Breakthroughs in Genetic Engineering: Shaping Science
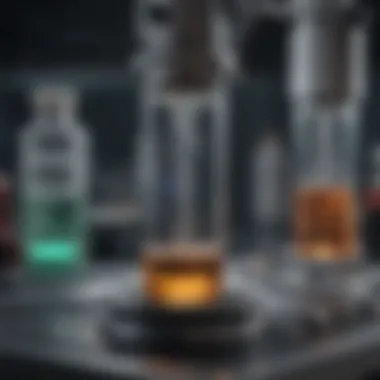
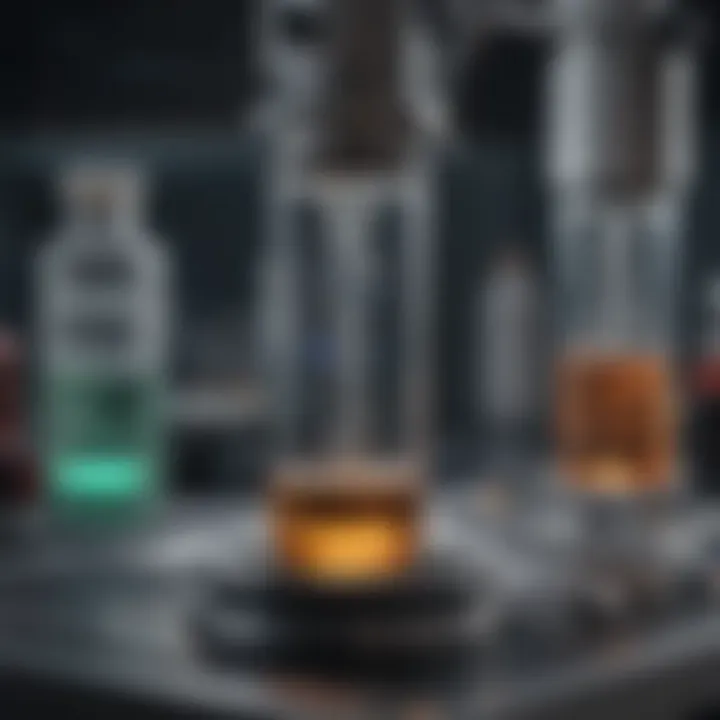
Intro
Genetic engineering represents a paradigm shift in how we understand and manipulate the building blocks of life. Recent advancements have significantly changed the landscape of this field, leading to novel applications that were once thought impossible. This article will explore key breakthroughs in genetic engineering, revealing their profound implications for science and society.
The integration of specific technologies has allowed researchers to edit genes with a level of precision that was previously unattainable. These innovations present exciting possibilities in various domains, including medicine and agriculture. However, as we delve into the remarkable accomplishments, it is also crucial to address the ethical dimensions surrounding genetic modification.
This examination will illuminate the transformative potential of genetic engineering while ensuring a balanced view that considers the accompanying moral questions. In doing so, the discussions will focus on the importance of understanding both the advancements and the implications tied to them.
Intro to Genetic Engineering
Genetic engineering is a pivotal field that reshapes our understanding of biology, medicine, and agriculture. The advances in this area have profound implications. Genetic engineering is not simply about modifying organisms. It presents a chance to address long-standing issues, such as genetic disorders, food security, and disease control. As researchers delve deeper, new methodologies emerge, pushing the boundaries of what is possible.
Historical Background
The roots of genetic engineering can be traced back to the early 20th century. Researchers started uncovering the basics of heredity, laying the foundation for genetic manipulation. In the 1950s, the structure of DNA was discovered by James Watson and Francis Crick. This breakthrough provided a crucial understanding of how genes work. In the following decades, scientists developed various techniques, such as recombinant DNA technology, enabling them to combine genes from different organisms. This marked the beginning of a new era in genetic engineering.
"The modern era of genetic engineering began with the discovery of recombinant DNA techniques in the 1970s."
By the late 20th century, the Human Genome Project took shape, aimed at mapping all human genes. The completion of this project in 2003 opened new frontiers in understanding genetic diseases and has set the stage for further advancements.
Definition and Scope
Genetic engineering refers to a set of technologies used to change the genetic makeup of organisms. It involves various techniques, including gene editing, cloning, and transformation. The scope of genetic engineering extends across multiple domains—medicine, agriculture, industrial biotechnology, and environmental applications.
In medicine, for example, genetic engineering plays a crucial role in developing gene therapies for conditions like cystic fibrosis and certain cancers. In agriculture, engineered crops can resist pests or tolerate harsh environmental conditions. This versatility makes genetic engineering a transformative science.
In summary, genetic engineering encourages exploration and innovation, revealing its potential to solve critical issues in society. As the article unfolds, it will delve into specific mechanisms, recent breakthroughs, and the ethical considerations surrounding these advancements.
The Mechanisms of Genetic Engineering
Understanding the mechanisms of genetic engineering is crucial for informing its applications and potential impact on society. This section explores specific gene editing techniques and cloning processes that are foundational to genetic manipulation. Each technique offers distinct advantages and limitations, influencing the course of scientific inquiries and innovations.
Gene Editing Techniques
Gene editing is at the forefront of genetic engineering. It allows for precise alterations to DNA sequences, leading to significant advances in various fields, including medicine and agriculture. Each technique in this domain has unique characteristics that shape its suitability for different applications.
