are expected to be very light and weakly interacting, transforming them into viable candidates for dark matter.
Researchers have designed various experiments to detect axions. The Axion Dark Matter Experiment (ADMX) is one such initiative that looks for axion-to-photon conversion in strong magnetic fields. The exploration of axions could transcend traditional particle theories, highlighting the interplay of fundamental forces at play. Moreover, other candidates like sterile neutrinos and various supersymmetric particles have been proposed, each offering unique implications for our understanding of dark matter. The ongoing research into these candidates will either fashion a new narrative for dark matter or reinforce existing ones.
Modified Gravity Theories
Modified gravity theories challenge the conventional understanding of gravity as described by Einstein's General Relativity. Instead of positing the existence of dark matter particles, these theories suggest that the effects attributed to dark matter may arise from modified laws of gravity.
Notable examples include MOND (Modified Newtonian Dynamics) and TeVeS (Tensorβvectorβscalar gravity). These theories aim to explain observed cosmic phenomena β such as galaxy rotation curves β without invoking dark matter. While they have garnered both interest and skepticism, the significance of modified gravity theories lies in their potential to redefine how we model cosmic dynamics. Their advocates argue that understanding gravity's role may open new doors to comprehend dark matter's implications on cosmic scales.
In summary, the exploration of WIMPs, axions, and modified gravity theories represents a significant aspect of dark matter research. Each brings a unique perspective and highlights the ongoing endeavors within the scientific community to uncover the universe's mysteries. As these concepts advance through experiments and theoretical developments, they continue to shape our understanding of the cosmos.
Recent Discoveries
Recent discoveries in dark matter research have propelled the field into new territory. They are crucial in understanding how dark matter interacts with regular matter and influences the universe's structure. Insights gleaned from these findings shape theories and inform future experiments, highlighting key elements that demand continued investigation. The significance of these discoveries is twofold: they augment our knowledge of cosmology and push technological advancements in detection methods.
Breakthrough Experiments
Fermi Gamma-ray Space Telescope
The Fermi Gamma-ray Space Telescope has made notable contributions to dark matter research, especially through its ability to survey the entire sky for high-energy gamma rays. One key aspect is its potential to detect signals from dark matter annihilation. Fermi's unique capability to analyze large volumes of data and identify gamma-ray emissions allows researchers to probe regions in space that could contain dark matter, providing indirect evidence of its presence.
The telescope's sensitivity to different gamma-ray energies makes it a favored tool in the investigation of dark matter candidates. Its level of detail can elucidate structures in cosmic rays and provides indirect evidence by measuring excess emissions in specific regions, such as the center of the Milky Way.
However, while Fermi brings significant advantages, challenges arise. Analyzing the data requires careful interpretation, since signals could also stem from cosmic phenomena other than dark matter. The collaboration of the astrophysical community plays a vital role in navigating these complexities of data analysis and signal interpretation.
XENON1T Results
XENON1T serves as a pivotal experiment in direct dark matter detection. Its main strength lies in its ultra-sensitive detection of weakly interacting massive particles. Through an extensive underground setup, XENON1T has measured cosmic rays and the faint signals resulting from a potential dark matter interaction. Its ability to operate in a controlled environment minimizes background noise, enhancing detection capabilities.
Recently, the XENON1T experiment generated headlines with its results suggesting hints of potential dark matter signals. This has stirred discussions about the existence and nature of dark matter particles. The unique feature of XENON1T lies in its use of a liquid xenon target, which provides superior sensitivity compared to other methods, allowing researchers to probe lower mass ranges of dark matter particles.
Nonetheless, confirming dark matter signals from XENON1T remains contentious, with scientists debating if the observed anomalies result from actual dark matter interactions or unforeseen environmental factors. Rigorous analysis continues, heralding the importance of straightforward yet cautious interpretations of experimental outcomes.
Observational Evidence
Galaxy Rotation Curves
Galaxy rotation curves have become a cornerstone in dark matter research. These curves illustrate the velocity of stars orbiting the center of galaxies and serve as a critical evidence line for dark matter's existence. Observations highlight that stars far from the galactic center move at unexpectedly high speeds compared to predictions made with visible matter alone. Such discrepancies strongly suggest the presence of substantial unseen mass, which is interpreted as dark matter.