CRISPR-Cas9
CRISPR-Cas9 represents a revolutionary advancement in gene editing. This technology utilizes a guide RNA to identify the target DNA sequence, where the Cas9 enzyme induces a double-strand break. This ability to make alterations at specific locations in the genome is what makes CRISPR-Cas9 so impactful. Its key characteristic is precision. Researchers appreciate that it can be utilized across various organisms, making it versatile.
The unique feature of CRISPR-Cas9 lies in its adaptability. Scientists can engineer guide RNAs for specific genes, facilitating targeted modifications. However, this precision comes with some disadvantages. Off-target effects, where unintended genetic changes occur, remain a concern. Moreover, ethical implications surrounding its use in germline editing are still being debated.
TALENs
Transcription Activator-Like Effector Nucleases (TALENs) are another important method for gene editing. This technique employs protein-based approaches to target specific DNA sequences. Its key characteristic is the use of tailored proteins that bind to DNA and create breaks, allowing for new genetic material to be inserted.
The unique feature of TALENs is their customizability. Each TALEN can be designed for a specific DNA sequence. This characteristic allows them to navigate complex genomic environments effectively. However, compared to CRISPR-Cas9, TALENs are often considered more cumbersome, as they require intricate protein engineering which can make their application more complex and costly.
ZFN
Zinc Finger Nucleases (ZFNs) comprise another gene editing tool. These proteins recognize specific DNA sequences to induce cuts, similar to TALENs. The key characteristic of ZFNs is their long history of use in genetic engineering, making them a time-tested choice.
A unique feature is their ability to target multiple genes simultaneously. This characteristic enables complex modifications to be carried out effectively. However, like TALENs, ZFNs require significant design work for custom applications. Their effectiveness relies heavily on the successful binding of the zinc finger protein to its target site, presenting a challenge that can complicate outcomes in practical scenarios.
Cloning and Transformation
Cloning is the process of creating copies of organisms or cells. In the context of genetic engineering, it plays a vital role in generating organisms with desired traits. Transformation, which often follows cloning, involves introducing foreign DNA into a host organism. This allows for precise genetic modifications that can be expressed in the recipient's phenotype.
By combining cloning and transformation approaches, scientists can explore new frontiers in genetic engineering, enhancing existing practices and driving research into innovative territories. The exploration of these mechanisms not only adds depth to the field but also defines the frameworks within which future research and applications will evolve.
Recent Breakthroughs in the Field
Recent advancements in genetic engineering represent a pivotal shift in scientific capability, impacting various disciplines. This segment emphasizes the significance of breakthroughs that not only improve health outcomes but also enhance food production methods and sustainability practices. The evolution of technologies, like gene editing and genomic analysis, showcases the potential of genetic engineering to tackle pressing challenges facing humanity today.
Applications in Medicine
Gene Therapy
Gene therapy is a revolutionary approach with the aim of treating genetic disorders by directly modifying genes. The primary characteristic of gene therapy is its potential to address the root cause of diseases rather than just managing symptoms. For instance, its application in hereditary conditions like cystic fibrosis and hemophilia has demonstrated substantial improvement in patients' quality of life.
A unique feature of gene therapy is its ability to introduce healthy copies of genes into affected cells. This technique can lead to long-lasting effects, reducing the need for frequent treatments. However, there are challenges to consider, such as the potential for immune reactions and the complexity of delivering the therapeutic agents effectively to target tissues.
Personalized Medicine
Personalized medicine tailors medical treatment to individual characteristics of each patient, often based on genetic information. It stands out as a crucial advancement in healthcare, allowing for more effective treatment plans and better patient outcomes. By utilizing genetic profiling, healthcare providers can predict how patients will respond to specific medications. This aspect is especially beneficial in oncology, where targeted therapies can significantly increase survival rates.
The distinct feature of personalized medicine lies in its focus on the individual rather than a one-size-fits-all approach. However, this method also comes with disadvantages. The high cost of genetic testing and the complexity of obtaining accurate data can present hurdles. Additionally, ethical concerns regarding genetic data privacy must be addressed.
Advancements in Agriculture
GMO Crops
Genetically modified organism (GMO) crops play a vital role in enhancing agricultural productivity and addressing food security. These crops are engineered to possess desirable traits, such as increased resistance to pests and tolerance to harsh environmental conditions. One key characteristic of GMO crops is their capacity to yield more food per acre compared to traditional crops, which is essential as the global population continues to grow.
A unique feature of GMO crops is the ability to decrease reliance on chemical pesticides, promoting more sustainable farming practices. However, there are debates about their long-term ecological impacts. Concerns over biodiversity loss and potential health issues related to GMO consumption persist, warranting thorough investigation and regulation.
Sustainable Practices
Sustainable practices in genetic engineering focus on creating agricultural systems that are environmentally friendly and resource-efficient. These practices contribute significantly to the overarching goal of mitigating climate change and preserving natural resources. A key aspect is the integration of genetic engineering with ecological farming methods to improve soil health and enhance crop resilience.
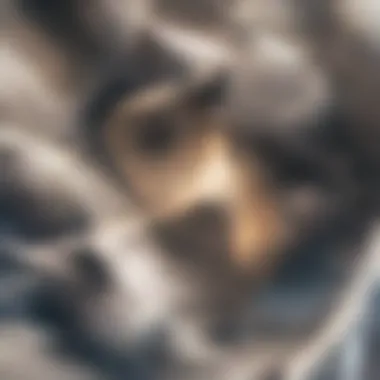
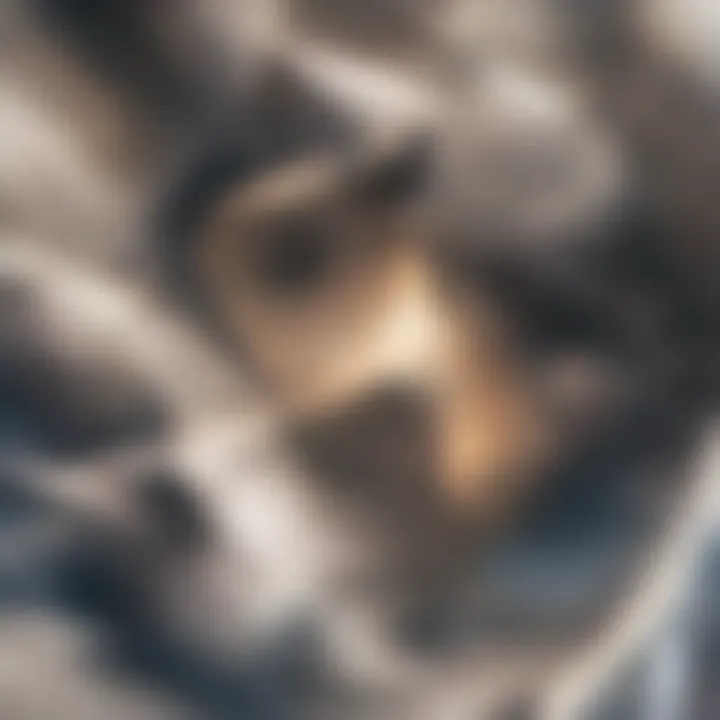
The unique attributes of sustainable practices include using innovative techniques to improve crop yields while minimizing impact on ecosystems. However, transitioning to these practices can require substantial investment and education; not all farmers may be equipped to adopt them readily. Overall, the collaboration between scientific innovation and sustainability will be crucial as we move forward in addressing global challenges.
Ethical Considerations
Ethical considerations play a crucial role in the discourse surrounding genetic engineering. As advancements continue, the moral dimensions of these technologies become more complex. Society must grapple with questions of safety, access, and the potential for misuse. This section aims to highlight these significant elements to provide a nuanced understanding of the ethical landscape in genetic engineering.
Public Perception
Public perception of genetic engineering is often shaped by a mix of hope and fear. Many individuals view genetic advancements as a pathway to curing diseases and enhancing human health. However, there is also a notable apprehension regarding the potential for unintended consequences, such as ecological disruption or ethical dilemmas around designer babies.
Public debates often focus on a few key concerns:
- Safety Risks: There are worries about the long-term effects of genetic modifications on human health and biodiversity.
- Equity Issues: Access to genetic therapies and enhancements may be limited to affluent segments of society, raising concerns about social inequality.
- Misinformation: A lack of understanding can foster myths that complicate discussions — for instance, fears regarding GMOs.
Educating the public on these topics is vital. It allows for informed discourse and can lead to broader acceptance of beneficial technologies while addressing legitimate concerns. This can be achieved through transparent communication and inclusive dialogue between scientists, ethicists, and the general public.
Regulatory Frameworks
The establishment of regulatory frameworks is essential to guide the responsible development of genetic engineering. These frameworks are designed to ensure safety, efficacy, and ethical compliance in genetic research and applications. They vary significantly worldwide, reflecting different cultural values and priorities regarding science and technology.
Key components of these frameworks include:
- Safety Assessments: Rigorous evaluations must be conducted before any new genetic techniques are allowed in clinical settings or agricultural applications.
- Public Engagement: Regulatory bodies must consider public opinions and ethical arguments when framing their policies. This engagement can help to build trust and legitimacy.
- International Standards: Global collaboration is often necessary to address cross-border issues, such as the release of genetically modified organisms and their impact on biodiversity.
"Effective regulation does not just protect; it also fosters innovation by establishing clear guidelines for researchers and industries."
Navigating the ethical terrain of genetic engineering requires ongoing assessment and collaboration between multiple stakeholders. As science progresses, these frameworks must evolve to effectively balance innovation and responsibility.
Challenges in Genetic Engineering
Navigating the realm of genetic engineering presents notable challenges impacting both the scientific community and society at large. This section evaluates prominent difficulties through which researchers and practitioners must maneuver. Understanding these challenges is essential to the advancement and responsible implementation of genetic engineering technologies. The implications of these challenges reach beyond laboratory walls and into practical applications, influencing public opinion, regulatory frameworks, and interdisciplinary collaborations.
Technical Limitations
Technical limitations manifest in various ways, impacting genetic engineering's capabilities and scope. Key challenges include inefficiency in certain editing techniques and unintended off-target effects, which can compromise results. For instance, CRISPR-Cas9, while revolutionary, can sometimes modify unintended genes, leading to potential hazards in clinical settings.
In addition to off-target effects, the delivery of genetic materials into target cells remains a hurdle. Various methods exist, such as viral vectors or nanoparticles, yet each carries its own set of risks and limitations. Overcoming these technical challenges requires continued innovation and robust validation processes to ensure safety and effectiveness.