The prominence of galaxy rotation curves in research results from their straightforward observational approach. They provide a clear method to infer the overall mass distribution within galaxies without the assumption of dark matter's properties. However, interpretation remains complex, as it invites debates about modifications to gravitational theory and other explanations.
Gravitational Lensing Studies
Gravitational lensing studies further substantiate the dark matter hypothesis through the study of light bending around massive objects. This technique allows scientists to observe how the gravity of a foreground object distorts the light coming from background objects. The ability to map dark matter's distribution from these distortions has proven tremendously beneficial for researchers aiming to understand cosmic structure's evolution.
One unique feature of gravitational lensing is its applicability across vast scalesβfrom galaxy clusters to individual galaxies. It highlights regions where dark matter concentrations are expected to exist. This method's strength lies in its reliance on general relativity, providing a robust framework for understanding gravitational effects.
However, gravitational lensing requires extensive data collection and sophisticated modeling to interpret the results accurately. The challenge lies in disentangling complex astrophysical parameters, making this work a collaborative effort among astrophysicists, mathematicians, and astronomers to refine the models used.
Recent advancements in dark matter research, including breakthrough experiments and observational evidence, illustrate the evolving nature of this field and highlight the necessity for interdisciplinary cooperation.
Technological Innovations
Technological innovations play a crucial role in dark matter research as they facilitate new approaches to detecting and understanding this enigmatic substance. As researchers explore the universe's mysteries, advancements in detection technologies have proven fundamental in gathering data. They also allow scientists to test existing theories and develop new ones, increasing the potential for significant breakthroughs.
Advancements in Detection Technology
Direct Detection Methods
Direct detection methods aim to identify dark matter by observing its interactions with regular matter. The significance of this technique lies in its ability to provide firsthand evidence of dark matter particles. Particularly, these methods employ sensitive detectors located deep underground or in space to minimize background noise.
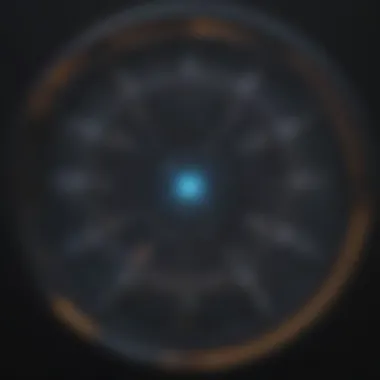
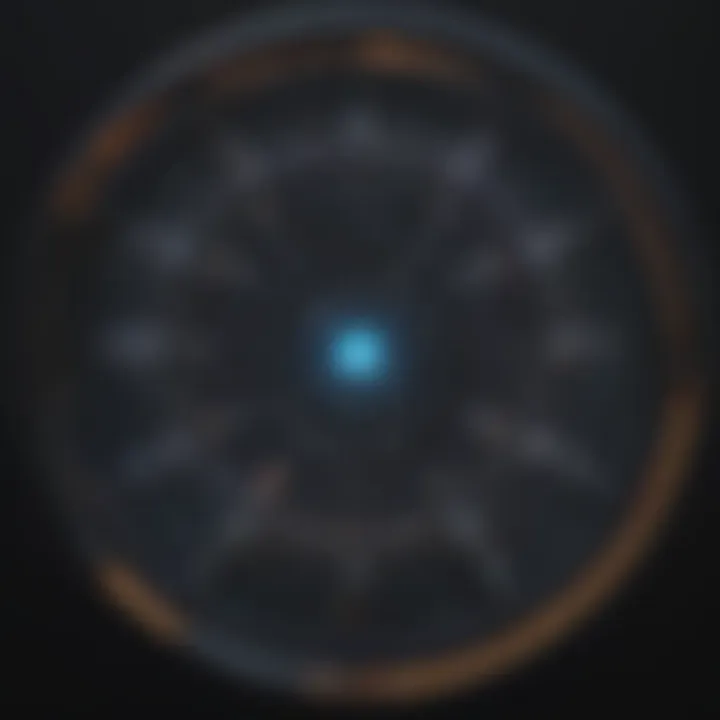
A key characteristic of direct detection systems is their ability to measure energy deposits caused by potential dark matter collisions. This unique feature offers high precision and a direct semblance to particle physics experiments, making it a popular choice among researchers. Notable projects like the LUX-ZEPLIN experiment utilize these methods to search for weakly interacting massive particles (WIMPs).