Societal Concerns
Bioethics
Bioethics encompasses the moral questions arising from genetic interventions. This aspect is vital as it shapes the framework of guidelines and principles governing practices in genetic engineering. The essence of bioethics lies in addressing concerns related to consent, the long-term consequences of genetic modifications, and the implications of playing a role in evolution.
One key characteristic of bioethics is its focus on the dignity and rights of individuals. In the context of genetic engineering, bioethics demands transparency and respect for personal autonomy. These elements are crucial for fostering trust among the public. Engaging in open dialogue about these concerns enhances understanding while promoting responsible innovations.
However, bioethical considerations often result in conflicting viewpoints, highlighting the advantages and disadvantages inherent in ethical discussions. Balancing scientific advancement with ethical responsibility presents ongoing challenges. As genetic engineering evolves, continuous evaluation of ethical practices remains vital.
Socioeconomic Impact
The socioeconomic impact of genetic engineering merges scientific advancement with human societal structures. Accessibility and inequality are among the pressing issues in this context. As genetic technologies unfold, they often carry the risk of widening existing social disparities. For example, access to cutting-edge gene therapies may be restricted to affluent populations, raising questions about equity in healthcare.
Moreover, the economic implications for certain industries can create tension. Traditional methods of agriculture might be overshadowed by genetically modified organisms (GMOs), leading to job displacement and economic disruption in established fields.
A key characteristic of evaluating socioeconomic impact is recognizing its multifaceted nature. Understanding these dynamics ensures that innovation occurs in a comprehensive, inclusive manner that considers all societal segments. Thus, embracing this unique feature both enriches discourse and educates stakeholders on the broader ramifications of genetic engineering.
This exploration of challenges in genetic engineering serves as a foundation for identifying solutions and advancing the field responsibly. Comprehensive insights can guide ongoing discussions and research efforts, amplifying the conversation around genetic engineering's future.
Future Directions for Research
The field of genetic engineering is rapidly evolving, and understanding future directions for research is critical. Exploration of new technologies enhances our capacity to manipulate genetic material with precision and efficiency. It expands the abilities of scientists and researchers to address complex challenges in medicine, agriculture, and beyond.
Emerging Technologies
Synthetic Biology
Synthetic biology aims to design and construct biological parts, devices, and systems. This field is essential for creating organisms with novel capabilities. One key characteristic of synthetic biology is its focus on standardized biological components. This promotes rapid experimentation and innovation. Synthetic biology is popular due to its versatility in applications, from developing new drugs to biofuels production.
A unique feature of synthetic biology is its ability to simplify complex biological processes, making it easier to engineer and manipulate genetic traits. Some advantages include the potential for more sustainable practices and increased efficiency in producing desired outcomes. However, there are also disadvantages, such as the ethical implications and risks associated with creating synthetic life forms.
Gene Drive Technologies
Gene drive technologies enable the spread of specific genetic traits throughout a population. This aspect is crucial for controlling pests and diseases. A main characteristic of gene drive technologies is their ability to ensure that a modified gene is passed on to the next generation, increasing the probability of it becoming widespread. This makes gene drives a beneficial choice for addressing urgent issues like vector-borne diseases.
The unique feature of gene drive technologies is their potential impact on ecosystem dynamics. They offer significant advantages, such as reducing the populations of disease-carrying insects, which can lead to improved public health. However, there are disadvantages as well, including concerns about ecological balance and unintended consequences that may arise from widespread genetic modifications.
Interdisciplinary Approaches
Interdisciplinary approaches are vital for advancing research in genetic engineering. By integrating knowledge from diverse fields, it fosters innovation and enhances problem-solving abilities. Collaboration between life sciences, engineering, and ethics is necessary to navigate the complexities of genetic modifications.
In summary, the exploration of emerging technologies and interdisciplinary approaches will shape the future of genetic engineering. These directions offer promising opportunities for scientific advancement and practical application with the potential to address some of the world’s most pressing challenges.
"Continued research in genetic engineering will not only transform medicine and agriculture but will also require careful consideration of ethical implications."
The role of innovative technologies and collaborative efforts cannot be underestimated in this journey.
Case Studies of Successful Genetic Engineering Projects
Case studies serve as essential frameworks for understanding the real-world implications of genetic engineering. By examining specific projects, we gain insight into the mechanisms, outcomes, and ethical considerations of genetic interventions. These studies not only highlight successful applications but also underscore the benefits and challenges encountered along the way. Such analyses enable researchers, policymakers, and the public to grasp the tangible impacts of genetic technologies on health and agriculture.
Treatment of Genetic Disorders
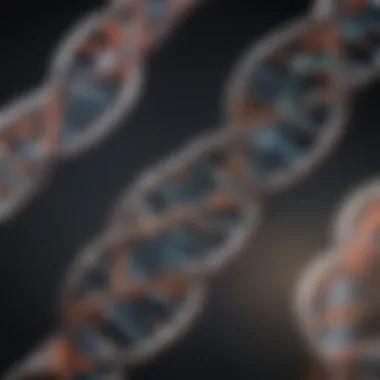
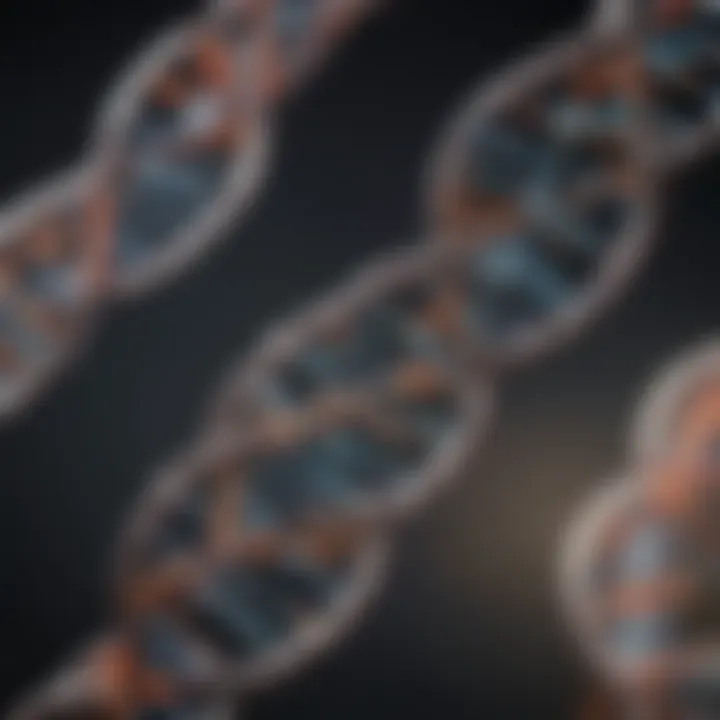
One prominent case study in genetic engineering involves gene therapy for genetic disorders. This innovative approach has demonstrated remarkable potential in treating ailments such as cystic fibrosis and muscular dystrophy. A notable example is the use of Luxturna, a gene therapy designed to treat inherited retinal diseases.
Luxturna works by delivering a functional copy of the RPE65 gene directly into retinal cells. This method has restored vision for patients with specific genetic mutations, showcasing the power of targeted genetic interventions. With increasing understanding of the genetic underpinnings of other disorders, similar therapies are likely to emerge, allowing treatments to become more personalized and effective.
Development of Resilient Crop Varieties
In the domain of agriculture, the development of resilient crop varieties exemplifies the impactful use of genetic engineering. Crops such as Bt cotton and Golden Rice represent groundbreaking advancements in agricultural science.
Bt cotton is engineered to express a bacterial protein that protects against pests, reducing the need for chemical pesticides and, consequently, minimizing environmental impact. This modification has led to increased yields, benefiting farmers and contributing to food security.
On the other hand, Golden Rice has been engineered to produce vitamin A. This fortification aims to combat vitamin A deficiency, which affects millions around the world. The success of both crop varieties illustrates how genetic engineering can address urgent agricultural challenges and improve nutritional outcomes.
As these case studies reveal, genetic engineering is transforming the landscape of medicine and agriculture. They not only show the possibilities of these technologies but also highlight the importance of ongoing research and ethical discussions in navigating the future of genetic interventions.
The Role of Collaboration in Advancing Genetic Engineering
Collaboration plays a crucial role in the ongoing evolution of genetic engineering. This field, characterized by rapid advancements, requires the pooling of knowledge, skills, and resources from various sectors. By working together, academic institutions, private industry, and government agencies can enhance research and development efforts, thus accelerating innovation and application in medicine, agriculture, and other domains. This collaborative approach not only fosters the exchange of ideas but also strengthens the scientific infrastructure necessary for complex genetic engineering projects.
The significance of collaboration in genetic engineering can be summarized in a few key elements.
- Resource Sharing: Collaboration allows institutions to share expensive technologies and facilities. Many genetic engineering projects require sophisticated equipment, which may be cost-prohibitive for a single entity. By sharing resources, organizations can conduct experiments that would otherwise be impossible.
- Diverse Expertise: Genetic engineering encompasses a range of disciplines, including molecular biology, bioinformatics, and ethics. Collaboration brings together experts from these fields, promoting a multidisciplinary approach that can yield more comprehensive solutions.
- Increased Funding Opportunities: Joint ventures often attract larger grants and funding from governmental bodies and philanthropic organizations. Working collaboratively can create a stronger case for financial support due to the pooled expertise and shared risk involved.
"Collaboration is the bedrock of scientific progress, driving breakthroughs that single entities often cannot achieve alone."
As we delve into the specific elements of collaboration, we can examine academic partnerships and industry involvement in greater detail.
Academic Partnerships
Academic institutions are fundamental to advancing research in genetic engineering. They cultivate the next generation of scientists and provide a sandbox for innovation. Through partnerships with other educational institutions, researchers can tackle more significant challenges and share findings more efficiently. Academic partnerships also facilitate internships and training programs, preparing students for careers in genetic engineering and related fields.
Research consortia formed by universities can allow institutions to share methodology and findings. These partnerships often lead to novel discoveries and have been essential in areas such as gene therapy and the development of genetically modified organisms.
Effective collaborations often result in groundbreaking publications and patents, both of which enhance the credibility and visibility of the partnering institutions.
Industry Involvement
Industry involvement in genetic engineering has become increasingly evident, as companies recognize the potential for profit and innovation. The biotechnology sector thrives on advancements in genetic technologies. Collaboration between academia and industry can help translate basic research into practical applications, bridging the gap between theoretical research and market-ready products.