However, direct detection methods also face challenges. The rarity of dark matter interactions makes these measurements exceedingly difficult. Additionally, the technology involved is complex and expensive, limiting the number of experiments that can be conducted.
Indirect Detection Techniques
Indirect detection techniques focus on observing the byproducts of dark matter interactions, which occur when dark matter particles collide or decay. This approach is essential for uncovering the presence of dark matter without relying on direct interactions.
A primary advantage of indirect detection methods is their ability to utilize existing astronomical observations. This characteristic allows researchers to analyze cosmic rays, gamma rays, and neutrinos for signals that could indicate dark matter activity. The Fermi Gamma-ray Space Telescope, for example, plays a vital role in this aspect by monitoring gamma-ray emissions from potential dark matter annihilation.
Nonetheless, indirect detection techniques come with their own limitations. The signals can often be difficult to distinguish from other cosmic phenomena, leading to uncertainties in data interpretation. Furthermore, the correlation of detected signals to dark matter interactions is not always straightforward, posing additional challenges to researchers.
Computational Models and Simulations
Computational models and simulations are indispensable in dark matter research. They allow scientists to simulate the behavior of dark matter under various cosmological conditions. Through these models, researchers can explore how dark matter influences the structure and evolution of the universe.
One of the critical benefits of using simulations is their ability to generate predictions about observable cosmic structures. This capability helps lay the groundwork for future observational campaigns that can test these predictions against actual galactic formations or phenomena. Using intricate algorithms, such as those found in the Illustris project, extensive simulations aid scientists in visualizing complex interactions that are impossible to replicate in laboratory settings.
The continuous enhancement of computer processing power further enables increasingly sophisticated models. However, the reliance on simulations also brings a degree of uncertainty, as results are only as accurate as the underlying assumptions. Furthermore, discrepancies between simulations and observational data may lead to revisions in the models, emphasizing the intricate relationship between theory and practice in this field.
Challenges in Dark Matter Research
The exploration of dark matter presents numerous challenges that are critical to our understanding of the universe. These challenges are deeply interwoven with the complexity of dark matter itself. Addressing these hurdles is essential for future breakthroughs. Researchers face intricate issues, ranging from data interpretation difficulties to funding constraints, both of which significantly impact progress in the field.
Data Interpretation Issues
One of the most significant challenges in dark matter research is the interpretation of data. Observational evidence often arrives from indirect measurements, leading to uncertainties. The data collected from various astronomical phenomena must be meticulously analyzed to extract meaningful conclusions about dark matter's characteristics.
For instance, galaxy rotation curves imply vast amounts of unseen mass, suggesting the presence of dark matter. However, unexpected variations can lead to conflicting results. Different models may explain the same data, creating a dilemma for scientists. It raises questions about the fidelity of existing models and the necessity for new ones. Thus, researchers must apply rigorous statistical techniques and continuously refine theoretical frameworks to ensure that interpretations remain valid.
Moreover, data noise from instruments can obscure real signals of dark matter interactions. Detecting these subtle signals often requires advanced algorithms and deep understanding of astrophysics.
Funding and Resource Allocation
Funding and resource allocation represent another major challenge in dark matter research. As with many areas in scientific inquiry, limited budgets can restrict the scope of projects and experiments. Funding bodies often prefer projects with immediate application or likelihood of success, which can sideline lesser-known yet crucial areas like dark matter studies.
The need for sophisticated equipment and extensive datasets leads to increased costs. Facilities such as the Large Hadron Collider or advanced telescopes require significant investments, making research access unequal. Limited funding may hinder innovative projects or delay timelines, ultimately affecting the speed of discovery in the field.
Researchers may also find that the competition for grants is fierce, leading to a focus on mainstream topics rather than exploring fringe theories or untested ideas. Consequently, it becomes hard to sustain long-term investigation in this enigmatic field.
"Securing adequate funding is vital not only for research but also for nurturing the next generation of scientists in dark matter inquiry."