Collaboration with private companies can provide universities with funding and access to technology. In return, industry partners benefit from academic innovations that can lead to the next major breakthrough in their products.
Key Benefits of Industry Collaboration:
- Accelerated Development: Companies can accelerate the development process of new technologies by accessing cutting-edge research already conducted in academic labs.
- Market Insights: Industry partners often bring knowledge about market needs, aligning academic research with real-world applications.
- Commercialization Opportunities: Companies that collaborate with academic institutions gain insights that can lead to the successful commercialization of new technologies in genetic engineering.
Real-world examples illustrate the successful interplay between academia and industry. Companies like CRISPR Therapeutics collaborate with research institutions to advance gene editing techniques, illustrating how strategic partnerships can propel the field forward.
Impact on Global Health
Genetic engineering plays a crucial role in shaping global health by addressing some of the most pressing health challenges we face. The advancements in this field have not only intensified our understanding of genetics but also shifted the paradigms of both treatment and prevention of diseases. Notably, breakthroughs in this sector provide the ability to design therapeutic interventions tailored to individual genetic profiles, which can result in enhanced efficacy and reduced side effects. This personalization is a significant leap toward better patient care, especially in areas like oncology and rare genetic disorders.
In the realm of public health, genetic engineering can drive substantial improvements in disease prevention and control. By utilizing the tools of genetic modification, we can enhance the production of vaccines and develop novel therapies to combat infectious diseases. The implications for global health are profound, as these approaches address both existing and emerging health threats.
Vaccination Development
Vaccines are a cornerstone of public health, effectively reducing the incidence of infectious diseases. Genetic engineering has revolutionized the way vaccines are developed, providing tools to create more effective and safer vaccines at a faster pace than traditional methods.
Designing vaccines using genetic engineering involves using recombinant DNA technology. This allows scientists to insert genes from pathogens into safe host organisms to produce antigens that stimulate an immune response without causing disease. For example, the development of the HPV vaccine involved the use of genetic engineering to create virus-like particles that mimicked the structure of the actual virus. This technique not only shortened the development time but also enhanced the safety profile, leading to widespread vaccination campaigns.
"Genetic engineering allows for the rapid development of vaccines, paving the way for efficient responses to outbreaks and pandemics."
Such advancements have become indispensable, especially in response to emerging infectious diseases. The recent COVID-19 pandemic showcased this capability, as mRNA vaccines were developed and distributed within a year of identification, demonstrating the power of genetic engineering in urgent public health scenarios.
Addressing Infectious Diseases
Infectious diseases continue to pose a serious global health challenge, affecting millions each year. Genetic engineering addresses these challenges in various ways, enhancing our capability to control outbreaks and develop new treatments.
One effective strategy involves gene editing technologies like CRISPR. By targeting specific genes in pathogens, researchers can potentially render them less virulent, or even custom design bacteria and viruses that can combat resistant strains. This has important implications not only for developing new antibiotics but also for controlling diseases that have proved difficult to manage through traditional methods.
Additionally, genetic engineering facilitates the creation of genetically modified organisms that can produce therapeutic proteins or antibodies essential for treating infectious diseases. For example, genetically modified plants and animals can serve as biofactories to produce life-saving drugs and vaccines.
This dual approach of prevention through vaccination and treatment via advanced therapies embodies the potential of genetic engineering in global health. Addressing infectious diseases through these methods not only promises to save lives but also to improve the quality of life for affected populations around the world.
Understanding Genetic Privacy
The topic of genetic privacy is increasingly significant within the framework of genetic engineering. As advancements in this field continue to flourish, the way individuals view their genetic data evolves. Genetic privacy encompasses the protection, ownership, and ethical considerations of genetic information, which holds implications for personal identity and societal norms. It affects everything from medical data usage to personal autonomy over genetic material, highlighting the need for a robust understanding of these dynamics.
Genetic Data Ownership
With the rise of genetic testing services like 23andMe and AncestryDNA, questions of genetic data ownership have gained prominence. Who truly owns the genetic information collected? The dynamics become complex when third-party companies gain access to this sensitive information.
- Patients often sign agreements that may permit companies to use their genetic data for research and commercial purposes without direct compensation or recognition.
- Researchers face dilemmas about how to manage and utilize genetic data in a way that respects the privacy of individuals.
- Legislators are pressured to implement frameworks that ensure individuals retain control over their own information.
The challenge remains in creating balances between utilitarian benefits from genetic research and respecting personal ownership of genetic data.
Implications for Individuals
The implications of genetic privacy directly affect individuals and broader society. When people provide their genetic information, it can result in both benefits and potential risks:
- Benefits include:
- Risks involve:
- Enhanced healthcare: Genetic data contributes to personalized treatments and targeted therapies.
- Understanding predispositions: Individuals gain insights about genetic risks for diseases, enabling proactive health management.
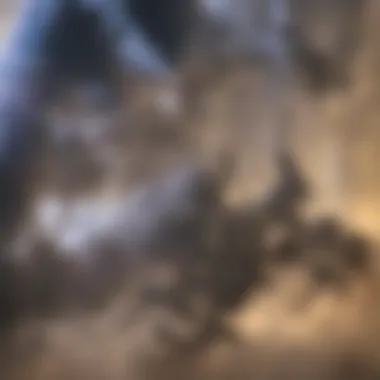
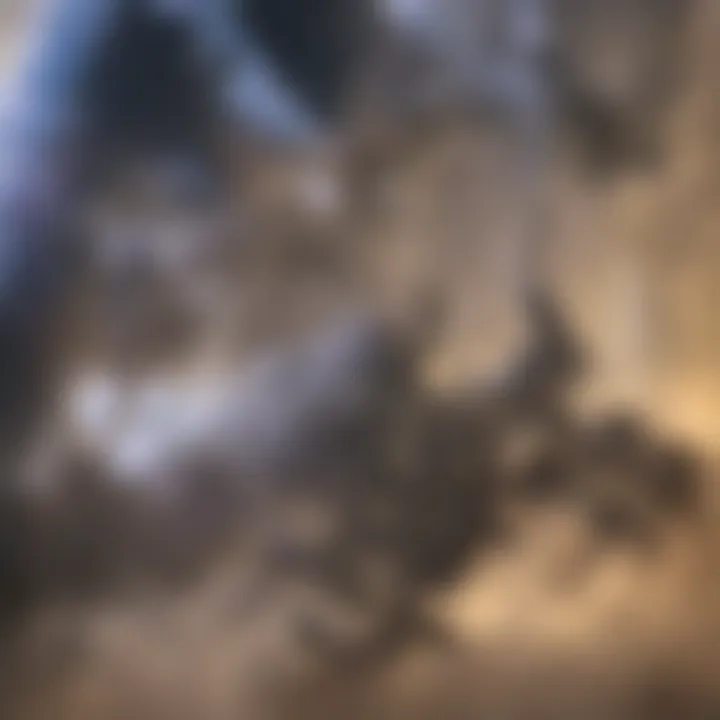
- Discrimination: There is potential for misuse of genetic information by employers or insurance companies, leading to discriminatory practices.
- Loss of autonomy: Individuals might feel a lack of control over how their genetic information is used, potentially infringing on their rights.
Public Policy and Genetic Engineering
Public policy plays a pivotal role in the realm of genetic engineering. As the field evolves rapidly, it is imperative to have a framework that governs research, application, and commercialization of genetic technologies. This section will outline the relevance of public policy in genetic engineering, focusing on vital aspects, benefits, and necessary considerations.
Policies ensure that genetic engineering practices are safe, ethical, and in line with societal values. Without adequate regulation, genetic manipulation can pose dangers not just to individual patients or consumers, but to entire ecosystems. Policymakers must grapple with various questions, such as the long-term impact of genetically modified organisms (GMOs) on agriculture or the implications of genetic therapies on human genetics.
Legislation and Governance
Legislation surrounding genetic engineering is critical. It establishes the legal framework that dictates how research and technology can be developed and used. In many jurisdictions, laws are structured around the concepts of safety, accountability, and transparency. These laws can govern everything from laboratory practices to field trials of genetically modified crops.
Governance structures need to be dynamic. They must adapt to advancements such as CRISPR and synthetic biology, which challenge existing regulations. Countries differ significantly in how they handle genetic technologies. For example, the European Union has stringent regulations compared to the more permissive policies in the United States. This disparity can affect international collaboration and research initiatives.
Influencing Policy Decisions
Influencing policy decisions in genetic engineering requires the integration of diverse perspectives—from scientists and ethicists to the general public. Awareness campaigns and educational initiatives can help demystify genetic technologies, promoting informed public dialogue. Public understanding is vital, as perceptions can heavily influence policymakers.
The role of advocacy groups cannot be understated. They can provide valuable insights into public opinion and ethical considerations that politicians must address. Additionally, collaborating with stakeholders from academia, industry, and civil society helps create a well-rounded approach to policy-making.
"Effective public policy in genetic engineering can bridge the gap between innovation and safety, ensuring that breakthroughs improve lives while minimizing risks."
By creating inclusive dialogues and leveraging scientific advancements, stakeholders can drive responsible legislation that fosters innovation while protecting public interests.
The Intersection of Genetic Engineering and Artificial Intelligence
The integration of artificial intelligence (AI) into the field of genetic engineering represents a transformative advancement. It allows researchers to analyze vast datasets and interpret complex genetic information with remarkable speed and accuracy. The role of AI extends beyond mere data processing; it opens new pathways for discovery, facilitates innovative solutions in genetics, and addresses significant challenges. By combining these two powerful fields, we can enhance the precision of genetic modifications and accelerate the pace of scientific breakthroughs.
Data Analysis and Genomics
The use of AI for data analysis in genomics cannot be overstated. Generating genomic data is a resource-intensive process, producing enormous amounts of information. AI algorithms, particularly machine learning models, help to sift through this data efficiently. They identify patterns and correlations that might be overlooked by traditional analysis methods.
Some specific benefits include:
- Improved accuracy in determining gene functions.
- Accelerated identification of genetic variations associated with diseases.
- Enhanced capabilities in predicting genetic interactions and outcomes.
The ability of AI to enhance the annotation of genomic data allows scientists to better understand the roles of specific genes in health and disease. For instance, AI techniques have already shown promise in elucidating the complexities of interactions within gene networks.
AI in Drug Development
In the realm of drug development, AI demonstrates its potential by streamlining the identification of new pharmaceutical compounds. Traditional methods of drug discovery can be slow and costly, often requiring years of research before a viable product emerges. AI can significantly reduce the timeline for discovering new drugs by rapidly analyzing potential molecular targets and predicting their interactions.