Overall, overcoming these challenges is imperative to advance our understanding of dark matter. The interpretation of data must be refined, and adequate funding allocated to support critical research initiatives. Only then can a clearer picture of dark matter emerge from the shadows.
Implications for Cosmology
Understanding dark matter is crucial for the study of cosmology. It plays a significant role in shaping our universe, influencing the formation and evolution of galaxies. This section delves into how dark matter impacts cosmology, focusing on specific elements like galaxy formation and the cosmic microwave background.
Impact on Galaxy Formation
Dark matter serves as a framework for galaxies, providing the gravitational forces necessary for their creation. In the early universe, after the Big Bang, dark matter existed in the form of halos. These halos acted as gravitational wells, drawing in normal matter to form stars and galaxies. The presence of dark matter determines the distribution of galaxies across the cosmos. Without it, the universe would not look as it does today.
Observations of galaxies reveal that they rotate faster than what would be expected based on visible matter alone. This discrepancy is attributed to the invisible mass of dark matter surrounding them. Studies of galaxy clusters further support this notion, demonstrating that their mass cannot be accounted for by observable matter alone.
"The role of dark matter in galaxy formation is akin to invisible scaffolding, allowing structures to grow and evolve in a gravitational dance."
Further research continues to refine our understanding of how dark matter influences mass distribution and galaxy morphology. Insights gained from simulations provide clues about the complex mechanics of gravitational interactions driven by dark matter.
Influence on Cosmic Microwave Background
The cosmic microwave background (CMB) provides vital information about the early universe. It is the afterglow of the Big Bang, revealing how matter and energy were distributed shortly after the event. Dark matter significantly influences the CMB through its gravitational effects and density fluctuations.
When the universe was dense and hot, dark matter and ordinary matter existed within a sea of photons. As the universe expanded and cooled, these elements began to decouple. The density variations in dark matter created gravitational potentials that affected the motion of ordinary matter and photons. This created the anisotropies observed in the CMB today.
The study of CMB allows scientists to measure the density of dark matter relative to normal matter in the universe. These measurements inform cosmological models, affecting our understanding of the universeβs structure, expansion rate, and ultimate fate.
For more detailed insights, consider looking up additional resources like Wikipedia or Britannica.
Interdisciplinary Perspectives
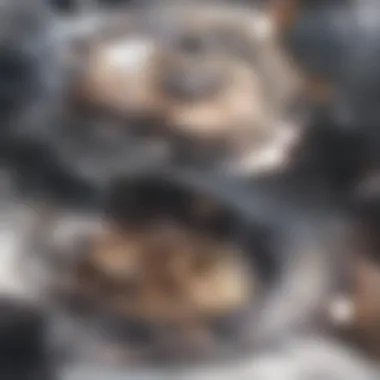
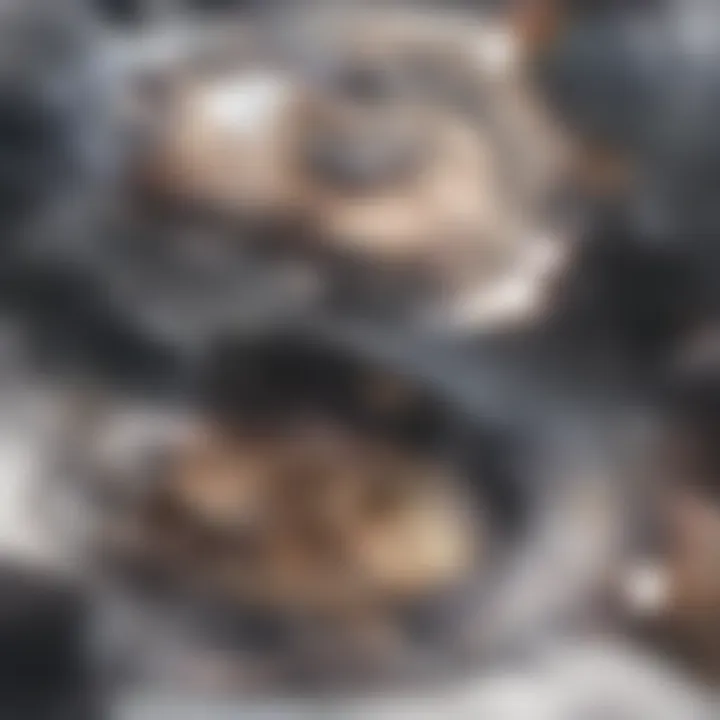
Given the complex nature of dark matter, an interdisciplinary approach is vital for advancing research in this field. Dark matter research intersects several scientific disciplines, including physics, astronomy, and mathematics. Each discipline contributes unique methodologies, theoretical frameworks, and insights that enrich our understanding of dark matter. The benefits of these interdisciplinary perspectives cannot be overstated, as they foster collaboration that can lead to innovative solutions for existing challenges in dark matter research.