Key aspects of AI applications in drug development include:
- Predictive modeling: AI can forecast how different compounds will behave in biological systems.
- Clinical trial optimization: Algorithms can help identify suitable candidates for trials, improving efficiency and outcomes.
- Repositioning existing drugs: AI can uncover new therapeutic uses for already approved medications, a process often termed drug repurposing.
The potential of AI to transform drug development not only promises more effective treatments but also addresses key issues around accessibility. By making drug discovery faster and cheaper, we could see significant improvements in global health outcomes.
"The synergy of AI and genetic engineering could lead to a deeper understanding of human biology and innovative therapeutic strategies that were previously unthinkable."
The intersection of AI and genetic engineering stands as a beacon of future possibilities, holding the promise to reshape how we understand and manipulate the genetic fabric of life.
Challenges in Public Understanding of Genetic Engineering
Understanding genetic engineering requires a nuanced approach due to its complexity and multifaceted implications. The public’s grasp on this subject often struggles against a backdrop of misinformation and misconceptions. This section aims to clarify these challenges, emphasizing the necessity of sound knowledge in order to foster informed discussions surrounding genetic engineering.
Misinformation and Myths
Misinformation prevails in the realm of genetic engineering, leading to widespread confusion. This sometimes stems from sensationalized media coverage or lack of expertise. For instance, many people regard genetic modification as inherently dangerous or unnatural. Such beliefs are often informed by myths, such as the assumption that all genetically modified organisms (GMOs) are harmful. The scientific community has repeatedly shown that GMOs undergo rigorous testing before becoming available in the market. Yet, these realities are frequently overshadowed by exaggerated alarmist narratives.
Some common myths include:
- All GMOs are bad: Scientific assessments reveal that GMOs can be beneficial, providing higher yields and resistance to pests, which can enhance food security.
- Genetic engineering is the same as cloning: Genetic engineering focuses on altering specific genes while cloning aims to replicate entire organisms.
These erroneous beliefs can erode public trust and impede progress in genetic research. To address this misinformation, it is essential to convey accurate, digestible information in various formats and platforms.
Educational Initiatives
Educational initiatives play a crucial role in bridging the knowledge gap in genetic engineering. These programs aim to demystify the science and clarify its implications in a straightforward manner. They can include workshops, community discussions, and school curricula, targeting various audiences from students to adults.
Key components of successful educational initiatives may include:
- Hands-on Learning: Incorporating practical experiences, such as laboratory visits or biotechnology demonstrations, to engage learners directly.
- Collaborative Efforts: Partnerships between scientists and educators to design clear and relatable teaching materials.
- Utilization of Technology: Offering online courses and webinars to reach a broader audience, including those in remote areas.
"Education is essential in shaping public perceptions and acceptance of genetic engineering advancements."
By implementing such initiatives, society can cultivate a better understanding and ultimately, foster a more informed dialogue about the future of genetic engineering. Public engagement with accurate, evidence-based education is paramount. This approach can demystify the complexities of genetic engineering, paving the way for wise policy decisions and enhanced public support for scientific endeavors.
End
The topic of conclusion holds significant value in any article, especially one focused on breakthroughs in genetic engineering. Here, it serves as a crucial element that distills complex discussions into digestible insights. A well-crafted conclusion can cohesively synthesize findings, ensuring the audience retains key information.
This article meticulously examined various aspects of genetic engineering, emphasizing its advancements and implications. By highlighting crucial points throughout, we positioned genetic engineering not only as a scientific endeavor but also as a field that influences public health, agriculture, and ethical discussions. It is essential to note the intertwined nature of technology and society that we explored, revealing how advancements can generate both enthusiasm and skepticism among the public.
In summary, the conclusion offers an opportunity to reflect on the developments discussed, reiterating the importance of responsible practices and ethical frameworks. It underscores that while we stand at the brink of remarkable possibilities, careful navigation through the associated challenges is necessary. The overarching message here is that genetic engineering, when approached thoughtfully, can unlock potential that benefits society at large.
Summary of Key Points
- Technological Innovations: Genetic engineering has made significant strides due to techniques like CRISPR-Cas9, making precise modifications feasible.
- Applications in Medicine and Agriculture: The impact of genetic modifications is prominent in areas such as gene therapy and the development of resilient crops.
- Ethical Considerations: Issues surrounding public perception and regulatory frameworks are pivotal when discussing the implications of genetic engineering.
- Challenges and Future Directions: Addressing technical limitations and societal concerns will guide the future trajectories of research in genetic engineering.
The Path Forward
The future of genetic engineering is both promising and complex. As we continue to explore emerging technologies like synthetic biology and gene drive technologies, interdisciplinary approaches become crucial. These methods not only harness the knowledge from genetics but also integrate insights from fields like computational biology and ethics.
Engaging with diverse stakeholders—academics, industries, and policy-makers—will help shape effective frameworks. This collaborative approach is essential for advancing genetic engineering responsibly. Policy decisions should reflect a balance between innovation and ethical considerations, addressing public concerns while fostering scientific progress.
Ultimately, the path forward will depend on our ability to communicate effectively about the benefits and risks of genetic engineering. Education initiatives can play a vital role in demystifying the field, making complex concepts accessible to a wider audience. This will ensure informed discussions about the potential and limitations of genetic engineering in transforming both science and society.