One of the primary elements of this collaboration involves shared resources and expertise. For instance, physicists can design experiments to test various hypotheses about dark matter, while astronomers provide observational data about the cosmos. This synergy often results in a more holistic understanding of the universe, helping researchers to identify dark matter's behavior and role in galaxy formation.
Moreover, interdisciplinary studies enable scientists to tackle complex problems that are beyond the scope of a single field. Researchers can draw upon statistical methods from mathematics to analyze large datasets generated by astronomical observations or experimental results. By employing various mathematical models, researchers can simulate dark matter scenarios and predict outcomes that can be tested empirically.
In addition to practical benefits, interdisciplinary perspectives also encourage innovative thinking. By integrating concepts from different fields, scientists are more likely to challenge existing paradigms and propose novel theories regarding dark matter's nature. Such creative approaches can lead to breakthroughs, enhancing our overall comprehension of this elusive substance.
In summary, fostering interdisciplinary perspectives in dark matter research facilitates numerous advantages. It enables collaborative efforts between diverse scientific fields, enhances problem-solving capabilities, and promotes innovative ideas. This holistic approach is essential for unraveling the mysteries of dark matter, extending our grasp of the universe.
Collaboration Between Physics and Astronomy
Collaboration between physicists and astronomers is essential in dark matter research. The physics community often focuses on theoretical aspects of dark matter, such as particle candidates like WIMPs and axions. In contrast, astronomers provide crucial observational evidence about the cosmos, carrying out studies like gravitational lensing and galaxy rotation curves. These observations are fundamental for validating or refuting theoretical models proposed by physicists.
Through collaborative projects, researchers can design experiments that directly test dark matter theories against astronomical data. For instance, astrophysics experiments such as the XENON1T aim to detect dark matter interactions based on theoretical predictions. Additionally, telescopes like the Fermi Gamma-ray Space Telescope assist in tracing high-energy phenomena linked to dark matter.
This interaction among the disciplines allows a richer exchange of information and stimulates the progression of knowledge.
Role of Mathematics in Modeling
Mathematics plays a critical role in modeling dark matter phenomena. Researchers utilize mathematical frameworks to create models that describe the influence of dark matter on cosmic structures. These models help predict celestial behaviors and interactions that align with observational data.
Mathematical modeling serves several purposes:
- Simulation of Dark Matter Dynamics: Using numerical simulations, scientists can replicate the effects of dark matter on galaxy formation and evolution, enabling a deeper understanding of its role in the universe.
- Statistical Analysis: Advanced statistical techniques allow researchers to analyze data sets for patterns and anomalies associated with dark matter.
- Theoretical Frameworks: Mathematics helps formulate theoretical concepts that explain dark matter's properties and interactions, providing a foundation for experimental design.
In light of these functions, mathematics not only supports but enhances astrophysical research in dark matter. By connecting theoretical models with empirical observations, mathematics paves the way for exciting discoveries.
Future Directions in Research
The future of dark matter research holds significant promise. As scientists delve deeper into the cosmos, new theories and investigations are emerging. The understanding of dark matter continues to evolve, demanding fresh perspectives and innovative approaches. This article examines crucial topics related to future directions, specifically emerging theories and concepts along with predictions and future experiments. Both areas are vital for advancing knowledge in this intriguing field.
Emerging Theories and Concepts
Recent advances have led to varied emerging theories regarding dark matter. The scientific community is increasingly considering alternatives to traditional views. One notable theory is the Self-Interacting Dark Matter (SIDM), which proposes that dark matter could have strong interactions with itself. This might help explain certain astrophysical observations that do not align well with the current understanding of dark matter interactions.
Another exciting avenue includes the Emerging Quantum Field Theories, which hint at new interactions of dark matter particles in a quantum realm. This can lead to profound shifts in understanding not just dark matter, but also its implications in the overall structure of matter in the universe.
Moreover, the idea of Composite Dark Matter suggests that dark matter might not be a fundamental entity but instead consists of more basic components. Researchers are keenly exploring how these concepts intertwine with cosmic structures. The continued exploration of these theories can illuminate the nature of dark matter, potentially leading to groundbreaking discoveries that reshape our comprehension of the universe.
Predictions and Future Experiments
Looking ahead, the scientific community is gearing up for critical experiments that could clarify dark matter's elusive properties. The Large Hadron Collider (LHC) remains a focal point. Scientists aim to discover potential dark matter candidates by colliding particles at unprecedented energies. Such interactions could provide evidence for the existence of new particles or forces beyond the Standard Model, significantly impacting our understanding.
In addition, next-generation observatories, like the Cherenkov Telescope Array, aim to capture cosmic rays more effectively. They will also explore high-energy gamma rays possibly originating from dark matter interactions. Likewise, the PandaX experiment seeks to detect dark matter directly, offering another crucial method to test hypotheses and reveal insights.
Furthermore, advances in gravitational wave astronomy may open doors to new detection mechanisms. As events such as black hole mergers are observed, they can enhance understanding of how dark matter influences gravity and the structure of the universe.
Science is on the brink of major tenets that could drive the future of dark matter studies. By synthesizing emerging theories with concrete experimental efforts, researchers will progressively unravel the complexities of dark matter, bringing clarity to one of the universe's greatest mysteries.
Public Engagement and Education
Public engagement and education are vital components of advancing knowledge in dark matter research. The complexity and abstract nature of dark matter can alienate the wider public. Hence, it is crucial to make this field accessible and to foster understanding. Effective communication can amplify interest and investment in scientific inquiry.
Several specific elements contribute to the importance of public education in dark matter research:
- Understanding Scientific Concepts: Dark matter research involves intricate theories and models. Making these concepts more approachable enhances public comprehension.
- Encouraging Future Scientists: Engaging young minds can inspire the next generation of physicists and astronomers. Early exposure to such topics can spark curiosity and passion for science.
- Promoting Funding and Support: Public awareness can lead to increased funding and resources for research. When people understand the significance of dark matter, they may advocate for its prioritization in scientific inquiry.
- Building Community: Education initiatives can create a community of interested individuals. This can facilitate discussions and innovations from diverse perspectives.
In summary, public engagement and education around dark matter serve not just to inform, but also to inspire and mobilize action for further exploration of the universe.
How to Make Dark Matter Accessible
To enhance accessibility, various strategies can be implemented. The goal is to simplify without trivializing complex ideas. Here are methods to consider:
- Use of Visual Aids: Infographics and videos can present complicated subjects in an engaging way.
- Workshops and Lectures: Organizing sessions in schools and community centers encourages direct interaction with scientists.
- Interactive Online Courses: Offering structured learning experiences can allow individuals to explore dark matter topics at their own pace.
- Hands-on Activities: Simple experiments that illustrate fundamental concepts can be effective teaching tools for younger audiences.
Through these efforts, dark matter can become a more familiar topic. A mindset shift will occur as the community begins to appreciate its importance within the cosmos.
Role of Online Platforms and Media
The role of online platforms and media in dark matter education is increasingly significant. They provide a reach that traditional methods may not. Digital platforms offer unique opportunities to disseminate knowledge.
A few key points include:
- Widespread Access: Websites, social media, and video sharing platforms can reach viewers globally, transcending geographical barriers.
- Engaging Formats: Podcasts and interactive content allow for varied learning styles. This can be especially effective in attracting younger audiences.
- Real-Time Updates: Online media can instantly report breakthroughs in research. This keeps the public informed and engaged with current developments.
- Community Interaction: Forums and social media groups encourage discussions. This creates a space for shared learning and inquiry.